
Introduction
Projectile weaponry, in its broadest terms, is found in all regions and among all human cultures of the world, yet its antiquity and evolution remain incompletely understood (Brooks et al. Reference Brooks, Yellen, Nevell, Hartman, Hovers and Kuhn2006; Shea Reference Shea2006). The earliest proposed evidence for hafted projectiles comes in the form of the approximately 500000-year-old stone points from Kathu Pan in South Africa (Wilkins et al. Reference Wilkins, Schoville, Brown and Chazan2012), thought to have been used as spearheads. The oldest wooden spears are from Schöningen in Germany, dated to around 400000 years ago (Thieme Reference Thieme1997). There is a conceptual distinction between weapons that are projected by hand, such as those from Schöningen, and those that are projected by means of a mechanical instrument, such as a bow or atlatl (Haidle Reference Haidle2010). The bow and arrow is thought to signal higher-level cognitive functioning and is considered a hallmark of complex modern human behaviour. Complex cognition emerged approximately 100kya, and establishing precisely how far back in time we can recognise and verify mechanically assisted projectile use, and more specifically bow-and-arrow technology, is an ongoing theme of research and debate (Shea Reference Shea2006; Villa & Lenoir Reference Villa and Lenoir2006; Wadley & Mohapi Reference Wadley and Mohapi2008; Lombard Reference Lombard2011; Langley Reference Langley2016 and papers therein).
The use of cylindrically modified bones as hafted arrowheads is well established within the historic period of southern Africa, and extends deep into the Later Stone Age (Schapera Reference Schapera1927; Deacon Reference Deacon1976; Lee Reference Lee1979; Parkington Reference Parkington and Kent1998; d'Errico et al. Reference d'Errico, Backwell, Villa, Degano, Lucejko, Bamford, Higham, Colombini and Beaumont2012a; Robbins et al. Reference Robbins, Campbell, Brook, Murphy and Hitchcock2012; Bradfield Reference Bradfield2015). A Middle Stone Age date has been proposed for a single bone point from a Howiesons Poort (HP) layer dated to 61.7±1.5kya BP from Sibudu Cave in South Africa (Backwell et al. Reference Backwell, d'Errico and Wadley2008; Jacobs et al. Reference Jacobs, Roberts, Galbraith, Deacon, Grün, Mackay, Mitchell, Vogelsang and Wadley2008; Backwell & d'Errico Reference Backwell, d'Errico and Langley2016). Small stone segments from the same layers support the use of bow-and-arrow technology at this time, preceded by an established bone-tool manufacturing tradition in pre-Still Bay layers aged 72.5±2.0 kya to 77.2±2.1kya (Lombard Reference Lombard2011; d'Errico et al. Reference d'Errico, Backwell and Wadley2012b).
The Sibudu bone point (Figure 1) comes from square B5d, layer GR. The body of the piece was found in the top ash of hearth c (subsequently renamed hearth 12), while the tip came from below the body in white ash (Figure 2). The specimen represents a large mammal limb bone shaft (of indeterminate species) with an ancient break on the proximal (butt) end. When refitted, it measures 49.34mm in length, and has a maximum width and thickness of 5.51mm and 5.61mm respectively. It is morphologically akin to un-poisoned Type 4 (fixed, not reversible) bone arrowheads used in the twentieth century by San hunters (Schapera Reference Schapera1927; Goodwin Reference Goodwin1945). Manganese coats much of the piece, although exposed areas show ochre-brown discolouration of the type caused by heat. Traces of manufacture consist of clear longitudinal facets covered in places by thin parallel striations produced by a stone tool (Backwell et al. Reference Backwell, d'Errico and Wadley2008). Macroscopic impact fractures diagnostic of longitudinal impact support its use as a weapon component, rather than as a domestic implement (Bradfield & Lombard Reference Bradfield and Lombard2011; Bradfield & Brand Reference Bradfield and Brand2015). In this study, we used high-resolution CT to image the Sibudu bone point and seven experimentally manufactured replicas for comparison. Six of these replicas were experimentally used as arrowheads to evaluate internal damage resulting from impact, while one replica was subjected to heat to assess the possibility that natural desiccation is a confounding variable in eliciting internal damage. The research presented here was undertaken to investigate further the function of the Sibudu bone point.
