Impact statement
The future of the world’s coasts will be influenced by ongoing sea-level rise and forthcoming storms, which will combine to increase the likelihood for coastal flooding and erosion. However, many coastal settings receive natural supplies of sediment from adjacent rivers and landscapes, and these sediments can physically build up coastal landforms and reduce the potential for erosion and flooding. It is very important to better understand sediment delivery to the coast, as this is one of the key factors in assessing coastal climate change impacts. The delivery of new sediment to the coast commonly has long intervals with very little sediment discharged punctuated by brief events with tremendous sediment discharge. These sediment input events are generally caused by disturbances in the landscape, which can include wildfires, earthquakes, heavy rainfall, volcanic eruptions and human impacts. Over decades to centuries, infrequent, high-magnitude sediment input events can be responsible for most of the sediment that exists along the coast. Climate change has the potential to increase sediment discharge to some coastal settings because of its imminent effects on wildfire, heavy rainfall and the extent of frozen areas within high altitudes and in polar regions. Because of this, there is an increased need to understand the amount of sediment input to coastal regions both now and in the future. Teams of scientists will be needed to monitor and predict future sediment inputs with an eye on better understanding how the coast will respond to climate change.
Introduction
Coastal landforms and the human communities and natural resources located on these features are increasingly vulnerable to flooding and erosion because of ongoing and accelerating eustatic sea-level rise (FitzGerald et al., Reference FitzGerald, Fenster, Argow and Buynevich2008; Syvitski et al., Reference Syvitski, Kettner, Overeem, Hutton, Hannon and Brakenridge2009; Vitousek et al., Reference Vitousek, Barnard, Fletcher, Frazer, Erikson and Storlazzi2017; Schuerch et al., Reference Schuerch, Spencer, Temmerman, Kirwan, Wolff and Lincke2018). Coastal landforms evolve over time in response to a combination of oceanic, geologic, biologic and fluvial processes, and the relative importance of these processes can vary considerably along the world’s coasts and over time (Inman and Nordstrom, Reference Inman and Nordstrom1971; Wright and Short, Reference Wright and Short1984; Komar, Reference Komar1998; Friedrichs and Perry, Reference Friedrichs and Perry2001; Murray et al., Reference Murray, Knaapen, Tal and Kirwan2008; Winterwerp et al., Reference Winterwerp, Erftemeijer, Suryadiputra, van Eijk and Zhang2013; Splinter et al., Reference Splinter, Turner, Reinhardt and Ruessink2017; Wright et al., Reference Wright, Syvitski, Nichols, Zinnert, Wright and Nichols2019). Primary factors in the evolution of many coastal landforms are the volume of sediment available to these systems and the physical processes that move sediment over time (Syvitski, Reference Syvitski2005; Frihy et al., Reference Frihy, Shereet and El Banna2008; Anthony et al., Reference Anthony, Marriner and Morhange2014; Nienhuis et al., Reference Nienhuis, Ashton, Nardin, Fagherazzi and Giosan2016; Yang et al., Reference Yang, Yang, Xu, Wu, Shi and Zhu2017; Tessler et al., Reference Tessler, Vörösmarty, Overeem and Syvitski2018; Besset et al., Reference Besset, Anthony and Bouchette2019; Warrick et al., Reference Warrick, Stevens, Miller, Harrison, Ritchie and Gelfenbaum2019).
Coastal landforms can be characterised by the amounts and types of sediment available to them, and there are two endmembers with respect to terrestrial sediment supply: (1) coastal landforms that receive no direct terrestrial sediment, such as many atolls and barrier islands (Heron et al., Reference Heron, Moslow, Berelson, Herbert, Steele, Susman, Greenwood and Davis1984; FitzGerald et al., Reference FitzGerald, Fenster, Argow and Buynevich2008; Duvat, Reference Duvat2019), and (2) landforms that are derived almost entirely from inputs of terrestrial sediment, such as deltas of the world’s rivers and littoral cells along many of the world’s active continental margins (Inman and Nordstrom, Reference Inman and Nordstrom1971; Hicks and Inman, Reference Hicks and Inman1987; Ashton and Giosan, Reference Ashton and Giosan2011; Nienhuis et al., Reference Nienhuis, Ashton, Roos, Hulscher and Giosan2013; Giosan et al., Reference Giosan, Syvitski, Constantinescu and Day2014; Anthony, Reference Anthony2015). For coastal systems that are derived from terrestrial sediment, changes in the volume or grain size of sediment delivered to the coast can result in pronounced changes in the morphology or morphodynamical trajectory (Cooper, Reference Cooper2001; Nienhuis et al., Reference Nienhuis, Ashton, Roos, Hulscher and Giosan2013, Reference Nienhuis, Ashton, Edmonds, Hoitink, Kettner and Rowland2020; Anthony et al., Reference Anthony, Marriner and Morhange2014; Giosan et al., Reference Giosan, Syvitski, Constantinescu and Day2014; Bendixen et al., Reference Bendixen, Iversen, Bjørk, Elberling, Westergaard-Nielsen, Overeem, Barnhart, Khan, Box, Abermann, Langley and Kroon2017; Luo et al., Reference Luo, Yang, Wang, Zhang and Li2017; Warrick et al., Reference Warrick, Stevens, Miller, Harrison, Ritchie and Gelfenbaum2019; Hoitink et al., Reference Hoitink, Nittrouer, Passalacqua, Shaw, Langendoen and Huismans2020; Yang et al., Reference Yang, Luo, Temmerman, Kirwan, Bouma and Xu2020; Syvitski et al., Reference Syvitski, Ángel, Saito, Overeem, Vörösmarty and Wang2022). Thus, to evaluate and predict coastal changes for systems influenced by terrestrial sediment, it is essential to understand several characteristics of the sediment supply, including the processes that deliver sediment to the coast, the timing, volume and characteristics, including grain size distributions, of these sediment contributions and the littoral processes that disperse sediment from the river mouth (Hicks and Inman, Reference Hicks and Inman1987; Orton and Reading, Reference Orton and Reading1993; Kao and Milliman, Reference Kao and Milliman2008; Romans et al., Reference Romans, Normark, McGann, Covault and Graham2009; Milliman and Farnsworth, Reference Milliman and Farnsworth2013; Nienhuis et al., Reference Nienhuis, Ashton, Roos, Hulscher and Giosan2013; Anthony, Reference Anthony2015; Warrick, Reference Warrick2020).
