Introduction
Cadmium (Z = 48) belongs to Group 12 of the periodic table and is similar to Zn (Z = 30) and Hg (Z = 80) in its chemical and physical properties. Abundances of Cd, Zn and Hg in the upper continental crust are 0.102, 52 and 0.056 μg/g, respectively (Wedepohl, Reference Wedepohl1995). Owing to its chalcophile behaviour, Cd is hosted mainly in sulfides and usually replaces Zn in sphalerite and other Zn-bearing chalcogenides. Currently, only 28 minerals contain Cd as a species-forming element, and the most represented crystal-chemical classes are those of sulfides and sulfosalts (11 species), sulfates (7), and phosphates and arsenates (6). Cadmium chalcogenide minerals are given in Table 1. Among them, the most common minerals are the two dimorphs of the CdS compound, greenockite (wurtzite-type) and hawleyite (sphalerite-type); they usually occur in hydrothermal environments as well as in coatings on sphalerite. Tennantite-(Cd) is the last addition to this group of Cd-bearing compounds.
Table 1. Cadmium sulfides and sulfosalts.

*Chemical formulae after the official IMA-CNMNC List of Mineral Names (http://cnmnc.main.jp/ updated May 2022).
The examination of an old specimen from the Berenguela mining district, Pacajes Province, La Paz, Bolivia, originally belonging to an old German collection, led to the recognition of a new potential Cd-member of the tennantite series. Further chemical and crystallographic investigations confirmed such a preliminary identification, allowing the proposal of the new mineral species tennantite-(Cd). The new mineral and its name, in keeping with the current nomenclature of tetrahedrite group (Biagioni et al., Reference Biagioni, George, Cook, Makovicky, Moëlo, Pasero, Sejkora, Stanley, Welch and Bosi2020a), have been approved by the Commission on New Minerals, Nomenclature and Classification of the International Mineralogical Association, under the voting number 2021-083 (Biagioni et al., Reference Biagioni, Kasatkin, Sejkora, Nestola and Škoda2022b). Its mineral symbol, in accord with Warr (Reference Warr2021), is Tnt-Cd. The holotype specimen is deposited in the collections of the Fersman Mineralogical Museum of the Russian Academy of Sciences, Moscow, Russia, under catalogue number 97773. Cotype material is kept in the collections of the Department of Mineralogy and Petrology, National Museum in Prague, Cirkusová 1740, 193 00 Praha 9, Czech Republic, with registration number P1P 47/2021.
In this paper the description of tennantite-(Cd) is reported.
Occurrence and physical properties
Tennantite-(Cd) was found on a specimen from the Berenguela mining district, which is located ~35 km north-east of where the borders of Chile, Peru and Bolivia intersect, at the headwaters of the Rio Berenguela (Fig. 1). The mining district includes several sediment- and volcanic-hosted veins that were exploited during Spanish colonial times. No mining activity has taken place since World War II. The rocks of the Berenguela district are divided broadly into Eocene to Oligocene continental sedimentary rocks and Miocene volcanic, volcaniclastic and intrusive rocks. The older sedimentary rocks include the red quartz arenites and mudstones of the Berenguela Formation and black to red arkoses of the Mauri Formation. The Mauri–Berenguela contact is disconformable to uncomformable. The younger volcanic-rich units include interbedded sandstones, conglomerates, reworked tuffaceous sediments and pyroclastic airfall deposits (Wallace, Reference Wallace1975; Jimenez et al., Reference Jimenez Ch., Barrera, Flores, Lizeca, Murillo, Sanjines, Hardyman, Tosdal and Wallace1993).