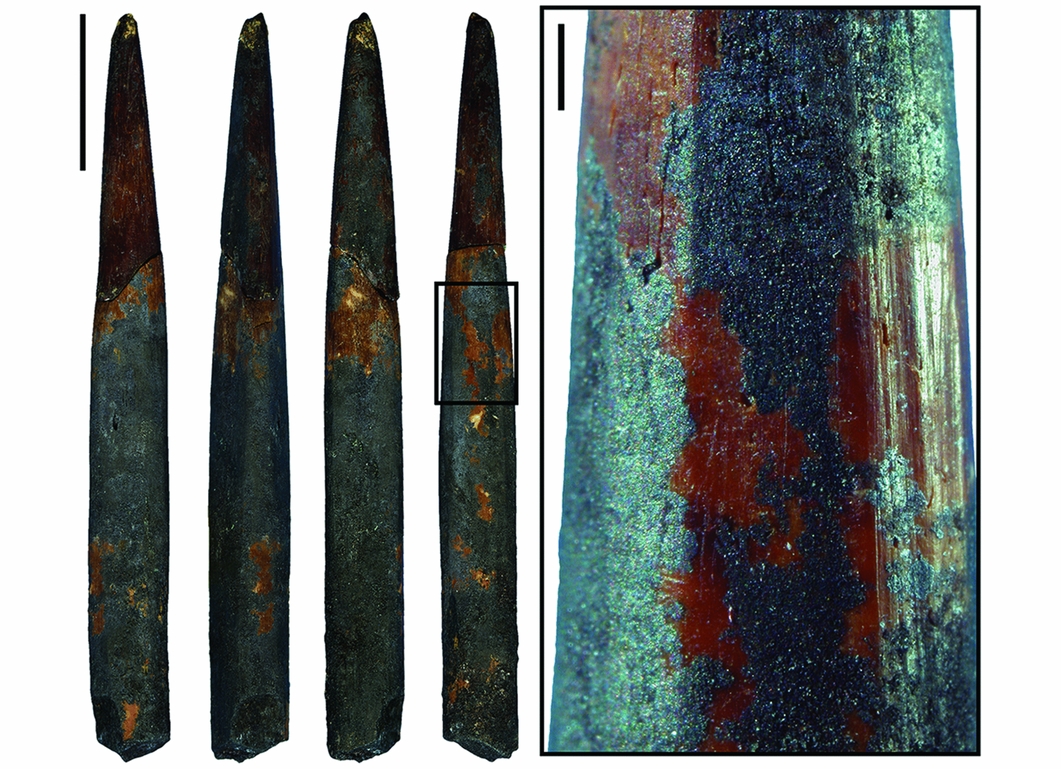
Figure 1. Refitted bone point from Sibudu Cave with a close-up view of the facets produced by a lithic edge. Note the dark brown colour of the surface, most probably created by heat, and the silver-black manganese dioxide coating. Scale bars = 10mm.

Figure 2. Sibudu Cave stratigraphic sequence on the north wall of excavation squares B5 and B6. The Howiesons Poort layers are in grey. The asterisk denotes the position where the bone point was found in layer GR, but 1m in from the profile drawn here. Modified after Wadley (Reference Wadley2007); d'Errico et al. (Reference d'Errico, Backwell and Wadley2012b).
Methods
Bone point replicas
Seven bone point replicas were made to the specifications of the Sibudu bone point. The experimental points were fashioned from cortical midshaft sections of two fresh adult eland (Taurotragus oryx) metacarpals, following Kalahari San practice in northern Namibia. Bone point blanks were cut using a fine band saw (Figure 3a), whittled at one end using a sharp knife (Figure 3b) and shaped and smoothed with a rotary sander. Each replica was scanned at 5µm voxel size resolution prior to the commencement of the experimental protocol (shooting and heating). Scanning prior to the experiments ensured that any pre-existing cracks in the bone resulting from the manufacturing process were known and registered. After the experiments were conducted, each replica was scanned at 5µm voxel size resolution a second time, using the same protocol. In order to ensure maximum comparability, the same scanning equipment and parameters (see below) were used for all scanning episodes.

