INTRODUCTION
Shoe soles are almost universally contaminated with infectious microorganisms including many that cause hospital-acquired infection [Reference Agarwal, Hamilton-Stewart and Dixon1, Reference Alam2]. Our research group has recently reported that up to 40% of shoe soles in non-healthcare settings are contaminated with toxigenic Clostridium difficile [Reference Alam2]. Several others have shown that shoe soles are a source of contamination of floor surfaces and environmental ground contamination in the hospital and non-hospital setting [Reference Amass3–Reference Eisenberg7]. For example, a study in rural Alaska asked participants to walk on a pre-determined pathway to a local community healthcare centre wearing pre-sterilized boots [Reference Chambers8]. Upon entering the building, the participants stepped onto a clean piece of sterilized linoleum. Coliforms including Escherichia coli were present on all boots at the end of the walk and approximately half of the linoleum flooring walked on was also contaminated with these microorganisms. Earlier studies have demonstrated that redistribution of bacteria into the air from the operating-room floor accounts for up to 15% of all airborne bacteria and walking on contaminated floors was a more effective airborne dispersal method than either mopping or sweeping [Reference Hambraeus, Bengtsson and Laurell9]. Despite several apparent mechanisms by which contaminated floors or environmental ground contamination could be a vector for human infections, the transmission dynamics between environmental surfaces and humans have not been systematically reviewed. Thus, this systematic review sets out to assess methods by which floor surfaces or environmental ground contamination can serve as vectors for infectious diseases.
METHODS
The systematic review used the preferred reporting items of PRISMA to address the study objectives [Reference Moher10]. To be eligible for inclusion, studies had to either directly or indirectly assess infectious microorganism transmission from floor surfaces (hospitals or other healthcare centres) or soil (community) directly to human contact or indirectly to a secondary vector that could transmit the microorganism closer to patient contact. Studies were excluded if they investigated (1) questions not related to modes of transmission of infectious organisms from floors or soil, (2) were infection control or prevention or outbreaks, (3) diversity, geographical distribution, pathology or microbiology of bacterial, viral or fungal agents, (4) genetic or metabolic pathways of infectious agents, (5) disease pathophysiology, diagnosis and treatment in humans, (6) immunization or gene therapies, (7) genetic, modelling, or in vitro research, (8) non-human (plant or animal) infections or (9) were not original research. Only relevant articles published in English between 1946 and 15 September 2016 with available full texts were included in the final review. To maximize the sensitivity to identify relevant articles, the medical databases Medline (1946 to present), PubMed (NLM) and EMBASE (1947 to present) were searched using a broad set of concepts and MeSH terms. MeSH terms included bacteria, virus, fungi, fomites, shoes, disease transmission, reaerosolization, dispersal and dispersion. A complete search strategy for each database (Medline, PubMed, EMBASE) is given in Supplementary Table S1. Scopus (Elsevier) as well as the bibliographies of identified articles were searched for additional studies not found using the initial database search. An auto alert service was set up in Medline (Ovid) for notification of any new articles indexed matching the search terms. An update literature search was done on 15 September 2016 to look for any new related articles being published after the initial database search. Once the search was complete all the citations were uploaded to a citation manager. An online bibliographical management program (Refworks; www.refworks.com/refworks2) was used to manage citations including removal of the internal and external duplicates within and among the databases. A custom MS Excel workbook (Microsoft Corp, USA) for systematic review was designed and used to screen abstracts [11]. Data were abstracted using an Excel file with pre-determined data fields, and was developed, pilot-tested on a random sample of 15 titles, and revised accordingly by the authors. All abstracts and full-text articles were independently reviewed and data were abstracted by two authors (T.R., I.H.) and discrepancies resolved by consensus. Information included author, year of publication, country, study design, study setting, method and findings. Data for findings included source of contamination, contaminant, microbial concentration, measures of association and any other related factors. All data were integrated into a descriptive summary table (Table 1). The primary outcome measure was percentage of microbiological contaminant after contact (directly or indirectly) from floor or ground surfaces.
Table 1. Transmission dynamics from floor surfaces or environmental ground contamination to cause human infection

Obs., Observational; Exp, experimental; HAI, healthcare-associated infection; OR, odds ratio; MCP, microbe-containing particle; ESBL, extended-spectrum beta-lactamase.
* Arthropods included cockroaches, ants, and other insects.
Quality control during article screening was achieved through (1) a database search conducted by an experienced author, (2) a high Cohen's kappa for agreement between the two authors screening the abstracts, (3) independent searching of all the abstracts and titles by two authors, (4) screeners were blinded to the study author, and (5) independent review of all full-text articles by the two authors. Methodological quality, sample processing, and outcome was used to ascertain the quality of the studies.
