Introduction
Comprehensive Behavioral Intervention for Tics (CBIT) is recommended as a first-line treatment for Tourette syndrome (TS) in children and adults (Andrén et al., Reference Andrén, Jakubovski, Murphy, Woitecki, Tarnok, Zimmerman-Brenner and Verdellen2022; Pringsheim et al., Reference Pringsheim, Okun, Müller-Vahl, Martino, Jankovic, Cavanna and Piacentini2019). The goal of CBIT is to increase the awareness of tics and premonitory urges and to engage in a behavior that is physically incompatible with the tic (Woods et al., Reference Woods, Piacentini, Chang, Deckersbach, Ginsburg, Peterson and Wilhelm2008). While there is strong evidence proving the efficacy of CBIT (Piacentini et al., Reference Piacentini, Woods, Scahill, Wilhelm, Peterson, Chang and Walkup2010; Wilhelm et al., Reference Wilhelm, Peterson, Piacentini, Woods, Deckersbach, Sukhodolsky and Scahill2012), the mechanisms underlying reductions in tic severity produced by this treatment are still poorly understood.
Recently, Essoe, Ramsey, Singer, Grados, and McGuire (Reference Essoe, Ramsey, Singer, Grados and McGuire2021a) reviewed the available evidence related to three potential mechanisms of behavior therapy for tics, namely reinforcement learning, habituation, and cognitive control. Reinforcement learning, which refers to the learning of an association between a behavior and an outcome, is thought to be linked with tic generation (Palminteri & Pessiglione, Reference Palminteri, Pessiglione, Martino and Cavanna2013). Previous CBIT research has hinted toward a possible role of different types of reinforcement learning, such as positive reinforcement (Essoe et al., Reference Essoe, Ricketts, Ramsey, Piacentini, Woods, Peterson and McGuire2021b) and reversal learning (McGuire et al., Reference McGuire, Ginder, Ramsey, Essoe, Ricketts, McCracken and Piacentini2020). More research has been conducted on cognitive control, given that many cognitive control subprocesses are thought to play a role in CBIT: goal selection (awareness of internal and external contexts influencing tic expression), response selection (selection of competing responses), response inhibition (implementation of competing response inhibiting tic expression), and performance monitoring (making adjustments) (Essoe et al., Reference Essoe, Ramsey, Singer, Grados and McGuire2021a; McGuire et al., Reference McGuire, Sturm, Ricketts, Montalbano, Chang, Loo and Piacentini2022). While two studies reported a possible role of response inhibition and switching in CBIT (McGuire et al., Reference McGuire, Sturm, Ricketts, Montalbano, Chang, Loo and Piacentini2022; Petruo et al., Reference Petruo, Bodmer, Bluschke, Münchau, Roessner and Beste2020), two large neuropsychological studies stemming from the initial randomized controlled trials (RCTs) of CBIT (Piacentini et al., Reference Piacentini, Woods, Scahill, Wilhelm, Peterson, Chang and Walkup2010; Wilhelm et al., Reference Wilhelm, Peterson, Piacentini, Woods, Deckersbach, Sukhodolsky and Scahill2012) revealed that baseline cognitive control abilities did not predict CBIT outcome (Abramovitch et al., Reference Abramovitch, Hallion, Reese, Woods, Peterson, Walkup and Wilhelm2017; Chang et al., Reference Chang, McGuire, Walkup, Woods, Scahill, Wilhelm and Piacentini2018). Our group also showed that EEG markers of response inhibition were unrelated to CBIT outcome (Morand-Beaulieu et al., Reference Morand-Beaulieu, Crowley, Grantz, Leckman, Scahill and Sukhodolsky2022). Thus, specific aspects of cognitive control may play a role in CBIT but more research is definitely needed. Finally, there is some evidence supporting the role of habituation as a mechanism in some behavioral therapies such as exposure and response prevention (Hoogduin, Verdellen, & Cath, Reference Hoogduin, Verdellen and Cath1997; Verdellen et al., Reference Verdellen, Hoogduin, Kato, Keijsers, Cath and Hoijtink2008), but it has not been investigated much in CBIT and so far its role remains unconclusive (Houghton et al., Reference Houghton, Capriotti, Scahill, Wilhelm, Peterson, Walkup and Woods2017).