The goal of this paper is to examine and summarise the temporal variability in terrestrial sediment supply to the coast over the decadal to century timescales important to coastal land management. We focus on littoral systems that are derived from terrestrial sources of sediment, which are generally found along active continental margins of the world and have small, steep coastal watersheds that are collectively the dominant supply of sediment to the world’s coasts (Inman and Nordstrom, Reference Inman and Nordstrom1971; Milliman and Syvitski, Reference Milliman and Syvitski1992; Milliman and Farnsworth, Reference Milliman and Farnsworth2013). Because of the efficient transfer of sediment through these small, steep watersheds (Milliman and Farnsworth, Reference Milliman and Farnsworth2013; Romans et al., Reference Romans, Castelltort, Covault, Fildani and Walsh2016), we will highlight how coastal supplies of sediment are influenced by natural hazards, infrequent events and ongoing and pending climate change. We acknowledge that the sediment budgets of many coastal systems, such as barrier islands and atolls, are not influenced by these watershed processes, owing to negligible sediment contributions from terrestrial landscapes to these coastal landforms (Meade, Reference Meade1982; Woodroffe et al., Reference Woodroffe, Samosorn, Hua and Hart2007; Perry et al., Reference Perry, Kench, O’Leary, Morgan and Januchowski-Hartley2015). We also acknowledge that terrestrial supplies of sediment may not be fully integrated into littoral cells, because of offshore transport to the deep sea either during the river discharge event or in the years, decades or centuries following these events (Mulder et al., Reference Mulder, Syvitski, Migeon, Faugères and Savoye2003; Khripounoff et al., Reference Khripounoff, Vangriesheim, Crassous and Etoubleau2009; Casalbore et al., Reference Casalbore, Chiocci, Scarascia Mugnozza, Tommasi and Sposato2011; Liu et al., Reference Liu, Hsu, Hung, Chang, Wang and Rendle-Bühring2016; Romans et al., Reference Romans, Castelltort, Covault, Fildani and Walsh2016; Steel et al., Reference Steel, Simms, Warrick and Yokoyama2016; Warrick, Reference Warrick2020). As such, we are reminded that every river and coastal setting is unique, and that care should be taken to understand sediment sources, dispersal processes and timescales, and sinks for each coastal system. In the synthesis below, we summarise characteristic shoreline changes caused by time-varying river sediment discharge and provide considerations for future research and monitoring in the light of ongoing climate change and sea-level rise.