RESULTS
An initial search of Medline, PubMed and EMBASE databases using the key words and MeSH terms yielded 9134 citations. After removal of external and internal duplicates, 5678 unique citations were identified and following screening of the titles and abstracts, 5503 articles were excluded. This yielded 175 full-text articles which were assessed for eligibility and data abstracted; 152 of these did not meet the inclusion and exclusion criteria and were discarded. An additional seven articles which met the selection criteria were identified through bibliographical search of the selected articles and were included in the review, giving a final total of 30 articles which met all study criteria (Table 1). A Cohen's kappa of agreement between the two reviewers was 96% [Cohen's kappa for inter rater reliability κ = 0·96]. The detailed article selection process is outlined in the PRISMA [Preferred Reporting Items for Systemic Reviews] flowchart in Figure 1. Methods of disease transmission were categorized as direct or indirect pathways. Direct pathways included the transmission mechanisms resulting in direct contact of human beings with microorganisms on floor or ground surfaces. Indirect pathways were defined as mechanisms that were not directly related to human contact of floor or ground surfaces but included vectors that could lead to human infection via a secondary transmission event. Of the 30 articles (published between 1968 and 2015) included in the review, 19 examined direct pathways of transmission and 11, indirect pathways; 20 were observational and 10 were experimental studies. Most studies were from the USA or Europe.
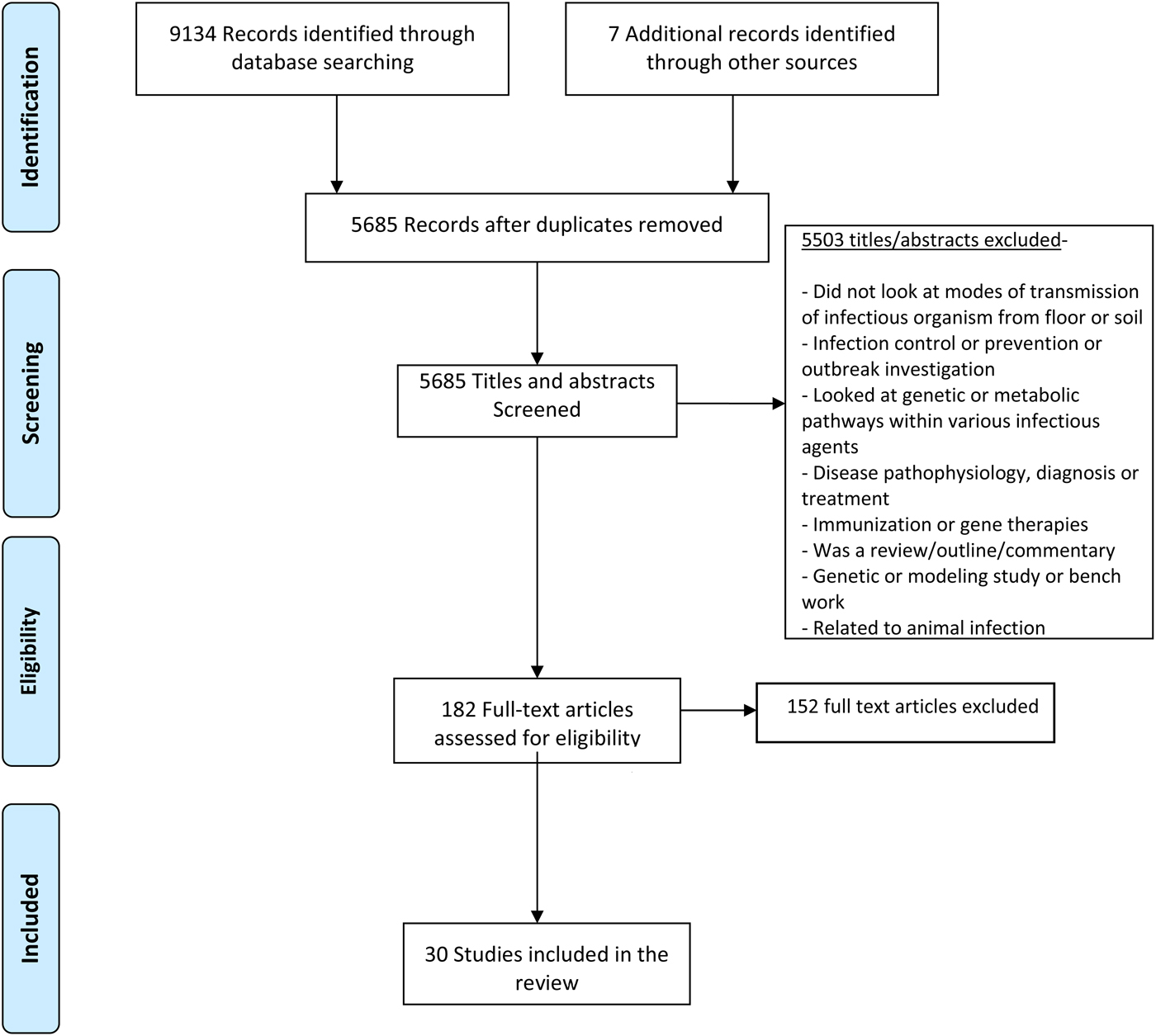
Fig. 1. PRISMA flowchart for screening and evaluation of studies looking at transmission dynamics.