Another process that may be linked with CBIT is voluntary tic suppression. Most people with TS can voluntarily suppress their tics, though tic suppressibility varies widely across individuals (Conelea et al., Reference Conelea, Wellen, Woods, Greene, Black, Specht and Capriotti2018; Ueda, Kim, Greene, & Black, Reference Ueda, Kim, Greene and Black2021). While learning to control tics through CBIT is not the same as voluntarily suppressing them, there may be some parallels between both processes. Once CBIT is learned and put in application, it involves self-initiated techniques aimed toward controlling tic expression, similar to voluntary tic suppression. Both CBIT and tic suppression involve resisting a tic in response to a premonitory urge. Awareness of those urges is a core feature of CBIT (Woods et al., Reference Woods, Piacentini, Chang, Deckersbach, Ginsburg, Peterson and Wilhelm2008) and is also associated with better tic suppression capacities (Matsuda et al., Reference Matsuda, Nonaka, Kono, Fujio, Nobuyoshi and Kano2020). It has also been suggested that behavioral therapies for tics may bolster tic suppression capacities (Specht et al., Reference Specht, Nicotra, Kelly, Woods, Ricketts, Perry-Parrish and Walkup2014).
While the exact mechanism underlying tic suppression is still unclear, it could, just like behavior therapy, rely on a mix of reinforcement learning, cognitive control, and habituation. Prior research has found that tic suppression can be improved with positive reinforcement (Conelea et al., Reference Conelea, Wellen, Woods, Greene, Black, Specht and Capriotti2018). Additionally, some brain imaging studies (although conflicting findings exist; see van der Salm et al., Reference van der Salm, van der Meer, Cath, Groot, van der Werf, Brouwers and Tijssen2018) suggested some overlap between the brain regions involved in tic suppression and those involved in response inhibition (Ganos et al., Reference Ganos, Kahl, Brandt, Schunke, Baumer, Thomalla and Kuhn2014; Peterson et al., Reference Peterson, Skudlarski, Anderson, Zhang, Gatenby, Lacadie and Gore1998). Research on exposure and response prevention suggests that tic suppression may also partly operate through habituation, given that the person suppressing the tics must get used to and tolerate the urges to tic (Verdellen et al., Reference Verdellen, Hoogduin, Kato, Keijsers, Cath and Hoijtink2008; Verdellen, Hoogduin, & Keijsers, Reference Verdellen, Hoogduin and Keijsers2007).
In a recent EEG study, our group identified a brain network in which functional connectivity was increased during tic suppression in children with TS (Morand-Beaulieu et al., Reference Morand-Beaulieu, Wu, Mayes, Grantz, Leckman, Crowley and Sukhodolsky2023). Given that voluntary tic suppression and CBIT may share some common underpinnings, we wanted to assess whether functional connectivity in this network was associated with CBIT outcome. In treatments across several neurodevelopmental and mental health conditions, functional connectivity in networks that are associated with specific mechanisms relevant for that condition or treatment was found to either predict treatment outcome (Baumel et al., Reference Baumel, Lu, Huang, Drysdale, Sweeny, Gong and Strawn2022; Cyr et al., Reference Cyr, Pagliaccio, Yanes-Lukin, Fontaine, Rynn and Marsh2020; Russman Block et al., Reference Russman Block, Norman, Zhang, Mannella, Yang, Angstadt and Fitzgerald2022) or change after treatment (Baumel et al., Reference Baumel, Lu, Huang, Drysdale, Sweeny, Gong and Strawn2022; Izadi-Najafabadi, Rinat, & Zwicker, Reference Izadi-Najafabadi, Rinat and Zwicker2022; Venkataraman et al., Reference Venkataraman, Yang, Dvornek, Staib, Duncan, Pelphrey and Ventola2016).
Therefore, the first objective of this study was to test whether functional connectivity associated with tic suppression would change from baseline to endpoint. We predicted that functional connectivity in the tic suppression network would increase from baseline to endpoint in the CBIT group but not in the treatment-as-usual (TAU) condition. Our second objective was to test whether functional connectivity predicted reduction in tic severity following CBIT. We hypothesized that increased brain connectivity during tic suppression at baseline would predict greater decreases in tic severity at endpoint in the CBIT group.