River sediment discharge to the coast
There are abundant examples of fluvial sediment discharge events influencing coastal sediment budgets and morphodynamics. One of these examples is observed at the Rio Rimac of Peru (Figure 1), which discharges exceptional amounts of sediment to the coast during years of high precipitation (French and Mechler, Reference French and Mechler2017; Guzman et al., Reference Guzman, Ramos and Dastgheib2020). During these wet years, the shoreline at the river mouth progrades hundreds of metres seaward, resulting in a river mouth delta with several distributary channels (Figure 1a–d). Following progradation, the delta recedes because of decreases in river sediment discharge and northward littoral sediment transport from wave action (Figure 1e–j). The littoral transport causes beach widening at least 7 km downdrift of the Rio Rimac mouth (Guzman et al., Reference Guzman, Ramos and Dastgheib2020). Similar patterns of coastal progradation followed by recession and sediment spreading are observed at river mouths around the world as they respond to time-varying sediment inputs (Inman and Nordstrom, Reference Inman and Nordstrom1971; Hicks and Inman, Reference Hicks and Inman1987; Cooper, Reference Cooper1993; Anthony and Blivi, Reference Anthony and Blivi1999; Barnard and Warrick, Reference Barnard and Warrick2010; Casalbore et al., Reference Casalbore, Chiocci, Scarascia Mugnozza, Tommasi and Sposato2011; Giosan et al., Reference Giosan, Coolen, Kaplan, Constantinescu, Filip and Filipova-Marinova2012; Milliman and Farnsworth, Reference Milliman and Farnsworth2013; Anthony et al., Reference Anthony, Marriner and Morhange2014; Bendixen et al., Reference Bendixen, Iversen, Bjørk, Elberling, Westergaard-Nielsen, Overeem, Barnhart, Khan, Box, Abermann, Langley and Kroon2017; Besset et al., Reference Besset, Anthony and Sabatier2017; Luo et al., Reference Luo, Yang, Wang, Zhang and Li2017; East et al., Reference East, Stevens, Ritchie, Barnard, Campbell-Swarzenski and Collins2018; Warrick et al., Reference Warrick, Stevens, Miller, Harrison, Ritchie and Gelfenbaum2019). Satellite imagery can provide important observations of the spatial and temporal response of shorelines to new contributions of river sediment (Besset et al., Reference Besset, Anthony, Brunier and Dussouillez2016, Reference Besset, Anthony and Bouchette2019; Guzman et al., Reference Guzman, Ramos and Dastgheib2020; Warrick et al., Reference Warrick, Vos, East and Vitousek2022), and example satellite records of the multitude of coastal systems with active river sediment supplies are provided in Figure 2.

Figure 1. The influence of river sediment discharge on the coastal morphology and shoreline positions at the mouth of Rio Rímac, Peru, from 2016 to 2021. As described by Guzman et al. (Reference Guzman, Ramos and Dastgheib2020), heavy flooding in early 2017 resulted in massive growth of the river mouth delta and spreading of this sediment northward in the subsequent years, similar to the coastal morphodynamics following flooding in 1983 and 1998. Imagery from Google Earth.
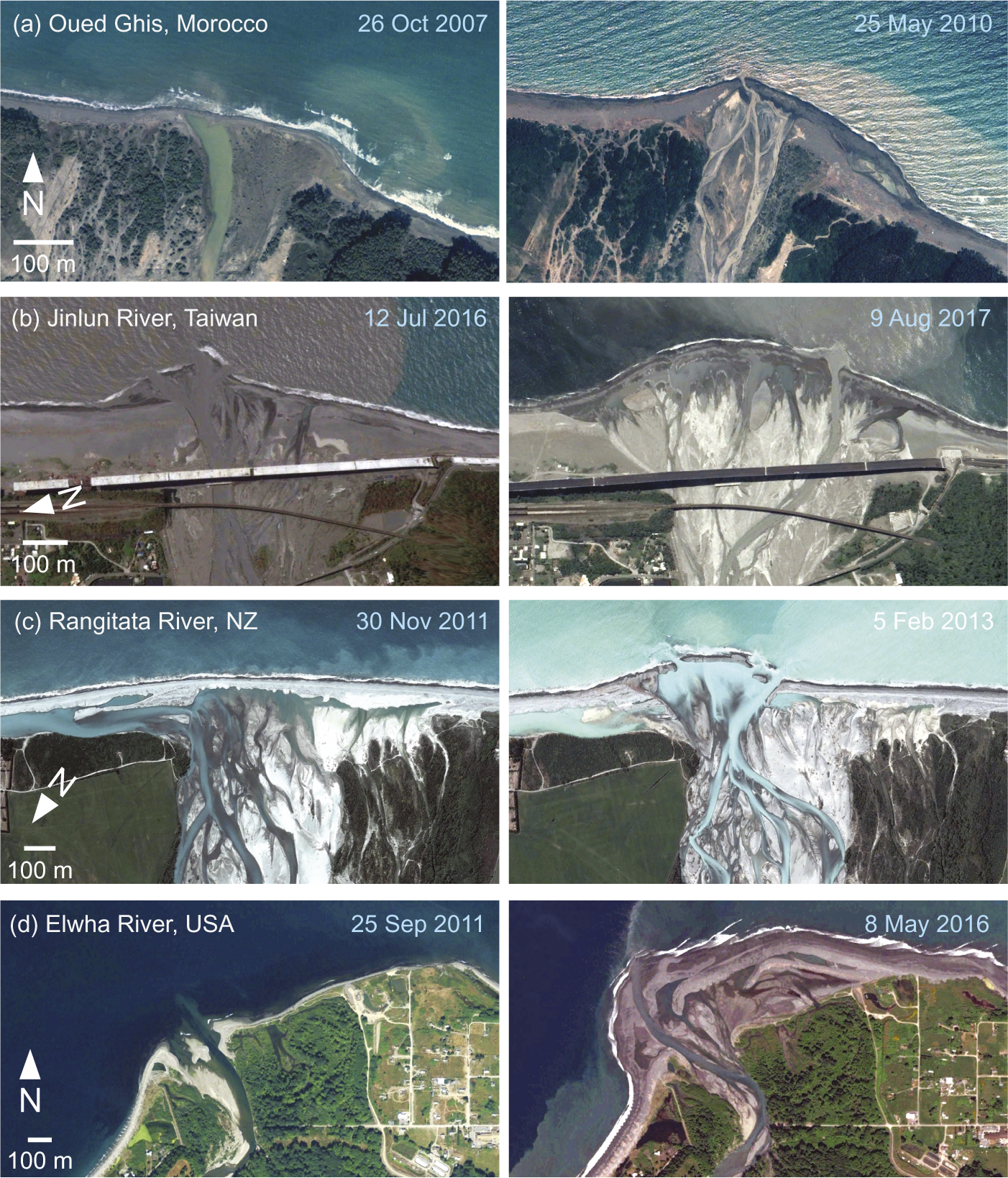
Figure 2. Examples of coastal changes at the mouths of small rivers of the world resulting from contributions of new sediment. Imagery from Google Earth.
