Book contents
- Frontmatter
- Contents
- Preface
- 1 Introduction
- 2 Soil properties
- 3 Forest soil development and classification
- 4 Soil fungi
- 5 Soil water
- 6 Forest carbon cycle
- 7 Nutrient cycling
- 8 Northern forests in a high-CO2 world
- 9 Soil acidity and heavy metal pollution
- 10 Nitrogen
- 11 Soil functioning and climate change
- References
- Index
- Plate section
- References
References
Published online by Cambridge University Press: 26 April 2011
- Frontmatter
- Contents
- Preface
- 1 Introduction
- 2 Soil properties
- 3 Forest soil development and classification
- 4 Soil fungi
- 5 Soil water
- 6 Forest carbon cycle
- 7 Nutrient cycling
- 8 Northern forests in a high-CO2 world
- 9 Soil acidity and heavy metal pollution
- 10 Nitrogen
- 11 Soil functioning and climate change
- References
- Index
- Plate section
- References
Summary
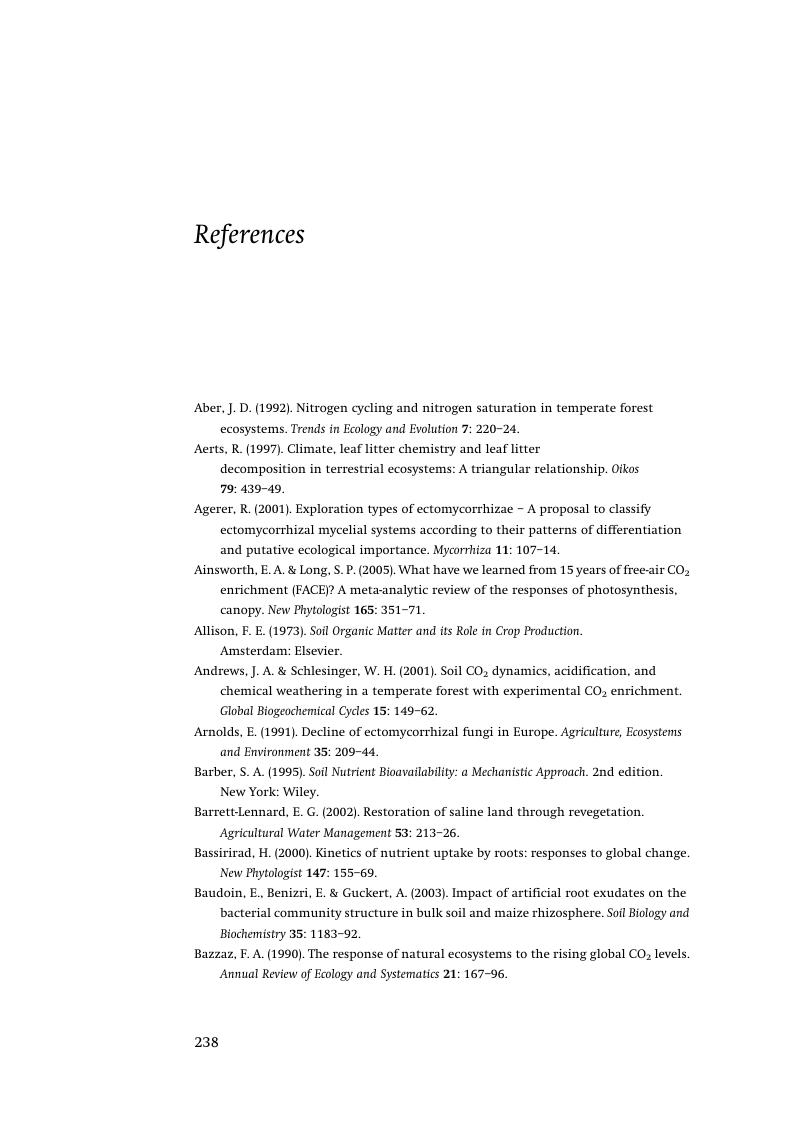
- Type
- Chapter
- Information
- Soil Ecology in Northern ForestsA Belowground View of a Changing World, pp. 238 - 252Publisher: Cambridge University PressPrint publication year: 2011