Methods
Participants
Thirty-two children with TS, aged 8 to 13 years old, participated in a RCT of CBIT v. a TAU control condition (Morand-Beaulieu et al., Reference Morand-Beaulieu, Crowley, Grantz, Leckman, Scahill and Sukhodolsky2022). Inclusion criteria consisted of (1) ages 8–14 years old; (2) DSM-IV-TR diagnosis criteria for TS or chronic tic disorder; (3) unmedicated or on stable medication for at least one month before initiating the study and throughout the duration of the study; (4) YGTSS Total Score > 14 or Total Score > 10 if only motor tics were present; and (5) fluent English speaker. Exclusion criteria consisted of (1) IQ < 80; (2) diagnosis of severe psychiatric disorder that could interfere with participation in the behavior therapy for tics (e.g. bipolar disorder or psychotic disorder); (3) presence of any psychiatric or psychosocial condition (e.g. depression or family discord) requiring initiation of treatment other than that provided in the current study (i.e. medication, family therapy) or change in current medication type or dose; (4) previous treatment with four or more sessions of habit reversal training/CBIT. The study was approved by Yale institutional review board and conducted according to the Declaration of Helsinki. Consent and assent were respectively obtained from parents and children prior to participation in the study. Families also received monetary compensation for their participation in the study assessment and therapy visits. Participants’ characteristics are presented in Table 1.
Table 1. Demographics and clinical characteristics by treatment group at baseline

Note: ADHD, attention-deficit/hyperactivity disorder; CBIT, Comprehensive Behavioral Intervention for Tics; OCD, obsessive-compulsive disorder; ODD, oppositional defiant disorder; s.d., standard deviation.
Procedures
Study design, randomization, and treatment
Full details pertaining to the structure of the RCT can be found in Morand-Beaulieu et al. (Reference Morand-Beaulieu, Crowley, Grantz, Leckman, Scahill and Sukhodolsky2022). In short, eligible participants were randomly assigned to receive 8 sessions of CBIT over a 10-week period or continue their current treatment plan as is (TAU group). In both treatment conditions, children maintained their ongoing treatment and appointments with their treating clinicians. Interventions were administered separately from the present study, based on the specific requirements of children and their parents, as well as the professional judgment of their treating clinician. Participants in both groups were allowed to receive their customary treatment and services, including, but not limited to, school-based services and individual child psychotherapy. Parents were requested to refrain from making any changes to their child's ongoing treatment or starting new treatments for the duration of the study.
EEG recordings
Continuous EEG was recorded at a 250 Hz sampling rate from 128 electrodes (HydroCel Geodesic Sensor Net) referenced online to the vertex electrode (Cz). We used a Net Amps 200 amplifier and Net Station Acquisition software version 4.2.1 (EGI, Inc.) to monitor signal acquisition. The sensor net was soaked in a potassium chloride solution prior to the recording session. Electrode impedance was assessed at or under 40 kΩ before recording. Data were online filtered with a 0.01 Hz high-pass filter and a 100 Hz low-pass filter.
Experimental task
EEG was recorded during three 2-min tic suppression sessions during which children were asked to suppress all tics. They were also asked to keep their eyes open while looking at the computer screen. Recordings took place in a dimly-lit room.
Outcome assessment
Changes in motor and vocal tic severity from baseline to endpoint were assessed by a blinded rater using the Yale Global Tic Severity Scale (Leckman et al., Reference Leckman, Riddle, Hardin, Ort, Swartz, Stevenson and Cohen1989). The YGTSS was administered by a trained clinician. It assesses the severity of tics over the past week. Motor and vocal tics are rated on 6-point scale (from 0 to 5) according to 5 dimensions: number, frequency, intensity, complexity, and interference. Thus, the motor and vocal subscale each range from 0 to 25 and can be combined to obtain the Total Tic Score.