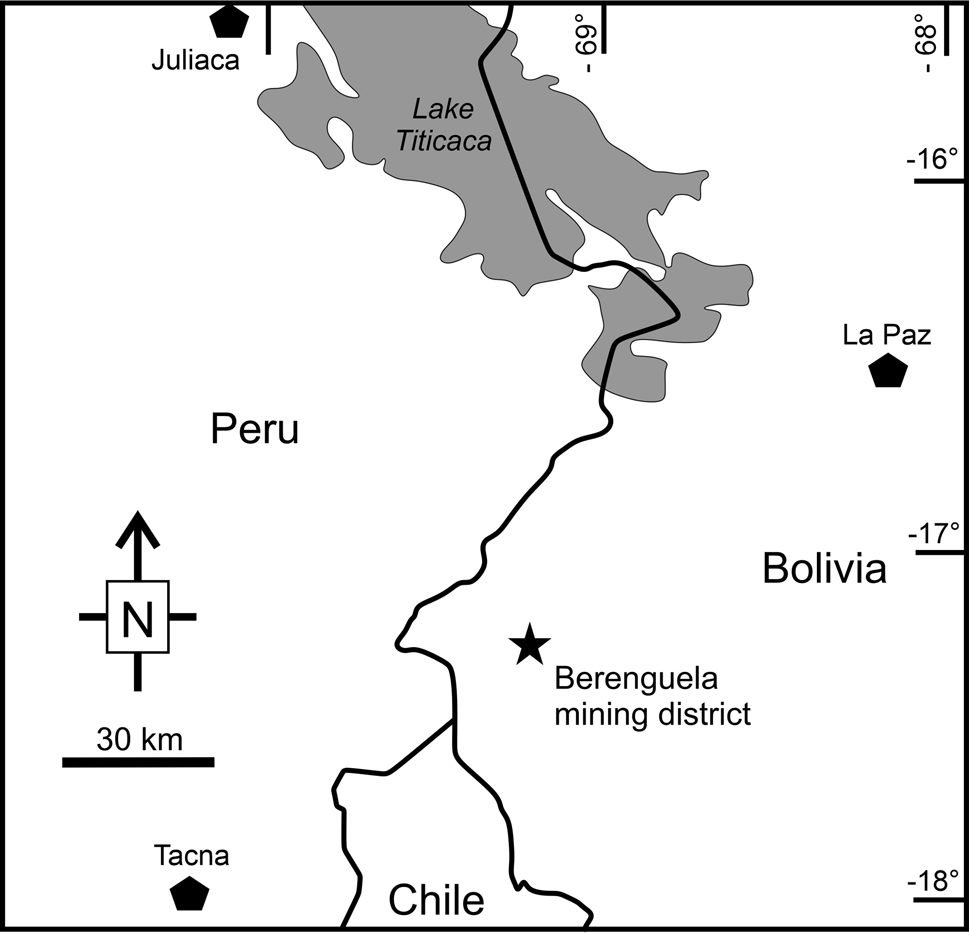
Fig. 1. Location of the Berenguela mining district.
The mineral deposits of the Berenguela district include Cd-rich veins in the Berenguela and lower Mauri Formations and Cd-poor veins in the younger volcaniclastic and intrusive rocks. The veins are localised at fracture intersections. The samples containing tennantite-(Cd) were probably mined in the upper part of the veins of the Berenguela Formation in the first half of the 20th century.
Tennantite-(Cd) occurs as anhedral grains, up to 1 × 1 mm in size, black in colour, with a reddish-brown streak, and metallic lustre (Fig. 2). Mohs hardness was not measured, but it should be close to 3½–4, in agreement with other members of the tetrahedrite group. It is brittle, with a conchoidal fracture and indistinct cleavage. Owing to the small amount of available material, density was not measured; on the basis of the empirical formula and the single-crystal X-ray diffraction data, the calculated density is 4.724 g/cm3. In reflected light, tennantite-(Cd) is isotropic. It is grey, with brownish tints. Internal reflections are very rare, with a brownish-red colour. Reflectance values were measured in air using a spectrophotometer MSP400 Tidas on a Leica microscope, with a 50× objective (Table 2). The reflectance curve for tennantite-(Cd) compared with published data for other tennantite-series minerals is shown in Fig. 3. In agreement with Spiridonov et al. (Reference Spiridonov, Petrov and Voropayev1988) for Cd-bearing samples belonging to the tetrahedrite and freibergite series, the reflectance curve of tennantite-(Cd) is similar to that of tennantite-(Zn).