Direct pathways of transmission
Direct human contact
Identified direct pathways included human activities directly related to touching of floor surfaces or aerosolization from contaminated floor surfaces to contact humans. Eight of nine direct human contact studies involved an actual or simulated hospital setting. A number of variables were associated with airborne microorganism contamination including number of visitors and human activities to a patient's room [Reference Verde12], number of persons and person movement in the operating room [Reference Andersson13], and presence of dirt (either soil or water) in hospital areas [Reference Limmathurotsakul14]. Carpets also yielded higher microbial concentrations per square inch compared to bare floors in a paediatric patient's room [Reference Anderson15].
Experimental studies investigated walking over contaminated flooring (two studies) or floor cleaning to prevent infection. Both studies that assessed walking identified increased contamination of either the surrounding floor [Reference Whyte16] or airborne contamination [Reference Buttner17] and varied by the type of flooring with cut pile carpet showing greater contamination than vinyl and loop pile carpet. Another experimental study [Reference Gwaltney and Hendley18] demonstrated reduced numbers of microorganisms on the finger tips following touching of artificially contaminated untreated tiles (61%) compared to tiles treated with an antiviral compound (21%). Finally, an observational study undertaken on two naval vessels identified a direct positive association between personnel concentration with bacterial concentration [Reference Wright, Vaichulis and Chatigny19]. Thus, all human contact studies showed that activities such as walking, touching or contact with floor surfaces are important factors for dispersal of pathogenic microorganisms.
Aerosolization
Eleven studies investigated transmission of floor or ground microorganisms by aerosolization. Two of the studies were performed in hospitals, four in the community, two in animal workers, and three in the laboratory. Aerosolization methods included airborne dispersal, and aerial and dust dissemination. In the hospital studies sporadic airborne dissemination of C. difficile in the hospital environment was identified during routine cleaning procedures and aerosolization was linked with the persistence of the bacteria on environmental surfaces [Reference Roberts20]. Hambraeus et al. [Reference Hambraeus, Bengtsson and Laurell9] investigated various modes of airborne dispersal of contaminated hospital-floor bacteria and showed that walking led to the highest dispersal of Staphylococcus aureus and other microorganisms at levels three-fold higher than found with heating, ventilation and air conditioning (HVAC) ventilation, and 17-fold higher than with floor mopping. Active air movement in the room (HVAC) increased persistence of bacterial particles in the environment from 1·9 h without ventilation to 14·3 h with ventilation. These results were corroborated by three community-based studies [Reference O'Connor, Tribe and Givney21–Reference Bródka25] in which airborne dispersal of pathogenic bacteria was identified in a university classroom, retail outlets, and office suites, and all studies recorded increased human movement as significant predictors of contamination. Notably, two of the retail outlet studies found the airborne bacterial load to be significantly related to the number of visitors attending the outlet [Reference Lues, Theron and Van24, Reference Bródka25].
Three experimental studies investigated the effect of flooring type on aerosolization (n = 1), and vertical dispersal of fungal or bacterial spores (n = 2) [Reference Gόrny and Dutkiewicz26–Reference Weis28]. Carpet was more likely to cause aerosolization than PVC flooring along with increased traffic of persons over the flooring. Aerosolization probability increased with higher air velocity, type and texture of surfaces, and number, size and type of microorganism. Greater than 80% of aerosolized bacterial particles were within the alveolar respirable size [Reference Weis28].
Indirect pathways of transmission
Arthropod-borne transmission
Five observational studies (four in hospitals) investigated arthropod-borne transmission of microorganisms [Reference Aquino29–Reference Fotedar and Banerjee32] and in chicken broiler farms [Reference Solà-Ginés33]. The hospital-based studies were performed in Brazil, Taiwan, India, and Czechoslovakia and examined the presence of healthcare-acquired organisms on the surfaces of arthropods. Healthcare-associated pathogens were identified in arthropods in all studies. Arthropod- and cockroach-borne transmission of bacteria was linked in two studies with nosocomial infection and spread of multidrug-resistant organisms [Reference Pai, Chen and Peng31, Reference Fotedar and Banerjee32]. In the community setting, flies in broiler houses were found to be contaminated with genetically identical E. coli strains producing extended-spectrum beta-lactamases which were also present in the farm environment [Reference Solà-Ginés33].