EEG signal treatment
EEG signal preprocessing
EEG recordings were preprocessed using the Maryland Analysis of Developmental EEG (MADE) pipeline (Debnath et al., Reference Debnath, Buzzell, Morales, Bowers, Leach and Fox2020) running on Matlab 2020a. The MADE pipeline, which is based on EEGLAB's (Delorme & Makeig, Reference Delorme and Makeig2004) functions and data structure, involves several steps which are fully described in the Supplement. Overall, signals were bandpass filtered from 1–50 Hz, bad channels were removed and interpolated, independent component analysis was performed to identify and remove non-neural artifact, continuous EEG recordings were epoched in 2-sec segments, residual artifacts in epoched EEG data were removed using a threshold rejection method, and data were re-referenced to the average of all electrodes. The number of valid 2-second epochs (CBIT: baseline: 131.0 ± 36.9, endpoint: 134.3 ± 44.8; TAU: baseline: 132.6 ± 37.6, endpoint: 122.2 ± 58.3) did not significantly differ across conditions or assessments [all F's < 0.42, all p values > 0.40].
Source-based connectivity pipeline
Brain sources were reconstructed with minimum source imaging (wMNE) in Brainstorm (Tadel, Baillet, Mosher, Pantazis, & Leahy, Reference Tadel, Baillet, Mosher, Pantazis and Leahy2011). Electrode positions were co-registered to the MNI-ICBM152 template using three reference points (nasion, left, and right preauricular points). The symmetric boundary element method implemented in OpenMEEG (Gramfort, Papadopoulo, Olivi, & Clerc, Reference Gramfort, Papadopoulo, Olivi and Clerc2010) was used to compute a three-layered (scalp, outer skull, inner skull) head model. The diagonal of the noise covariance matrix was used for source reconstruction. Sources were projected onto the Desikan-Killiany atlas which consists of 34 regions of interest (ROI) per hemisphere.
The phase-locking value (PLV) was computed in Brainstorm and served as our measure of functional connectivity. The PLV reflects the absolute value of the mean phase difference between two signals (Aydore, Pantazis, & Leahy, Reference Aydore, Pantazis and Leahy2013; Lachaux, Rodriguez, Martinerie, & Varela, Reference Lachaux, Rodriguez, Martinerie and Varela1999). It ranges from 0 to 1; larger values reflect stronger coupling between two signals. Prior research has shown that source-reconstructed connectivity using wMNE and PLV performed well against other methods (Hassan et al., Reference Hassan, Merlet, Mheich, Kabbara, Biraben, Nica and Wendling2017; Hassan, Dufor, Merlet, Berrou, & Wendling, Reference Hassan, Dufor, Merlet, Berrou and Wendling2014). The PLV was computed in the alpha band (8–13 Hz) with a Hilbert transform. The PLV was computed at the epoch level and was then averaged across all epochs. It was computed between all 68 ROI of the Desikan-Kiliany atlas yielding a 68 × 68 matrix for each participant at both baseline and endpoint. The subnetwork involved in tic suppression which we identified in a previous study (Morand-Beaulieu et al., Reference Morand-Beaulieu, Wu, Mayes, Grantz, Leckman, Crowley and Sukhodolsky2023) was applied as a mask over connectivity matrices computed at baseline and endpoint. That subnetwork involved 29 connections between 28 ROIs (connections involved in this subnetwork are listed in online Supplementary Table S1). Baseline and endpoint mean functional connectivity across all connections involved in that subnetwork were used as the dependent variables in our analyses. Network visualization was performed with BrainNet Viewer (Xia, Wang, & He, Reference Xia, Wang and He2013).
Statistical analyses
To assess whether functional connectivity during tic suppression changed from baseline to endpoint, we conducted a repeated-measures ANOVA with Functional connectivity as the dependent variable, and with the between-subjects factor Treatment (CBIT and TAU) and the within-subjects factor Time (baseline and endpoint). We also conducted t tests (Bonferroni-corrected) on the individual connections comprised in the tic suppression subnetwork to assess if there were treatment effects that were specific to a given connection.
To test whether functional connectivity differently predicted reductions in motor and vocal tics, we included the YGTSS motor and vocal tic subscales as a factor in our prediction analyses. Thus, we conducted an ANCOVA on reductions in tic severity from baseline to endpoint with the between-subjects factor Treatment (CBIT and TAU) and the continuous predictor Baseline mean functional connectivity.
Results
Results from the RCT have been published elsewhere (Morand-Beaulieu et al., Reference Morand-Beaulieu, Crowley, Grantz, Leckman, Scahill and Sukhodolsky2022). Overall, CBIT had a positive impact on tic symptoms, with YGTSS total tic score decreasing from 23.8 ± 6.0 at baseline to 16.9 ± 4.9 at endpoint in the CBIT group. In the TAU group, average YGTSS total tic score went from 24.4 ± 5.0 at baseline to 24.9 ± 5.0 at endpoint (Table 2).