Figure 3. a) Eland metapodial being cut to produce blanks; b) bone blank being whittled to a point using a sharp knife; c) //ao ≠Oma inserting an experimental bone point into a reed shaft; d) the six experimental bone points hafted into reed shafts; e) //ao ≠Oma preparing his bow by rubbing Grewia flava leaves on the drawstring made of sinew; and f) drawing the bow to shoot.
Hunting experiment
Six replicated bone points (arrows 1–6) were each hafted directly into reed shafts made from Phragmites australis, using animal sinew to secure them in place (Figure 3c & d). A traditional San bow was made from Grewia flava wood and strung with a twisted piece of kudu (Tragelaphus strepsiceros) tendon (Figure 3e). The bow draw was measured at 19 pounds (8.61kg). A complete fresh goat carcass was suspended from a cross-bar in a standing position. An experienced San hunter named //ao ≠Oma conducted the bow-and-arrow experiment. He shot the arrows at the carcass from a distance of 8.6m (Figure 3f), a comparable distance to that recorded during San hunting expeditions (e.g. Hitchcock & Bleed Reference Hitchcock, Bleed and Knecht1997). Due to the light weight of an arrow, he would occasionally move closer to the target (around 7m) before shooting. Most of the shots were on target, striking the carcass in the abdomen (Table 1). The experiment was designed to reproduce realistic frequencies of alteration to the structure of the cortical bone caused by longitudinal impact. //ao ≠Oma did occasionally miss, resulting in arrows landing on the soft sand of an animal enclosure. In one instance, a bone point broke upon hitting a tree (Table 1: arrow 3, shot 15). All other damage to points was caused by contact with the carcass, with the greatest probability of damage caused by striking skeletal elements. The number of shots varied because, even though an arrow could have been used many times more than it was, we decided to stop shooting in case it missed the target and struck the ground or a tree, thereby rendering it unrepresentative of a bone point that had struck an animal.
Table 1. Data from the hunting experiment showing the number of times that each arrow was shot, where it hit the target and from what distance.

Heat experiment
Given that the Sibudu bone point was found in a hearth, a seventh experimental bone point (arrow 7) was subjected to repeated heating, cooling and desiccation in an attempt to simulate the effect that heat has on bone placed in close proximity to a hearth. The bone point was placed on a dish of sand inside a convection oven (near the open door) set at 80°C. Heating events lasted approximately two hours, after which time the bone was submersed in cold water before being replaced in the oven, a procedure repeated three times.
High-resolution CT scanning
We made pre- and post-experimental high-resolution CT scans of all bone point replicas, as well as a single set of scans of the bone point from Sibudu. High-resolution CT scanning was carried out on a Nikon Metrology XTH 225/320 LC dual source industrial CT system housed in the Microfocus X-ray Computed Tomography Facility in the Evolutionary Studies Institute of the University of the Witwatersrand. All bone point replicas were scanned at 70kV and 115µA energy settings using 2000 projections, a 0.8mm copper filter and 5µm voxel size. The bone point from Sibudu was scanned at 60kV and 115µA energy settings using 2000 projections, a 1.2mm aluminium filter and 5µm voxel size. The minor alteration in scanning parameters was to accommodate slight mineralisation of the archaeological bone. All raw data were reconstructed in CT Pro 3D software. We employed minor beam hardening correction (i.e. correction factor pre-set 2) to optimise image quality by removing X-ray related artefacts. Owing to the absolute length of the bone points, the spatial resolution required to view histological features of interest with sufficient clarity and our desire to sample the entirety of each point, we scanned each bone point in a series of overlapping acquisitions (e.g. 7–10 scans per point). The continuous series of scans representing each bone point were aligned and digitally stitched into a single 16-bit unsigned .TIF image stack using VG Studio Max 2.3 software (Volume Graphics GmbH, Heidelberg, Germany).