Fig. 2. Tennantite-(Cd), as anhedral grains in baryte with blue aldridgeite. Holotype material (catalogue number 97773).

Fig. 3. Reflectance curve for tennantite-(Cd). For the sake of comparison, the reflectance curves of other members of the tennantite series are shown: tennantite-(Fe), Wheal Jewel, Gwennap, Cornwall, U.K. (Criddle and Stanley, Reference Criddle and Stanley1993); tennantite-(Zn), Tsumeb, Namibia (Criddle and Stanley, Reference Criddle and Stanley1993); tennantite-(Hg), Lengenbach, Binn Valley, Switzerland (Biagioni et al., Reference Biagioni, Sejkora, Raber, Roth, Moëlo, Dolníček and Pasero2021).
Table 2. Reflectance data for tennantite-(Cd).*

*The reference wavelengths required by the Commission on Ore Mineralogy (COM) are given
in bold.
In the holotype specimen, tennantite-(Cd) occurs in baryte, associated with montmorillonite and some Cd-secondary sulfates, i.e. aldridgeite, (Cd,Ca)(Cu,Zn)4(SO4)2(OH)6⋅3H2O, niedermayrite, Cu4Cd(SO4)2(OH)6⋅4H2O, and voudourisite, CdSO4⋅H2O (Fig. 2).
Chemical data
Quantitative chemical analyses were carried out using a Cameca SX 100 electron microprobe (Department of Geological Sciences, Faculty of Science, Masaryk University, Brno, Czech Republic) and the following experimental conditions: wavelength dispersive spectroscopy mode, accelerating voltage = 25 kV, beam current = 10 nA and beam diameter 2 μm. Standards (element, emission line) were: chalcopyrite (CuKα, SKα), Ag (AgLα), FeS2 (FeKα), ZnS (ZnKα), Cd (CdLβ), pararammelsbergite (AsLβ) and HgTe (HgMα). The contents of other sought elements with Z > 8 (including Sb) were below detection limits. Matrix correction by PAP procedure (Pouchou and Pichoir, Reference Pouchou, Pichoir and Armstrong1985) was applied to the data. Results are given in Table 3.
Table 3. Chemical data (in wt.%) and atoms per formula unit (apfu), based on ΣMe = 16 apfu, for tennantite-(Cd).

n = number of spot analyses; e.s.d. = estimated standard deviation.
X-ray crystallography
Powder X-ray diffraction data (Table 4) were collected at room temperature using a Bruker D8 Advance diffractometer equipped with a solid-state LynxEye detector and secondary monochromator generating CuKα radiation (Department of Mineralogy and Petrology, National Museum, Prague, Czech Republic). The instrument was operating at 40 kV and 40 mA. In order to minimise the background, the powder samples were placed on the surface of a flat Si wafer. The powder diffraction pattern was collected in the Bragg-Brentano geometry in the 2θ range 3–65°, step 0.01°, and counting time of 20 s per step (total duration of the experiment was ~30 hours). The positions and intensities of diffraction lines were found and refined using the Pearson VII profile-shape function of the ZDS program package (Ondruš, Reference Ondruš1993). Unit-cell parameters were refined using the least-squares software of Burnham (Reference Burnham1962) and gave a = 10.2976(8) Å and V = 1091.9(2) Å3.
Table 4. Powder X-ray diffraction data for tennantite-(Cd).*

*Notes: I calc1, d calc1 were calculated using the software PowderCell2.3 (Kraus and Nolze, Reference Kraus and Nolze1996) on the basis of the structural model given in Table 6. Only reflections with I calc > 1 are listed; the strongest lines are given in bold.