Unusually large sediment discharge events can produce shoreline changes that persist for decades or centuries, as evidenced by coastal accretion and geomorphic changes caused by upland volcanic activity on sediment transport from the Santo Tomas River of the Philippines and the Rio Salamá of Guatemala (Figure 3). Coastal accretion at the mouths of both systems extended hundreds of metres to several kilometres offshore of the pre-eruption shorelines, and sediment inputs influenced shoreline positions for several to tens of kilometres along the coast (Kuenzi et al., Reference Kuenzi, Horst and McGehee1979; Siringan and Ringor, Reference Siringan and Ringor2007). The shoreline accretion from the eruption of Santa Maria, Guatemala, continues to extend more than 1 km offshore of the pre-eruption shoreline even though the volcanic event occurred over 120 years ago, providing evidence for the longevity of coastal impacts from rare, but massive, sediment discharge events (Kuenzi et al., Reference Kuenzi, Horst and McGehee1979; Figure 3b).

Figure 3. Decadal to century persistence of coastal accretion from increases in river sediment yield resulting from volcanic activity in coastal watersheds. (a) The mouth of the Santo Tomas River 28 years after the eruption of Mount Pinatubo, Philippines. (b) The mouth of Rio Salamá almost 120 years after the eruption of Santa Maria, Guatemala. Additional shorelines from before and immediately following the eruptions from publicly available Landsat imagery or interpretations of Kuenzi et al. (Reference Kuenzi, Horst and McGehee1979). Imagery from Google Earth.
Detailed accounting of shoreline responses to river inputs can be obtained where physical monitoring or remote sensing records are adequately frequent (Hicks and Inman, Reference Hicks and Inman1987; Barnard and Warrick, Reference Barnard and Warrick2010; Besset et al., Reference Besset, Anthony, Brunier and Dussouillez2016, Reference Besset, Anthony and Bouchette2019; Vos et al., Reference Vos, Harley, Splinter, Simmons and Turner2019a; Guzman et al., Reference Guzman, Ramos and Dastgheib2020; Warrick et al., Reference Warrick, Vos, East and Vitousek2022). For example, the combination of satellite-derived shoreline positions from Landsat and Sentinel-2 imagery using the CoastSat technique (Vos et al., Reference Vos, Splinter, Harley, Simmons and Turner2019b) and estimates of littoral-grade sediment discharge (Barnard and Warrick, Reference Barnard and Warrick2010) for the Santa Clara River, California, shows how the shoreline responds differently in space and time to elevated river sediment discharge (Figure 4). Sand discharge by the Santa Clara River is punctuated by several wet years, including 1983, 1993, 1995, 1998 and 2005, which combined contributed almost 20 Mt, or approximately 13 million cubic metres, of littoral-grade sand to the coast (Figure 4a,b). The shoreline at the Santa Clara River mouth accreted rapidly during these wet years, followed by multiple-year to decadal-scale shoreline recession towards previous positions (Figure 4c,d). In contrast, shorelines greater than 1,000 m downcoast from the river mouth had progressively lagged and muted accretion responses (Figure 4e–g). Overall, the beach 2,000 m downcoast of the river mouth accreted approximately 60 m between 1990 and 2020 as a result of sediment spreading from the combined river sediment discharge events of the 1990s and 2005 (Figure 4e,f; Barnard and Warrick, Reference Barnard and Warrick2010). Combined, these river mouth systems show that sediment input signals can vary greatly in time and that these signals may propagate across and along the shoreline, as described in more detail by coastal observations and theory (Komar, Reference Komar1973; Hicks and Inman, Reference Hicks and Inman1987; Inman et al., Reference Inman, Jenkins, McLachlan, Orme, Leatherman, Whitman and Schwartz2005; Casalbore et al., Reference Casalbore, Chiocci, Scarascia Mugnozza, Tommasi and Sposato2011; Anthony, Reference Anthony2015; East et al., Reference East, Stevens, Ritchie, Barnard, Campbell-Swarzenski and Collins2018; Besset et al., Reference Besset, Anthony and Bouchette2019; Warrick, Reference Warrick2020).