Table 2. Change in tic severity from baseline to endpoint

The first objective of this study was to assess if functional connectivity increased from baseline to endpoint in the CBIT group relative to TAU. Our analyses revealed no main effect of Time [F (1,29) = 0.05, p = 0.82] and no Treatment by Time interaction [F (1,29) = 0.06, p = 0.81], suggesting that there were no changes in mean functional connectivity during tic suppression from baseline to endpoint (Fig. 1). There were also no treatment-induced changes in functional connectivity for any connection comprised in the tic suppression network [all t values < 1.82, all p values > 0.078].

Figure 1. CBIT did not impact tic suppression-related functional connectivity. BL, baseline; CBIT, Comprehensive Behavioral Intervention for Tics; EP, endpoint; TAU, treatment-as-usual.
Regarding our second objective about predicting CBIT outcome using mean connectivity within the tic suppression network, the interaction between Treatment and Baseline mean functional connectivity was not significant [F (1,27) = 3.14, p = 0.088]. Given that some studies have reported a differential impact on CBIT on motor v. vocal tics (Chen, Wang, Chang, & Hsueh, Reference Chen, Wang, Chang and Hsueh2020; Yates et al., Reference Yates, Edwards, King, Luzon, Evangeli, Stark and Murphy2016), we decided to assess whether tic-related functional connectivity may differentially predict the impact of CBIT on motor and vocal tics. Thus, we conducted an ANCOVA on reductions in tic severity from baseline to endpoint with the between-subjects factor Treatment (CBIT v. TAU), the within-subjects factor Type of tics (motor v. vocal), and the continuous predictor Functional connectivity. We found a Treatment by Type of tics by Functional connectivity interaction [F (1,27) = 4.89, p = 0.036]. Decomposition of this interaction revealed a Type of tics by Functional connectivity interaction in the CBIT group [F (1,14) = 5.82, p = 0.030] but not in the TAU group [F (1,13) = 0.12, p = 0.65]. In the CBIT group, functional brain connectivity during tic suppression at baseline predicted the decrease in vocal tic severity at endpoint [R 2 = 0.35, β = −0.59, t(14) = −2.57, p = 0.015]. However, it did not predict the decrease in motor tic severity [R 2 = 0.03, β = −0.16, t(14) = 0.62, p = 0.55] (Fig. 2).
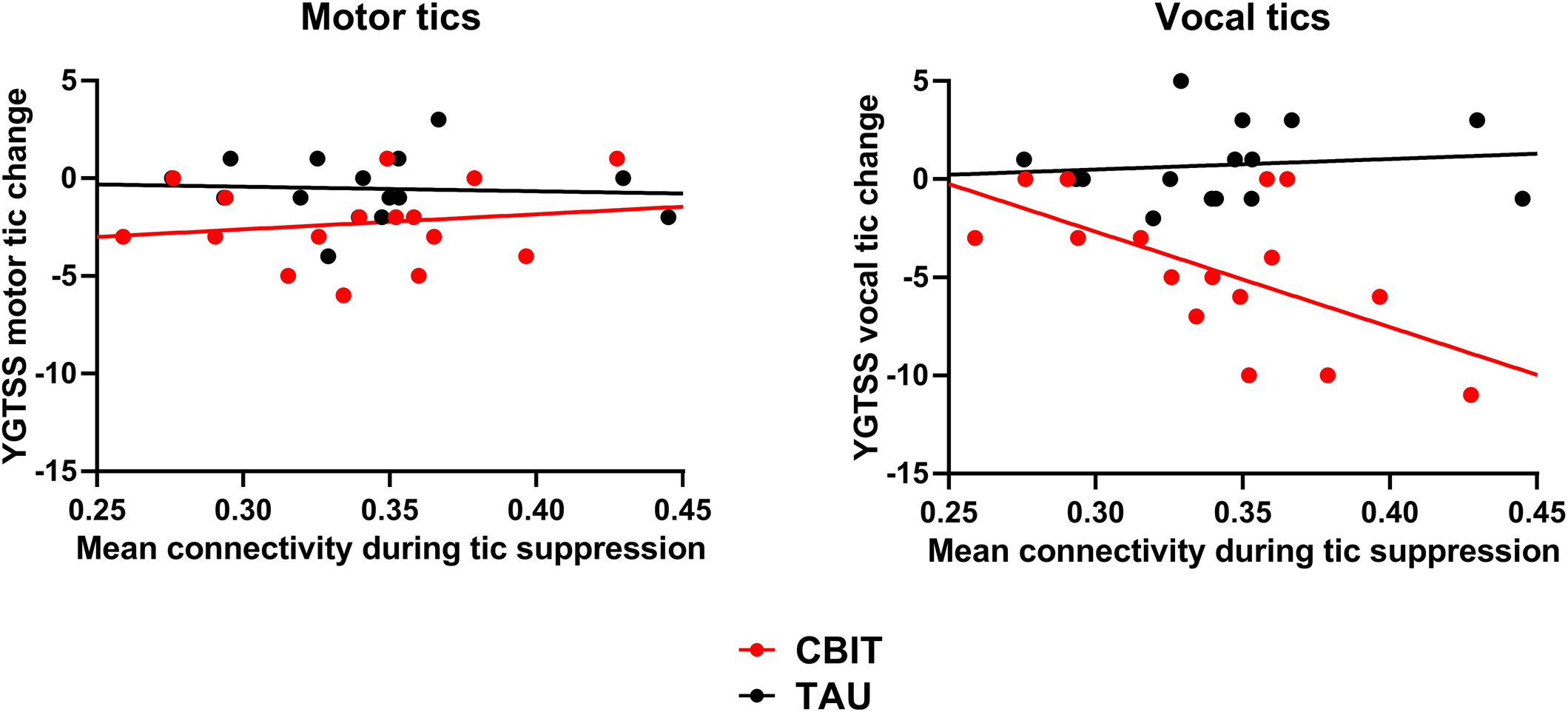