Single-crystal X-ray diffraction intensity data were collected using a Supernova Rigaku-Oxford Diffraction single-crystal diffractometer (50 kV and 0.12 mA) equipped with a 200 K Pilatus Dectris detector and graphite-monochromatised MoKα radiation (Dipartimento di Geoscienze, Università di Padova, Italy). The detector-to-crystal distance was set at 68 mm. Data were collected by 761 frames over 22 runs in 1° slices, with an exposure time of 60 s per frame, and they were corrected for Lorentz, polarisation, and absorption using the software package Crysalis Pro3 (version 41.64.113a). The refined unit-cell parameters are a = 10.3088(2) Å, V = 1095.53(6) Å3; and space group I $\bar{4}$3m. The crystal structure of tennantite-(Cd) was refined using Shelxl-2018 (Sheldrick, Reference Sheldrick2015) starting from the structural model of Johnson and Burnham (Reference Johnson and Burnham1985). The following neutral scattering curves, taken from the International Tables for Crystallography (Wilson, Reference Wilson1992), were initially used: Cu vs □ at M(2), Cu vs Cd at M(1), As at X(3), S at S(1) and S(2) sites. Several cycles of isotropic refinement converged to R 1 = 0.095 confirming the correctness of the structural model. The modelling of the racemic twin suggested that the structure had to be inverted. An anisotropic refinement for cations only converged to R 1 = 0.038. The M(2) site displayed a relatively large U eq value, suggesting its splitting, in agreement with previous authors (e.g. Andreasen et al., Reference Andreasen, Makovicky, Lebech and Karup-Møller2008; Welch et al., Reference Welch, Stanley, Spratt and Mills2018). After the addition of the split position, found in the difference-Fourier map, the R 1 value was lowered to 0.0242. The site occupancy factors (s.o.f.) at the two split positions M(2a) and M(2b) were constrained to be 1. After several cycles of anisotropic refinement for all the atoms, the R 1 converged to 0.0152 for 359 unique reflections with F o > 4σ(F o) and 22 refined parameters. Details of the data collection and crystal structure refinement are reported in Table 5. Fractional atomic coordinates and equivalent isotropic displacement parameters are reported in Table 6, whereas Table 7 reports selected bond distances and Table 8 the weighted bond-valence balance calculated according to the bond parameters of Brese and O'Keeffe (Reference Brese and O'Keeffe1991). The crystallographic information file has been deposited with the Principal Editor of Mineralogical Magazine and is available as Supplementary material (see below).
Table 5. Summary of crystal data and parameters describing data collection and refinement for tennantite-(Cd).

1 w = 1/[σ2(F o2)+(0.0108P)2+0.6772P].
2 Flack (Reference Flack1983)
Table 6. Sites, Wyckoff positions, site occupancy factors (s.o.f.), fractional atom coordinates and equivalent isotropic displacement parameters (Å2) for tennantite-(Cd).

Table 7. Selected bond distances (Å) for tennantite-(Cd).

Table 8. Weighted bond-valence sums (in valence unit) for tennantite-(Cd).*

*Note: left and right superscripts indicate the number of equivalent bonds involving cations and anions, respectively. The following site populations were used: M(2a) = Cu0.84; M(2b) = Cu0.08; M(1) = Cu0.67Cd0.26Zn0.07; X(3) = As1.00.
Results and discussions
Chemical formula
There are different approaches to the recalculation of the chemical formula of a tetrahedrite-group mineral; among them, the two better normalisation methods are based on ΣMe = 16 atoms per formula unit (apfu) or on the basis of (As + Sb + Te + Bi) = 4 apfu. The former approach assumes that no vacancies occur at the M(2), M(1) and X(3) sites, whereas the latter is based on the negligible variations in the ideal number of cations hosted at the X(3) site, as discussed by Johnson et al. (Reference Johnson, Craig and Rimstidt1986).
The first approach gives the chemical formula Cu9.98(9)Ag0.01(1)Cd1.57(15)Zn0.46(15)Fe0.01(1)As3.97(6)S13.05(19), whereas the other normalisation strategy corresponds to the formula Cu10.04(21)Ag0.01(1)Cd1.59(16)Zn0.46(15)Fe0.01(1)As4.00S13.14(35). The simplified formula of tennantite-(Cd) is Cu6Cu4(Cd,Zn,Fe)2As4S13, corresponding to the end-member formula Cu+6(Cu+4Cd2+2)As4S13. It corresponds to (in wt.%) Cu 40.30, Cd 14.26, As 19.01, S 26.43, total 100.00.