Figure 4. River sediment discharge and shoreline positions of the Santa Clara River, California, highlighting the effects of infrequent events on shoreline accretion and the spatial and temporal variations of shoreline response to new sediment. (a) Annual rainfall at a National Weather Service station near the river. (b) Littoral-grade sand (>125 μm) discharge from the Santa Clara River after Barnard and Warrick (Reference Barnard and Warrick2010); data from 2009 to 2021 were not estimated due to a lack of river gauging. (c–g) Shoreline positions from five transects derived from CoastSat analyses of Vos et al. (Reference Vos, Splinter, Harley, Simmons and Turner2019b). Shoreline positions are normalised to the average position of each transect from 1990 to 1992 when the shoreline was consistently narrow. (h) Satellite imagery of the Santa Clara River mouth following the 2005 sediment discharge events from Google Earth. Locations of the shoreline from a September 2004 image and the CoastSat transects are shown.
Temporal variability in river sediment discharge
Infrequent river sediment discharge events that dramatically alter coastal morphology and shoreline positions – such as the floods on the Rio Rímac (Figure 1) and the Santa Clara River (Figure 4), volcanic-related sediment discharge shown for the Santo Tomas River and Rio Salamá (Figure 3) or profound accretion from storm-induced debris-flow activity, as Casalbore et al. (Reference Casalbore, Chiocci, Scarascia Mugnozza, Tommasi and Sposato2011) documented on the coast of Sicily – provide important examples of how temporal variations in terrestrial sediment supply can strongly influence littoral systems over spatial scales of kilometres to tens of kilometres and temporal scales of years to over a century. As such, we will explore a few examples of the temporal variability of river sediment discharge, and especially the role of infrequent large discharge events, in the export of sediment from the land to the sea.
To assist with this exploration, we have included a series of sediment discharge records from watersheds ranging from a small, steep river draining the rugged Big Sur coast of California to the world’s largest river, the Amazon (Figure 5). Sediment discharge records from these rivers reveal that the smaller watersheds are generally more punctuated by infrequent high-discharge events, whereas the massive Amazon River has relatively constant sediment discharge from year to year. Additionally, the records also highlight the effects of perturbations, such as wildfires, floods, earthquakes, typhoons and human impacts, on year-to-year variations in sediment discharge (Figure 5), which are described more fully in original investigations of these and other rivers (Dadson et al., Reference Dadson, Hovius, Chen, Dade, Lin and Hsu2004; Gran and Montgomery, Reference Gran and Montgomery2005; Wang et al., Reference Wang, Yang, Saito, Liu, Sun and Wang2007; Hovius et al., Reference Hovius, Meunier, Lin, Chen, Chen and Dadson2011; Lee et al., Reference Lee, Huang, Lee, Jien, Zehetner and Kao2015; Gray, Reference Gray2018; Montanher et al., Reference Montanher, de Morais Novo and de Souza Filho2018; Collins et al., Reference Collins, Oakley, Perkins, East, Corbett and Hatchett2020; Warrick et al., Reference Warrick, Vos, East and Vitousek2022). Sediment discharge is especially elevated when a landscape perturbing event, such as wildfire or an earthquake, is followed by heavy precipitation, such as shown in the records from the Big Sur River and Choshoi River watersheds (Figure 5a,b; Dadson et al., Reference Dadson, Hovius, Chen, Dade, Lin and Hsu2004; Hovius et al., Reference Hovius, Meunier, Lin, Chen, Chen and Dadson2011; Warrick et al., Reference Warrick, Vos, East and Vitousek2022). Over larger watershed scales of hundreds of thousands to millions of square kilometres, events such as earthquakes or wildfires impact relatively small areas compared to the river’s total drainage basin area and thus contribute only marginally to the overall temporal variations in sediment fluxes (Milliman and Farnsworth, Reference Milliman and Farnsworth2013; Francis et al., Reference Francis, Fan, Hales, Hobley, Xu and Huang2022). Thus, temporal variability in sediment discharge from the large rivers of the world is often attributed to factors that influence broader areas of these watersheds, such as widespread land use change from agriculture development, dams on the mainstem river or climate patterns influencing the hydrology of the broader basin (Walling, Reference Walling2006; Wang et al., Reference Wang, Yang, Saito, Liu, Sun and Wang2007; Zheng et al., Reference Zheng, Xu, Cheng, Wang, Xu and Wu2018; Syvitski et al., Reference Syvitski, Ángel, Saito, Overeem, Vörösmarty and Wang2022; Figure 5c).

Figure 5. Annual sediment discharge measurements for four different rivers highlighting how temporal variations are influenced by perturbations such as wildfires, floods and earthquakes and the size of the watershed. Time series shown in (a)–(d) have been transformed into ranked annual exceedance values in (e) using the cumulative sediment discharge measured in each river. Recurrence intervals were estimated by the reciprocal of the annual exceedance probabilities. Data for (a)–(d) were derived from Warrick et al. (Reference Warrick, Vos, East and Vitousek2022), Lee et al. (Reference Lee, Huang, Lee, Jien, Zehetner and Kao2015), Wang et al. (Reference Wang, Yang, Saito, Liu, Sun and Wang2007), and Montanher et al. (Reference Montanher, de Morais Novo and de Souza Filho2018), respectively. Descriptive terms about the watershed sizes (right-hand side) are derived from discussion in Romans et al. (Reference Romans, Castelltort, Covault, Fildani and Walsh2016).