Figure 2. Prediction of CBIT outcome using baseline functional connectivity during tic suppression. Reduction in vocal tic severity following CBIT was predicted by mean connectivity during tic suppression at baseline. However, functional connectivity did not predict the decrease in motor tic severity. CBIT, Comprehensive Behavioral Intervention for Tics; TAU, treatment-as-usual; YGTSS, Yale Global Tic Severity Scale.
Supplementary analyses were performed to identify whether specific connections within the tic suppression subnetwork were mainly responsible for the relationship between baseline functional connectivity during tic suppression and decrease in vocal tic severity post-CBIT. To identify potential associations between improvement in total and motor tic severity, which could have been masked by looking at the mean connectivity of the tic suppression subnetwork, supplementary analyses were conducted with the motor and total tic scales as well. Within the CBIT group, correlations were performed between each of the 29 connections involved in the tic suppression subnetwork and the decrease in vocal, motor, and total tic severity. A Bonferroni-corrected p value of α = 0.05/(29 × 3) = 0.00057 was used to account for the number of correlations. Only one correlation exceeded this threshold. There was a significant correlation between decrease in vocal tic severity and functional connectivity between the right superior frontal gyrus and the right inferior parietal cortex [r(14) = −0.79, p = 0.0003] (Fig. 3).

Figure 3. Association between decrease in vocal tic severity post-CBIT and superior frontal gyrus – inferior parietal cortex connectivity. Supplementary analyses were performed between single connections involved in the tic suppression subnetwork and vocal tic severity decreases in the CBIT group. One connection was significant according to the Bonferroni-corrected significance threshold. Within the CBIT group, decreases in vocal tic severity from baseline to endpoint were predicted by the functional connectivity between the right superior frontal gyrus and the right inferior parietal cortex. A, anterior; L, left; P, posterior; R, right.
Discussion
In the current study, we wished to assess how functional connectivity associated with tic suppression was related to CBIT response. EEG was recorded during three 2-min tic suppression sessions at baseline and endpoint. Brain sources were then reconstructed. We assessed functional connectivity within a subnetwork involved in tic suppression (Morand-Beaulieu et al., Reference Morand-Beaulieu, Wu, Mayes, Grantz, Leckman, Crowley and Sukhodolsky2023) while children with TS were suppressing their tics.