In the sample studied, the Cu content is very close to the ideal (i.e. 10 apfu), with only a negligible Ag content. The C constituent is represented by Cd and minor Zn; iron, probably occurring as Fe3+ (e.g. Makovicky et al., Reference Makovicky, Tippelt, Forcher, Lottermoser, Karup-Møller and Amthauer2003), occurs in very low amounts. Figure 4 shows the Cd vs. Zn content in the 11 spot analyses performed on type material; an inverse relation between these two elements is apparent. Finally, chemical data do not support any significant S deficiency in tennantite-(Cd).

Fig. 4. Relationship between Cd and Zn content (in apfu) in tennantite-(Cd).
Crystal structure description
Tennantite-(Cd) is isotypic with the other members of the tetrahedrite group. Its crystal structure can be described as a framework of corner-sharing M(1)S(1)4 tetrahedra with cavities hosting X(3)S(1)3 pyramids around S(2)M(2)6 octahedra (e.g. Johnson et al., Reference Johnson, Craig and Rimstidt1988).
The M(1) site has a mixed (Cu,Cd,Zn) occupancy. The refined site scattering is 196.2 electrons per formula unit (epfu); this value can be compared with that calculated from the site population proposed on the basis of chemical data, i.e. M (1)(Cu4.00Cd1.55Zn0.45), corresponding to 203.9 epfu. The average bond distance is 2.370 Å, longer than that observed in mixed (Cu,Zn/Fe) M(1) sites in tetrahedrite-group minerals (e.g. Wuensch, Reference Wuensch1964; Wuensch et al., Reference Wuensch, Takéuchi and Nowacki1966), and similar to the bond distance observed in tetrahedrite-(Hg) and tennantite-(Hg), i.e. 2.3913 Å and 2.389 Å (Biagioni et al., Reference Biagioni, Sejkora, Musetti, Velebil and Pasero2020b; Reference Biagioni, Kasatkin, Sejkora, Nestola and Škoda2021), respectively. This agrees with the similar crystal radii of Cd2+ and Hg2+, i.e. 0.84 Å (Johnson et al., Reference Johnson, Craig and Rimstidt1988). Moreover, the ICSD database (Allen et al., Reference Allen, Bergerhoff and Sievers1987) reports structural data of synthetic Cu10Cd2Sb4S13, based on unit-cell parameters given by Johnson (Reference Johnson1991) and refined on the basis of powder X-ray diffraction data. In that structure refinement, the M(1)–S(1) distance is 2.367 Å, close to that observed in this work for tennantite-(Cd) from Berenguela.
Note that in the structure of synthetic Cu10Cd2Sb4S13, Cd is fully attributed to the M(2) site. An attempt to refine Cd at both the M(2) and M(1) sites in tennantite-(Cd) resulted in a slightly worse R 1 factor (R 1 = 0.0186). Taking into account the crystal-chemistry of Cd2+, a tetrahedral coordination seems more reasonable (e.g. Borsari, Reference Borsari and Scott2014), in agreement with Pattrick (Reference Pattrick1978), who discussed the replacement of Zn and Fe by Cd in Cd-rich tetrahedrite samples from Scotland, and with Charnock et al. (Reference Charnock, Garner, Pattrick and Vaughan1989), who confirmed the tetrahedral coordination of Cd by extended X-ray absorption fine structure (EXAFS). The bond-valence sum (BVS) at the M(1) site is 1.64 valence units (vu), higher than the theoretical value, 1.33 vu (Table 8). Such an overbonding has been observed in other members of the tetrahedrite group, as in tetrahedrite-(Hg) (1.64 vu) and tennantite-(Hg) (1.68 vu) (Biagioni et al., Reference Biagioni, Sejkora, Musetti, Velebil and Pasero2020b; Reference Biagioni, Kasatkin, Sejkora, Nestola and Škoda2021), as well as in tetrahedrite-related phases like arsiccioite (Biagioni et al., Reference Biagioni, Bonaccorsi, Moëlo, Orlandi, Bindi, D'Orazio and Vezzoni2014). Probably, this discrepancy is due to inaccurate bond parameters for Cd–S bonds. Indeed, using the bond parameters of Brese and O'Keeffe (Reference Brese and O'Keeffe1991), the following ideal bond distances for tetrahedral coordination can be calculated (in Å): Cu+ 2.373, Cd2+ 2.546 and Zn2+ 2.346 Å. Assuming the site population discussed above, the average <M(1)–S(1)> bond distance would be 2.416 Å. In greenockite and hawleyite, the observed <Cd–S> distances are 2.528 and 2.526 Å, respectively (Skinner, Reference Skinner1961; Xu and Ching, Reference Xu and Ching1993). Using an average <Cd–S> of 2.527 Å, the calculated M(1)–S(1) distance in tennantite-(Cd) would be 2.411 Å, larger than the observed one.