Six studies investigated indirect pathways of transmission of environmental microorganisms from plants, water, and soil to human infection. For example, strawberries grown in virus-contaminated soil resulted in internalization of the virus into the internal surface of the strawberry and it was postulated that the virus could then enter the human food chain and cause infection despite adequate washing of the external surface prior to eating [Reference DiCaprio, Culbertson and Li34]. Rain splash due to irrigation was also shown to cause local dispersal of contaminated soil to crops or other fresh produce [Reference Gagniere, Auvray and Carpentier35–Reference Penet38].
DISCUSSION
Transmission dynamics of infectious diseases from the environment are complex. Our research group has previously demonstrated that shoe soles are often highly contaminated with C. difficile [Reference Alam2], a finding concordant with several other studies showing the presence of pathogenic microorganisms on shoe soles [Reference Alam2, Reference Rashid39]. Such contamination can readily spread to the floor or ground surface through walking and so we decided to assess possible modes of transmission of infectious agents from such surfaces to human contact to increase the likelihood of human infection. This was achieved through a systematic literature review and to our knowledge this is the first such review to do so. After identifying 30 separate studies, we grouped transmission dynamics into two pathways: (1) that would directly increase the likelihood of human infection or (2) indirectly increase the likelihood via a secondary vector. Studies performed in hospitals, community, and animal farms have all demonstrated the increased likelihood of human infection either via direct contact with the floor surface or via aerosolization (Table 2) as walking over contaminated floors has consistently been shown to aerosolize infectious particles to a height at which respiration of the organism would be possible.
Table 2. Summary of transmission pathways

Despite a large amount of epidemiological and experimental evidence, very few interventional studies were identified but almost all studies demonstrated that increased human movement with walking increased the likelihood of airborne dispersal of microorganisms. This suggests that effective disinfection and cleaning of floors and shoe soles could directly impact on aerosol generation rates. This is supported by Gwaltney & Hendley [Reference Gwaltney and Hendley18] who found that cleaning of floors contaminated with a rhinovirus with a phenol/alcohol disinfectant significantly reduced both the recovery of the virus on the surface and the rate of detection of virus on fingers, as well as transmission and infection of volunteer recipients. For effective antimicrobial cleaning, a broad spectrum disinfecting agent would be required as studies have shown a very wide range of microorganisms including many with multi-drug resistance. This systematic review also suggests that targeted interventions in high-traffic area would likely have the highest probability of decreasing the likelihood of human infection via direct contact or aerosolization from a microbially contaminated environment. Indeed, in rooms contaminated with multidrug-resistant Acinetobacter baumannii, it has been repeatedly shown that up to 25% of rooms may remain contaminated with the organism despite apparent appropriate terminal cleaning [Reference Strassle40]. Moreover, a recent experimental study using a non-pathogenic viral surrogate marker to assess floor contamination also demonstrated the potential of hospital floors to be a source of pathogen dissemination [Reference Koganti41]. Taken together, these findings suggest that there is a high likelihood that contaminated shoe soles entering these rooms shortly after terminal cleaning result in re-contamination of the room. This hypothesis will require future study. According to recent studies, use of novel probiotic-based cleaning interventions or customized ultraviolet radiation disinfection devices may be more effective than chemical disinfection in cleaning rooms of pathogenic organisms [Reference Caselli42, Reference Mathew43].
The strengths of this study include state-of-the-art systematic review techniques including multiple database searches, multiple independent reviewers, and reproducible results, which points the way to future intervention studies. The limitations are that the search strategy was limited to articles identified from Ovid Medline, PubMed and EMBASE and some topic-related articles indexed in other databases may have been missed. Further, studies were heterogeneous in design with regard to sampling strategy, population surveyed, and disinfecting practice, making a meta-analysis of the data not possible. Nevertheless, a consistently high rate of environmental contamination was noted in all studies and future studies are warranted to understand better the probability and frequency of microbial transfer from shoes to flooring surfaces or other areas that impact on the disease transmission model. Certainly, an effective disinfection strategy for shoe soles is urgently needed.
In conclusion, this study identified direct and indirect routes of transmission of infectious microorganisms from floor or ground surfaces to human infection. Interventions including cleaning of floor surfaces and identification of vectors that transfer such organisms such as shoe soles could constitute part of an effective infection control strategy in hospital and community settings.
SUPPLEMENTARY MATERIAL
For supplementary material accompanying this paper visit http://dx.doi.org/10.1017/S0950268816002193.
ACKNOWLEDGEMENTS
This work was supported by a research grant from Healthy Sole LLC.
DECLARATION OF INTEREST
None.