Our analyses revealed that functional connectivity during tic suppression did not change from baseline to endpoint. In CBIT, tics are not directly suppressed but are replaced by competing responses (Piacentini et al., Reference Piacentini, Woods, Scahill, Wilhelm, Peterson, Chang and Walkup2010; Woods et al., Reference Woods, Piacentini, Chang, Deckersbach, Ginsburg, Peterson and Wilhelm2008). Thus, there is no exercise aimed toward enhancing voluntary tic suppression. While it has been proposed that CBIT may bolster tic suppression capacities (Specht et al., Reference Specht, Nicotra, Kelly, Woods, Ricketts, Perry-Parrish and Walkup2014), no study, to the best of our knowledge, has assessed whether voluntary tic suppression capacities were increased following CBIT. If CBIT does indeed have an impact on tic suppression capacities, this impact is not reflected on functional connectivity measures.
While functional connectivity during tic suppression is not altered by CBIT, it seems that it may predict its outcome. Indeed, baseline functional connectivity during tic suppression predicted reduction in vocal tic severity after CBIT. This suggests that strategies used in the voluntary suppression of vocal tics may be relevant during the course of the CBIT training. These results also suggest probable overlap between the mechanisms of voluntary tic suppression and those of behavior therapy for tics. However, in the present study, this effect was only present for vocal tics and not motor tics. In CBIT, competing responses for vocal tics generally consist of ‘controlled breathing’ (Woods et al., Reference Woods, Piacentini, Chang, Deckersbach, Ginsburg, Peterson and Wilhelm2008). Such a technique may involve mechanisms that are more similar to those of voluntary tic suppression than those of competing responses for motor tics, which generally involves a movement or a response that is opposed or incompatible with the tic (Woods et al., Reference Woods, Piacentini, Chang, Deckersbach, Ginsburg, Peterson and Wilhelm2008). Given the similarities between voluntary tic suppression and the management of vocal tics through CBIT, it is possible that children with increased functional connectivity during tic suppression were most likely to show larger decreases in vocal tic severity after CBIT. Another explanation may be related to the somatotopy of voluntary tic suppression. It is known that children are able to selectively inhibit particular tics, and in some contexts (e.g. in the classroom) they may inhibit vocal tics while continuing to have motor tics (Ganos et al., Reference Ganos, Bongert, Asmuss, Martino, Haggard and Münchau2015). Therefore, if children in the current study were more used to suppress vocal than motor tics, the mechanism underlying this suppression may be potentiated by CBIT, which would in turn be associated with less severe vocal tics after treatment. Future studies should assess whether the same brain mechanisms are used to specifically suppress motor and vocal tics.
To the best of our knowledge, only one study directly assessed the link between tic suppression and CBIT. McGuire et al. (Reference McGuire, Sturm, Ricketts, Montalbano, Chang, Loo and Piacentini2022) reported that tic suppressibility (i.e. how much someone can suppress their tics) did not significantly predict the reduction in tic severity following CBIT, although the results were in the expected direction. Furthermore, they did not assess whether CBIT's effect on vocal tics differed from that of motor tics, and associations with vocal tic severity may have been masked by looking at total tic severity. While preliminary, our results suggest that changes in motor and vocal tic severity following CBIT may occur through different mechanisms. Few studies have separately assessed changes in motor and vocal tic severity during the course of therapy, but differences may exist (Shou et al., Reference Shou, Li, Fan, Zhang, Yan, Lv and Wang2022). Our findings highlight the importance of providing separate measures of motor and vocal tic severity in clinical studies.
In our study, supplementary analyses revealed that the observed effect was mainly driven by the connection between the right superior frontal gyrus and the right inferior parietal cortex. In the Desikan-Killiany atlas, the anatomically-defined superior frontal gyrus encompasses the functionally-defined dorso-medial prefrontal cortex (dmPFC), and the inferior parietal cortex region includes both the inferior parietal gyrus and the angular gyrus (Desikan et al., Reference Desikan, Ségonne, Fischl, Quinn, Dickerson, Blacker and Killiany2006). The angular gyrus and the dmPFC are two important regions involved in the default mode network (Andrews-Hanna, Smallwood, & Spreng, Reference Andrews-Hanna, Smallwood and Spreng2014; Gusnard, Akbudak, Shulman, & Raichle, Reference Gusnard, Akbudak, Shulman and Raichle2001). Prior research has suggested that the default mode network may constitute one of the neural substrate of premonitory urges (Ramkiran, Heidemeyer, Gaebler, Shah, & Neuner, Reference Ramkiran, Heidemeyer, Gaebler, Shah and Neuner2019). Awareness of premonitory urges is important for both CBIT and tic suppression. Also, increased default mode network has been associated with reduced tic severity (Fan et al., Reference Fan, van den Heuvel, Cath, de Wit, Vriend, Veltman and van der Werf2018), which is consistent with our finding of larger decreases in vocal tic severity in those with increased baseline functional connectivity.