The M(2) site is split into two sub-positions, M(2a) and M(2b), separated by 0.84 Å, whereas the distance between two neighbouring M(2b) positions is 1.68 Å. These distances can be compared with those reported in other refinements of the crystal structure of tennantite-series minerals, e.g. in Cu-rich unsubstituted tennantite described by Makovicky et al. (Reference Makovicky, Karanović, Poleti, Balić-Žunić and Paar2005) or in tennantite-(Cu) (Biagioni et al., Reference Biagioni, Sejkora, Moëlo, Marcoux, Mauro and Dolníček2022a). The M(2a) position has a triangular planar coordination, whereas M(2b) has a flat trigonal pyramidal one. Average bond distances are 2.230 and 2.383 Å for M(2a) and M(2b) positions, respectively. A comparison of these distances can be made with those reported in Cu-pure and unsplit M(2) sites in tennantite (Wuensch et al., Reference Wuensch, Takéuchi and Nowacki1966), i.e. 2.240 Å, as well as those in split positions observed in tennantite-(Cu), i.e. 2.230 and 2.307 Å (Biagioni et al., Reference Biagioni, Sejkora, Moëlo, Marcoux, Mauro and Dolníček2022a), and in the Cu-excess tennantite-(Cu) described by Makovicky et al. (Reference Makovicky, Karanović, Poleti, Balić-Žunić and Paar2005), i.e. 2.217 Å for Cu2A and 2.467 Å for Cu2B. Moreover, the Cu2B of Makovicky et al. (Reference Makovicky, Karanović, Poleti, Balić-Žunić and Paar2005) is also close to the As site, 2.41 Å, whereas the M(2b)–X(3) distance is 2.695 Å in the sample from the Berenguela mining district. Probably, the relatively large <M(2b)–S> bond distance is due to the average nature of this position. An unconstrained refinement of the s.o.f. at the M(2a) and M(2b) positions indicated full occupancy of the M(2) site by Cu, with no evidence of the possible occurrence of Cu excess or of any other element heavier than Cu (e.g. Cd). The BVS at the M(2) site [i.e. M(2a) + 2×M(2b)] (Table 8) is 1.04 vu, in agreement with the presence of monovalent cations.
The X(3) site shows an average bond distance of 2.265 Å, in agreement with its As pure nature; BVS is 2.97 vu (Table 8).
Both S sites were found fully occupied by S; the BVS at the S(1) and S(2) sites are 2.15 and 2.16 vu, respectively.
Coupling the results of the crystal structure refinement and the electron microprobe analysis, the structural formula of holotype tennantite-(Cd) can be written as M (2)Cu6.00M (1)(Cu4.00Cd1.55Zn0.45)X (3)As4.00S(1)S12S(2)S.
