References
Published online by Cambridge University Press: 05 February 2016
Summary
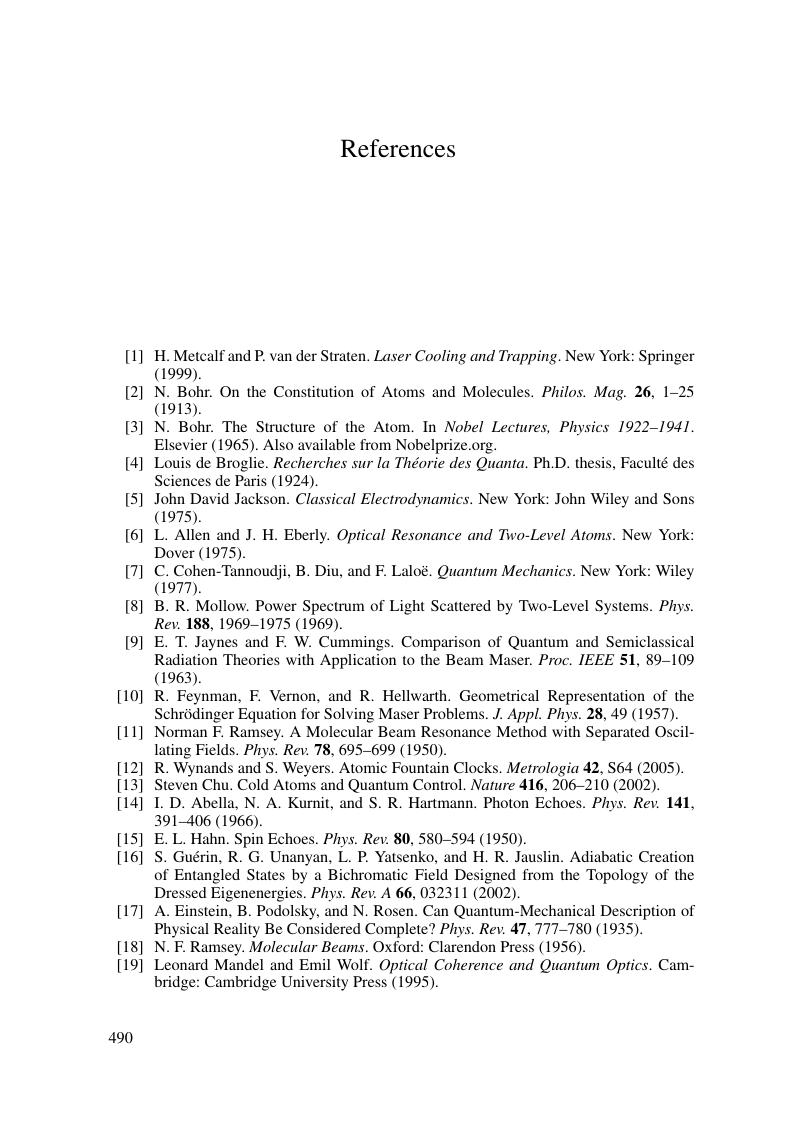
- Type
- Chapter
- Information
- Atoms and Molecules Interacting with LightAtomic Physics for the Laser Era, pp. 490 - 507Publisher: Cambridge University PressPrint publication year: 2016