Finding predictors of treatment response is important from at least two perspectives: identifying individuals for whom CBIT may work well and understanding by which processes reductions in tic severity occur during CBIT. So far, few baseline characteristics may predict who is more likely to strongly benefit from CBIT. Sukhodolsky et al. (Reference Sukhodolsky, Woods, Piacentini, Wilhelm, Peterson, Katsovich and Scahill2017) found that greater baseline tic severity and treatment expectancy were associated with greater reductions in tic severity, whereas the presence of anxiety disorders and greater severity of premonitory urges predicted lesser reductions in tic severity following treatment. However, these effects were also seen in psychoeducation and supportive therapy and were not specific to CBIT. In a study where CBIT was combined with exposure and response prevention, larger obsessive-compulsive symptoms predicted larger decreases in tic severity after treatment (Nissen, Parner, & Thomsen, Reference Nissen, Parner and Thomsen2019). Likewise, not much is known about the mechanisms predicting the outcome of CBIT. Better performance in a neurocognitive measure combining interference control and cognitive flexibility has been shown greater reductions in tic severity (McGuire et al., Reference McGuire, Sturm, Ricketts, Montalbano, Chang, Loo and Piacentini2022), suggesting that cognitive control may play a role in CBIT. Our study adds to this knowledge by identifying another process linked with treatment outcome. Such results are relevant for future studies aiming at better understanding the mechanisms of CBIT.
The findings of this study must be interpreted in the context of some limitations. First, our sample size was small, and our results need to be replicated in larger samples. Second, while using EEG allows the assessment of the synchronization of brain oscillations in a fast frequency band (alpha; 8–13 Hz), using fMRI would allow a more precise localization of the brain regions involved in tic suppression and CBIT response, compared with source-reconstructed EEG. Third, our hypotheses were based on potential parallels between tic suppression and behavior therapy. Thus, we decided to focus on a single subnetwork which we know is involved in tic suppression. Future studies should assess other known brain networks to better understand the role of functional connectivity in CBIT. Fourth, this study did not include a tic frequency count to assess the degree of tic suppressibility. This is in contrast with studies using the Tic Suppression Task from Woods and Himle (Reference Woods and Himle2004), which includes a behavioral index of tic suppression and reinforcement for not ticcing. Future studies investigating the brain correlates of tic suppression should use an observational system with established reliability to assess the degree of tic suppressibility (Black, Koller, & Black, Reference Black, Koller and Black2021; Sturm et al., Reference Sturm, Ricketts, McGuire, Lerner, Lee, Loo and Piacentini2021). Finally, functional connectivity in the current study was specifically assessed during tic suppression. It would be interesting to assess functional connectivity during tasks designed to measure other plausible mechanisms of CBIT.
Supplementary material
The supplementary material for this article can be found at https://doi.org/10.1017/S0033291723001940
Acknowledgements
We thank all children who participated in this study, as well as their family.
Financial support
This project was supported by NIMH grant K01MH079130 to DGS. SMB was supported by a postdoctoral fellowship award from the Canadian Institutes of Health Research (MFE164627) and by the Clinical Research Training Scholarship in Tourette syndrome from the Tourette Association of America and the American Brain Foundation, in collaboration with the American Academy of Neurology. The funding organizations played no role in collection, analysis and interpretation of data and in the writing of the manuscript. The authors have no biomedical financial interests or potential conflicts of interest to report.
Ethical standards
The authors assert that all procedures contributing to this work comply with the ethical standards of the relevant national and institutional committees on human experimentation and with the Helsinki Declaration of 1975, as revised in 2008.