Relation between Cd content and unit-cell parameters
The unit-cell parameter a of tennantite-(Cd) is ~10.31 Å. Charlat and Lévy (Reference Charlat and Lévy1975) observed that the Zn-to-Fe substitution does not affect the unit-cell parameter of tetrahedrite-group minerals; consequently, the increase of the a parameter with respect to that of ideal tennantite-(Fe/Zn), ~10.23 Å, is due to the occurrence of Cd2+.
Pattrick and Hall (Reference Pattrick and Hall1983) observed an increase in the unit-cell parameter of 0.06 Å per Cd atom replacing (Zn/Fe) in synthetic tetrahedrite. Assuming that this relation can be applied to the As-isotype tennantite, the expected unit-cell parameter of the sample studied should be a ≈ 10.32 Å, in reasonable agreement with the observed one. Johnson et al. (Reference Johnson, Craig and Rimstidt1987) proposed the following relation between chemistry and unit-cell parameter (for synthetic tetrahedrites, as no data were available for natural Cd-bearing tetrahedrites): a (Å) = 10.381 + 0.039 Ag + 0.003 Ag2 – 0.019 Cu* + 0.064 Cd – 0.037 As, where Cu* = [2 – (Fe + Zn + Hg + Cd)]. The calculated unit-cell parameter, assuming the idealised chemical formula Cu6[Cu4(Cd1.55Zn0.45)]As4S13, is a = 10.33 Å.
Cadmium in tetrahedrite-group minerals
Currently, several members of the tennantite series have been reported: tennantite-(Fe), tennantite-(Zn), tennantite-(Hg), tennantite-(Cu) and tennantite-(Ni) (Biagioni et al., Reference Biagioni, George, Cook, Makovicky, Moëlo, Pasero, Sejkora, Stanley, Welch and Bosi2020a, Reference Biagioni, Kasatkin, Sejkora, Nestola and Škoda2021, Reference Biagioni, Sejkora, Moëlo, Marcoux, Mauro and Dolníček2022a; Wang et al., Reference Wang, Chen, Gu, Yang, Hou, Fan, Ye and Qu2021). Tennantite-(Cd) is another addition to this series and its occurrence is in keeping with the similar crystal-chemical behaviour of Zn and Cd. Probably, the rarity of Cd-bearing tetrahedrite-group minerals may be related not only to the low Cd abundance but also to its preference to being partitioned in chalcopyrite and sphalerite; indeed Cd contents are higher in those tetrahedrite-group mineral samples crystallising in sphalerite- and chalcopyrite-free assemblages or in unusually Cd-enriched environments (George et al., Reference George, Cook and Ciobanu2017).
Notwithstanding this rarity, some authors reported the occurrence of Cd-rich tetrahedrite-group minerals. Pattrick et al. (Reference Pattrick1978) found up to 1.92 Cd apfu in samples from Tyndrum, Scotland, UK, whereas Voudouris et al. (Reference Voudouris, Spry, Sakellaris and Mavrogonatos2011) reported 1.97 Cd apfu from the Evia Island, Greece, and Jia et al. (Reference Jia, Fu, Zhang and Zhao1988) measured 1.85 Cd apfu in a sample from Xitieshan, China. These latter authors actually gave the formula (Cu6.95Ag3.03)Σ9.98(Cd1.85Zn0.15Fe0.15)Σ2.15(Sb4.19As0.25)Σ4.44S13; taking into account the partitioning of Ag at the M(2) site, this formula could represent an intermediate composition between the hypothetical end-members Ag6(Cu4Cd2)Sb4S13, i.e. ‘argentotetrahedrite-(Cd)’, and Cu6(Cu4Cd2)Sb4S13, i.e. ‘tetrahedrite-(Cd)’. The former potential mineral phase was also described by Voropayev et al. (Reference Voropayev, Spiridonov and Shchibrik1988) from the Ushkatyn-III deposit, in central Kazakhstan. Their chemical analyses has Cd contents ranging between 0.93 and 0.99 apfu (recalculated on the basis of ΣMe = 16 apfu), Ag ≈ 3.1 apfu, and a Sb/(Sb+As) atomic ratio of 0.96. Dobbe (Reference Dobbe1992) reported electron microprobe data corresponding to composition varying between argentotetrahedrite-(Fe), ‘argentotetrahedrite-(Mn)’, and ‘argentotetrahedrite-(Cd)’.