For the four rivers used in our example, the cumulative sediment discharge of the smaller rivers is more heavily dictated by infrequent events than the larger rivers. This is shown by the steepness of the cumulative sediment discharge curves in Figure 5e, which provides contrast between small rivers such as the Big Sur River of California, for which sediment discharge during the two biggest years represented roughly two-thirds the 50-yr sediment discharge to the coast, and the Amazon River, for which the sediment discharged every year is relatively constant. The high temporal variability in the Big Sur River is largely related to the combined effects of two wildfire and heavy precipitation events (labelled ‘Fire + Flood’; Figure 5a; Warrick et al., Reference Warrick, Vos, East and Vitousek2022), although sediment discharge from this river is still strongly variable in time if these events are not considered in the records (Figure 5e). The inverse relationship between watershed size and temporal variability in river sediment discharge shown in Figure 5 is consistent with broader understanding of the erosion and sediment transport for rivers throughout the world (Hicks et al., Reference Hicks, Gomez and Trustrum2000; Dadson et al., Reference Dadson, Hovius, Chen, Dade, Lin and Hsu2004; Kao and Milliman, Reference Kao and Milliman2008; Gonzalez-Hidalgo et al., Reference Gonzalez-Hidalgo, Batalla, Cerdà and de Luis2010; Milliman and Farnsworth, Reference Milliman and Farnsworth2013; Gray, Reference Gray2018). As such, small, steep watersheds may be considered ‘reactive’ with respect to perturbing effects on sediment discharge, whereas large, continental-scale watersheds may be considered more ‘buffered’ against these effects (cf. Romans et al., Reference Romans, Castelltort, Covault, Fildani and Walsh2016).
The accounting of sediment discharge to the coast – such as shown in Figure 5 – is generally derived from sampling of river sediment fluxes and applications of models derived from these data (Walling and Fang, Reference Walling and Fang2003; Milliman and Farnsworth, Reference Milliman and Farnsworth2013; Syvitski et al., Reference Syvitski, Ángel, Saito, Overeem, Vörösmarty and Wang2022). Unfortunately, the length of river sampling records is generally limited to intervals of years to several decades (Milliman and Farnsworth, Reference Milliman and Farnsworth2013; Warrick and Milliman, Reference Warrick and Milliman2018). Although monitoring records are essential for identifying rates and trends in river sediment transport (Gray, Reference Gray2018), the largest historical events may not be captured by limited duration of sediment sampling. In fact, the exclusion of the largest sediment discharge events is a primary factor for why river sampling records may underestimate long-term watershed sediment yields (Kirchner et al., Reference Kirchner, Finkel, Riebe, Granger, Clayton and King2001; Covault et al., Reference Covault, Craddock, Romans, Fildani and Gosai2013). Combined, this suggests that long-term sediment discharge to the coast – especially from the globally important small, steep rivers – is primarily related to the magnitude and frequency of rare large events.
Climate change
Climate change is modifying the event frequency and intensity of several watershed sediment yield factors discussed above, including the amount and intensity of precipitation and the size, frequency and intensity of wildfires (Westerling et al., Reference Westerling, Hidalgo, Cayan and Swetnam2006; Pachauri et al., Reference Pachauri and Mayer2015; Sankey et al., Reference Sankey, Kreitler, Hawbaker, McVay, Miller and Mueller2017; Swain et al., Reference Swain, Langenbrunner, Neelin and Hall2018; Ball et al., Reference Ball, Regier, González-Pinzón, Reale and Van Horn2021; Touma et al., Reference Touma, Stevenson, Swain, Singh, Kalashnikov and Huang2022). Additionally, rising temperatures are changing the hydrology and sediment yields of both Arctic and alpine landscapes (Bendixen et al., Reference Bendixen, Iversen, Bjørk, Elberling, Westergaard-Nielsen, Overeem, Barnhart, Khan, Box, Abermann, Langley and Kroon2017; Li et al., Reference Li, Lu, Overeem, Walling, Syvitski and Kettner2021a; Irrgang et al., Reference Irrgang, Bendixen, Farquharson, Baranskaya, Erikson and Gibbs2022; Vergara et al., Reference Vergara, Garreaud and Ayala2022). As such, there is a growing understanding that climate change is causing fundamental changes to the rate of sediment delivery from many landscapes to fluvial and coastal landforms. These effects are most clearly evident in Arctic settings, where terrestrial sediment inputs have increased at the same time that ice-free conditions in the adjacent seas are getting longer (Bendixen et al., Reference Bendixen, Iversen, Bjørk, Elberling, Westergaard-Nielsen, Overeem, Barnhart, Khan, Box, Abermann, Langley and Kroon2017; Irrgang et al., Reference Irrgang, Bendixen, Farquharson, Baranskaya, Erikson and Gibbs2022). Combined, this is resulting in the expansion of some Artic deltas – such as those along the Greenland coast – and accelerated erosion on many wave-exposed Arctic shorelines (Bendixen et al., Reference Bendixen, Iversen, Bjørk, Elberling, Westergaard-Nielsen, Overeem, Barnhart, Khan, Box, Abermann, Langley and Kroon2017; Irrgang et al., Reference Irrgang, Bendixen, Farquharson, Baranskaya, Erikson and Gibbs2022). There is also growing evidence for changes in fluvial sediment discharge in lower latitudes, as wildfire and precipitation are actively changing with climate (Lee et al., Reference Lee, Huang, Lee, Jien, Zehetner and Kao2015; East and Sankey, Reference East and Sankey2020; Touma et al., Reference Touma, Stevenson, Swain, Singh, Kalashnikov and Huang2022). Climate-induced changes are expected to continue with time, and they may dramatically alter sediment budgets of rivers and their downstream coasts.