Pre- and post-experimental bone point image data were registered in the same planes using the two-surface registration protocol in VG Studio Max 2.3. This registration permitted assessment of microcracks and other damage that was present after shooting or heating experiments but absent beforehand. Histological features were observable at the 5µm spatial resolution of registered image stacks. Features of structural damage were identified and differentiated through comparisons with known histological features and vascular patterns (sensu Francillon-Vieillot et al. Reference Francillon-Vieillot, de Buffrénil, Castanet, Géraudie, Meunier, Sire, Zylberberg, de Ricqlès and Carter1990). As no histomorphological studies exist for eland metapodials, we used criteria established from impala (Brits et al. Reference Brits, Steyn and Abbé2014). Long bones of impala (Aepyceros melampus) contain cortical bone consisting of primary vascular arrangements, rounded primary osteons arranged in bands and numerous reticular vascular canals that are evident in the acquired high-resolution CT images (see Figure 4).

Figure 4. Transverse sections of arrows (1,2, 3 & 5) used in the hunting experiments that developed microcracks as a result of impact (see blue arrows). Note that in all cases, except arrow 3, the microcracks generally extend parallel to planes of histological canals. The bottom row presents one or two orthogonal views (F: front, R: right) of longitudinal sections. Note the double cracks occurring on the same plane, most clearly shown in arrow 3, R.
Stress-related features were differentiated from histological ones based on their different orientation, consistency and regularity of crack borders, as well as through comparison with the pre-experiment scans and results reported in previous experiments (e.g. Bradfield Reference Bradfield2013; Bradfield et al. Reference Bradfield, Hoffman and de Beer2016). Stress-related microfractures in cortical bone with a plexiform arrangement propagate along the line of vascular canals, which represent the path of least resistance, and in extreme circumstances these fractures may transect lamellar structures (Wang et al. Reference Wang, Mabrey and Agrawal1998). Orientation, position and linear dimensions of stress-related features were measured using VG Studio Max 2.3 software. The minimum distance from the proximal end of each point to a microcrack was quantified, as was the length and maximum width of each crack (Table 2). Selected microcracks exhibiting characteristic features are illustrated in Figure 4.
Table 2. Microcrack metric data from the experimental and archaeological bone points.
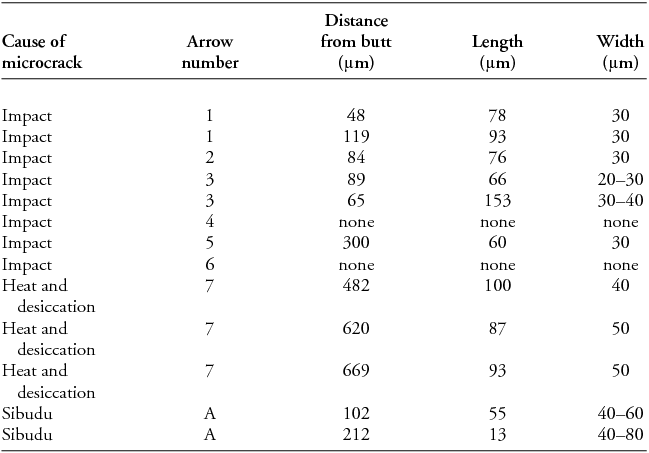
Results
Pre-experimental internal assessment
The pre-experimental images revealed that the only distinguishable microcracks present in the bone replicas prior to the experiments were probably created during manufacture when the bone was cut to the desired length. This damage takes the form of distinctive hash-shaped defects at the base of arrows 1 and 2, and extends approximately 500µm towards the tip in profile length. All of the replicas show plexiform organisation, except arrow 4, which displays only primary vascular organisation. Arrows 2 and 3 exhibit both plexiform and primary vascular organisation, with the latter concentrated closer to the marrow cavity.
Post-experiment internal assessment: hunting arrow bone points
The post-experiment images from arrows 1, 2, 3 and 5 reveal use-related internal damage (i.e. microcracks) near their base (Figure 4). These microcracks are absent from the respective pre-experiment image data, indicating that they were generated during the shooting experiments. Arrows 4 and 6, despite missing the target on several occasions, and striking areas near to bone when they were on target, did not accrue any noticeable damage during the experiment. In the four cases where internal damage was observed (Table 2), microcracks were consistently observed closer to the base of the arrow than the tip. Metric characteristics of the microcracks, such as length, width and initiating distance from the proximal end, are provided in Table 2. All microcracks propagated parallel to the lamellar structural planes of the bone, except in the case of arrow 3, where microcracks transect these structural planes. In addition to striking the spine and shoulder of the carcass, arrow 3 also struck a tree, which probably explains its crack pattern.