Škácha et al. (Reference Škácha, Sejkora, Palatinus, Makovicky, Plášil, Macek and Goliáš2016) described ‘hakite’ from Příbram, Central Bohemia (Czech Republic), and stressed the occurrence of different compositions characterised by the dominance of Hg2+, Zn2+, or Cd2+. They indicated these different compositions as ‘Hg-hakite’, ‘Zn-hakite’, and ‘Cd-hakite’, ideally Cu6(Cu4Hg2)Sb4Se13, Cu6(Cu4Zn2)Sb4Se13 and Cu6(Cu4Cd2)Sb4Se13, respectively. The crystal structure of ‘Hg-hakite’ was solved through electron diffraction tomography, confirming the isotypic relations with tetrahedrite and the occurrence of Hg2+ at the M(1) site.
Conclusion
Tennantite-(Cd) is a new member of the tetrahedrite group, further showing the role of these sulfosalts in hosting several transition elements typically occurring in hydrothermal environments. The recent revision of the nomenclature of the tetrahedrite group (Biagioni et al., Reference Biagioni, George, Cook, Makovicky, Moëlo, Pasero, Sejkora, Stanley, Welch and Bosi2020a), approved by the International Mineralogical Association Commission on New Minerals, Nomenclature and Classification (IMA–CNMNC), promoted several studies on these sulfosalts, with the description of many different new mineral species and an increasing understanding of their crystal chemistry. The new life of this important sulfosalt group has implications in both the environmental and technological fields. Indeed, tetrahedrite-group minerals host not only economically valuable metals but also some environmentally significant species, e.g. As, Sb, Hg and Cd, and some recent studies focused on the weathering of these minerals and the fate of the potentially toxic elements (e.g. Majzlan et al., Reference Majzlan, Kiefer, Herrmann, Števko, Sejkora, Chovan, Lánczos, Lazarov, Gerdes, Langenhorst, Borčinová Radková, Jamieson and Milovský2018; Keim et al., Reference Keim, Staude, Marquardt, Bachmann, Opitz and Markl2018). Moreover, the improved knowledge of their crystal chemistry is useful in evaluating the high-tech properties of these compounds (e.g. Chetty et al., Reference Chetty, Bali and Mallik2015; Levinsky et al., Reference Levinsky, Candolfi, Dauscher, Tobola, Hejtmánek and Lenoir2019).
The description of tennantite-(Cd) shows both kinds of implications, owing to the presence of the toxic element Cd (e.g. Genchi et al., Reference Genchi, Sinicropi, Lauria, Carocci and Catalano2020) as well as to its potential electronic properties, similar to those shown by its Sb-counterpart Cu10Cd2Sb4S13 (e.g. Kumar et al, Reference Kumar, Chetty, Rogl, Rogl, Bauer, Malar and Mallik2016; Ghisani et al., Reference Ghisani, Timmo, Altosaar, Mikli, Danilson, Grossberg and Kauk-Kuusik2022).
Finally, the identification of the Cd-member of the tennantite series in the Berenguela mining district suggests that the re-examination of tetrahedrite-group mineral samples from this area could be interesting, improving our knowledge on the crystal chemistry of Cd-rich members of this sulfosalt group.
Acknowledgements
CB acknowledges financial support from the Ministero dell'Istruzione, dell'Università e della Ricerca through the project PRIN 2017 “TEOREM – deciphering geological processes using Terrestrial and Extraterrestrial ORE Minerals”, prot. 2017AK8C32. The study was also financially supported by the Ministry of Culture of the Czech Republic (long-term project DKRVO 2019-2023/1.II.d; National Museum, 00023272) for JS. The comments of Peter Leverett, Yves Moëlo, and an anonymous reviewer improved the original manuscript.
Supplementary material
To view supplementary material for this article, please visit https://doi.org/10.1180/mgm.2022.61
Competing interests
The authors declare none.