Conceptual model and future directions
As highlighted above, infrequent fluvial sediment discharge events are a driving factor for many coastal littoral systems worldwide. This time-dependent variability commonly results in wet conditions delivering considerably more sediment than dry conditions do, and when these wet conditions are combined with increases in hillslope sediment supplies from wildfires, earthquakes or volcanic activity, sediment discharge can be exceptional. That is, infrequent events are generally responsible for the majority of sediment transport to the coast (Milliman and Farnsworth, Reference Milliman and Farnsworth2013).
We have integrated these concepts into a conceptual model of hypothetical watershed sediment yields and shoreline positions for a small, steep watershed that efficiently conveys sediment from source regions to the coast (cf. Romans et al., Reference Romans, Castelltort, Covault, Fildani and Walsh2016) that will be used to discuss historic and future trajectories of coastlines (Figure 6). In this simple model, there are several perturbations to watershed sediment yield: precipitation, which causes landscape erosion and downstream flooding; wildfire, which denudes the landscape and increases the potential for soil erosion; earthquakes, which liberate regolith throughout the watershed and volcanic activity, which introduces new sediment materials and disrupts the watershed landscape (Figure 6). As highlighted above, the effects of wildfires on watershed sediment yield are enhanced when they are followed closely by heavy precipitation, as denoted by three ‘fire + flood’ events in the hypothetical records (Figure 6). Additionally, the conceptual model includes characteristic human impacts to sediment yields, including increases from land use such as agriculture and road building and decreases from the construction of dams (Kosmas et al., Reference Kosmas, Danalatos, Cammeraat, Chabart, Diamantopoulos and Farand1997; Vörösmarty et al., Reference Vörösmarty, Meybeck, Fekete, Sharma, Green and Syvitski2003; Syvitski, Reference Syvitski2005; Walling, Reference Walling2006; Anthony et al., Reference Anthony, Marriner and Morhange2014; Luo et al., Reference Luo, Yang, Wang, Zhang and Li2017; Li et al., Reference Li, Li, Zhu, Meadows, Zhu and Zhang2021b; Syvitski et al., Reference Syvitski, Ángel, Saito, Overeem, Vörösmarty and Wang2022).

Figure 6. Conceptual model of coastal responses to watershed processes for a theoretical small, steep river basin (after the fire-flood model of Keller et al., Reference Keller, Valentine and Gibbs1997). Relative sediment yield of the watershed is influenced by stochastic events, including floods, wildfires, earthquakes, volcanic activity and combined events, such as wildfire followed by flooding (‘F + F’). The shoreline position of the littoral cell responds to increases in watershed sediment yield with accretion events (upward pointing arrows) because of the efficient transfer of river sediment to the littoral cell. Future shoreline positions (right-hand side) will be determined by balance between sediment supply and sea-level rise. Moreover, highlighted are hypothetical intervals of river sampling, climate change effects, land-use-change effects and damming of the river.
The time-varying rate of sediment yield, driven in large part by infrequent events and human impacts, has direct effects on the shoreline position of the littoral cell near the river mouth (Figure 6). For our hypothetical system, small increases in sediment yield result in short-lived changes in the shoreline position, much like the coastal response to monitored events in the Santa Clara River (cf. Figure 4). The five large sediment yield events (highlighted with grey shading; Figure 6) cause fundamental changes in the shoreline position as shown by accretion events (arrows; Figure 6). These large events are conceptually similar to the volcanic events highlighted above (cf. Figure 3). Lastly, human impacts may result in coastal accretion or erosion trends, depending on the nature and scale of these impacts (Figure 6).