Post-experiment internal assessment: heated bone point
The heated bone point exhibited the most numerous microcracks. Four distinct sets of microcracks were observed in a radial arrangement extending inward along two planes from the external surface of the bone (Figure 5). Microcrack propagation transects the vascular structures of the bone and does not propagate along the line of vascular canals, as seen in the experimental arrowheads. The widths of these cracks are slightly wider than those observed in the arrowheads used in the shooting experiments. In profile, the microcracks have a wispy appearance. Observed in transverse section, the four microcracks are of similar lengths and appear to emanate inwards from the exterior surface as the collagen fibres and extracellular matrix contract due to desiccation.

Figure 5. Transverse and two orthogonal longitudinal sections through arrow 7 after the heating experiment. White arrows indicate the location of microcracks. Note the four radially arranged centripetal cracks in the transverse section and the jagged profile of the microcracks in the longitudinal section.
Sibudu point internal assessment
The Sibudu bone point displays what appear to be primary vascular canals (visible where the concentric lamellae have mineralised; Figure 6, lower right), with no trace of a plexiform arrangement. It displays two types of microfractures. The first are four sets of short (1.5–10mm transverse length) cracks that occur in a radial arrangement at different positions along the bone's circumference, and at different levels along the bone's length. These are reminiscent of the microcracks seen in the desiccation experiment. The second type of microcracks are longer and appear to be much older, as they are either partially or completely filled by mineralised material, appearing as dense white lines (Figure 6). Patches of mineralisation occur throughout the length of the piece and are located primarily on one side, possibly indicating the side that was facedown when deposited. This mineralisation is probably due to the absorption of manganese from the sediment, as the outer surface of the point has a thin manganese coating (Figure 1). The second type of microcrack occurs near the base of the point and appears similar to those produced in the hunting experiment, even though the Sibudu piece lacks internal plexiform bone structure.

Figure 6. High-resolution CT images of internal organisation in the Sibudu bone point recovered from the Howiesons Poort layers. On the left are transverse and profile images of one of the larger mineralised microcracks. Note local areas of relative brightness indicative of mineralisation in each image. The images on the right show irregular patches of mineralisation occurring inside the bone.
Discussion
The microcracks that developed in the heated bone point (arrow 7) are similar in form to those that developed in the hunting arrows, except that the desiccation cracks have a jagged-edged or wispy appearance when viewed longitudinally (Figure 5). The most noticeable difference between the two types of microcrack is their frequency and location within the bone points. Microcracks resulting from heat desiccation (arrow 7) are randomly located along the length of the piece, rather than concentrated towards the proximal end. Arrows 1–3 and 5 developed cracks along only one plane, whereas arrow 7 developed cracks along two planes, that is, simultaneously from four directions (Figure 5). Even when more than one microcrack developed in the shot arrows (arrows 1–3), these occur close to one another and are uni-directional, as opposed to a more systematic contraction of bone throughout the piece when subjected to heating/drying. A consistent location of microcracks near the base of bone points used as projectiles probably reflects a point of stress concentration in hafted projectiles. The impact of the arrow against the arrow shaft, and the resultant macroscopic damage, has been observed on twentieth-century bone arrows in the Fourie Collection (Bradfield Reference Bradfield2012; Bradfield et al. Reference Bradfield, Hoffman and de Beer2016). In other hunting experiments, macro-fractures have also been shown to develop close to or at the proximal end of the bone point, in addition to at the tip (Bradfield & Lombard Reference Bradfield and Lombard2011). This is clearly demonstrated in the example of arrow 5, which, despite the tip breaking upon striking the hind leg of the carcass, developed microcracks near the base, rather than at the tip. Apart from minor qualitative differences in the appearance of microcracks, proximity to the butt and linear (vs radial) arrangement seem to be the most reliable means of differentiating impact from desiccation cracks. There is, however, still some degree of overlap. This is seen in arrow 3, whose microcracks do not resemble those of the other shot arrows, but rather cut across the lamella structures of the plexiform bone, reminiscent of those observed in heated arrow 7.
At least two of the cracks displayed in the Sibudu bone point have the same jagged edges seen in arrow 7. Signs of heating and desiccation are not surprising, given its deposition within a hearth. The two remaining microcracks are possibly older than the jagged-edged microcracks, as suggested by being filled with mineralised matrix. These cracks show up as bright areas of higher density in the image data obtained from the bone point (Figure 6). They were observed in the Sibudu specimen at different levels, both close to the current proximal end of the tool, and at different points along the circumference of the bone. Both cracks also display smooth edges reminiscent of the cracks observed in arrows 1–3 and 5. The larger of these two features is slightly curved in cross section.
Reference to the small study undertaken by Bradfield (Reference Bradfield2013) shows no parallels between the Sibudu point and hide piercing, or to incidental damage resulting from trampling or dropping (Pargeter & Bradfield Reference Pargeter and Bradfield2012; Bradfield & Brand Reference Bradfield and Brand2015). Mineralised microcracks present in the Sibudu bone point bear the closest resemblance to those produced through longitudinal impact when hunting, while at least four microcracks appear to have been caused by heat. This combination of features is in accordance with a scenario in which the fresh bone point was used as an arrowhead for hunting, after which it was lost or disposed of in a hearth. Alternatively, the internal damage may have been the result of it being discarded in the dying embers of a small hearth. Its ochre-brown colour (Figure 1) suggests that the Sibudu bone point was lightly burned at a low temperature. This interpretation is supported by the microscopic study of more than 377000 bones from Sibudu's HP and post-HP layers (Clark & Ligouis Reference Clark and Ligouis2010), which showed that, in general, HP bone fragments were lightly burned. It is also possible that the bone point may have been post-depositionally burnt. Stiner and colleagues (Reference Stiner, Kuhn, Weiner and Bar-Yosef1995) demonstrated that bone buried in sediment underneath a hearth can be variably heated and charred, but not calcined. Sievers and Wadley (Reference Sievers and Wadley2008) showed that light burning can take place when items are buried within 50mm of the surface and relatively low temperatures are sustained for some hours. Subsequent scanning of additional pieces of bone from Sibudu Cave reveals that they exhibit a microcrack pattern, which, albeit less pronounced, does not differ significantly from that observed in the experimentally heated and rapidly cooled arrow point. As these pieces would have cooled naturally and slowly, they demonstrate that heat desiccation of bone, no matter whether fast or slow, results in a similar fracture pattern.
Conclusion
Using a multidisciplinary approach that combines actualistic experiments and high-resolution CT technology, we are able to provide criteria that distinguish heated and dried bone points from those used in bow-and-arrow technology. High-resolution CT was used to identify internal damage characteristic of impact in six replicas of the Sibudu bone point. These were used with a bow and arrow to strike a goat carcass, and one additional replica was subjected to heating and drying only. Our results show that microcracks caused by impact are unilinear, on the same plane, tend to follow the vascular structures of the bone, initiate close to the proximal end and occur in close proximity to one another. When viewed in transverse section, they are relatively long, have a width of 20–40μm and an edge that is sharp and well defined. Damage resulting from heat and desiccation exhibits a radial arrangement of microcracks that occur along different planes, transect vascular structures, initiate randomly along the length of the point, are not associated with one another, are relatively short in transverse section, and have a width of 40–50μm and poorly defined wispy edges. These lines of evidence suggest that the microcrack pattern found in the bone arrow point from the HP layers at Sibudu Cave (dated to 61.7±1.5 kya) reflects a taphonomic history that includes longitudinal impact, followed by heating and drying. This study demonstrates that bow hunting was practised in southern Africa long before its appearance in the Eurasian Upper Palaeolithic.
Acknowledgements
This work was supported by the Oppenheimer Memorial Trust, France-South Africa Research cooperation programme, European Research Council Advanced Grant, TRACSYMBOLS number 249587 awarded under the FP7 programme, and the LaScArBx, a research programme supported by the ANR (ANR-10-LABX-52). The South African Department of Science and Technology and National Research Foundation provided funding for the high-resolution CT scanner and virtual imaging facilities at the University of the Witwatersrand. This work was partly supported by the Research Council of Norway through its Centres of Excellence funding scheme, SFF Centre for Early Sapiens Behaviour (SapienCE), project number 262618.
Supplementary material
To view supplementary material for this article, please visit https://doi.org/10.15184/aqy.2018.11