A few additional items are emphasised in the conceptual model. First, although the river sampling records (blue shading; Figure 6) are shown to capture several decades of sediment discharge including an era of human impacts, they do not include some of the largest and most significant events of the past two centuries. This is rather common for actual river records given that they are commonly years to decades in length (Warrick and Milliman, Reference Warrick and Milliman2018). To properly understand century-scale or longer sediment yields, river sampling records should be integrated with either an understanding of the role, magnitude and frequency of events that are not included in the sampling record, or with broader geologic understanding of the discharge record from measurements such as sediment cores or cosmogenic nuclides (Walling, Reference Walling1988; Lamoureux, Reference Lamoureux2000; Kirchner et al., Reference Kirchner, Finkel, Riebe, Granger, Clayton and King2001; von Blanckenburg, Reference Von Blanckenburg2005; Covault et al., Reference Covault, Craddock, Romans, Fildani and Gosai2013). Additionally, the lack of exceptional events in most sampling records highlights the importance of records that do include these unique events (e.g., Kuenzi et al., Reference Kuenzi, Horst and McGehee1979; Gran and Montgomery, Reference Gran and Montgomery2005; Korup, Reference Korup2012; Ritchie et al., Reference Ritchie, Warrick, East, Magirl, Stevens and Bountry2018; Fan et al., Reference Fan, Scaringi, Korup, West, Westen and Tanyas2019; Warrick et al., Reference Warrick, Vos, East and Vitousek2022), largely because they allow for the new understanding to be transferred to longer timescales and to other river systems. Second, in looking towards a future with continued climate change (yellow shading; Figure 6), the sustainability of coastal landforms such as the littoral systems highlighted here will depend on whether sediment contributions to the coast can balance the erosion caused by sea-level rise and associated impacts (FitzGerald et al., Reference FitzGerald, Fenster, Argow and Buynevich2008; Syvitski et al., Reference Syvitski, Kettner, Overeem, Hutton, Hannon and Brakenridge2009; Giosan et al., Reference Giosan, Syvitski, Constantinescu and Day2014; Nienhuis et al., Reference Nienhuis, (Ton) Hoitink and Törnqvist2018; Reimann et al., Reference Reimann, Vafeidis, Brown, Hinkel and Tol2018; Schuerch et al., Reference Schuerch, Spencer, Temmerman, Kirwan, Wolff and Lincke2018; Hoitink et al., Reference Hoitink, Nittrouer, Passalacqua, Shaw, Langendoen and Huismans2020). That is, the future of the world’s shorelines will be determined not only by global eustatic sea-level changes, but also by many local factors such as the rate and variability of sediment supply and the conditions and processes that are responsible for transporting sediment throughout the coastal zone (Casalbore et al., Reference Casalbore, Chiocci, Scarascia Mugnozza, Tommasi and Sposato2011; Anthony, Reference Anthony2015; Nienhuis et al., Reference Nienhuis, Ashton, Nardin, Fagherazzi and Giosan2016; Steel et al., Reference Steel, Simms, Warrick and Yokoyama2016; Caldwell et al., Reference Caldwell, Edmonds, Baumgardner, Paola, Roy and Nienhuis2019; Warrick, Reference Warrick2020).
Summarising, the future of many coasts will be tied to terrestrial sediment inputs (Anthony and Blivi, Reference Anthony and Blivi1999; Syvitski et al., Reference Syvitski, Kettner, Overeem, Hutton, Hannon and Brakenridge2009; Giosan et al., Reference Giosan, Coolen, Kaplan, Constantinescu, Filip and Filipova-Marinova2012, Reference Giosan, Syvitski, Constantinescu and Day2014; Anthony et al., Reference Anthony, Marriner and Morhange2014), so a better understanding of the magnitude, frequency and implications of sediment supply rates is needed. This is especially true for infrequent events, which as noted above can dominate long-term coastal sediment budgets and can redefine coastal morphology. As coastal communities have multiple management options to confront the challenges of climate change, including actively nourishing landforms with imported sediment (de Schipper et al., Reference de Schipper, de Vries, Ruessink, de Zeeuw, Rutten and van Gelder-Maas2016; Ludka et al., Reference Ludka, Guza and O’Reilly2018; Armstrong and Lazarus, Reference Armstrong and Lazarus2019), these will need to be balanced with an understanding of the inherent coastal processes and morphodynamics, including natural sediment supplies. In some cases, watershed sediment supplies may be adequate to produce relatively constant shoreline positions for decades or more; in other cases, sediment discharge may be inadequate to keep up with sea-level rise, and coastal erosion and land loss will ensue (Figure 6).
To build this understanding under the current and future conditions of climate change requires collaborative communication and research efforts across hydrologic, geomorphic and coastal research groups. We encourage the continued development and progress of cross-disciplinary studies of coastal landforms, especially with respect to the linkages between watershed processes and coastal morphodynamics. Although this requires integration across several discipline boundaries (hydrology, oceanography, geomorphology, ecology, meteorology and climate science), it is essential to build this cross-disciplinary understanding where terrestrial and coastal systems are integrally linked. Additionally, because this work will be highly relevant for coastal communities worldwide that are actively confronting the effects of climate change through land use and expenditure decisions, it is valuable to integrate stakeholder collaboration and the challenges that coastal managers face into research goals and methods (Lemos et al., Reference Lemos, Arnott, Ardoin, Baja, Bednarek and Dewulf2018; Ulibarri et al., Reference Ulibarri, Goodrich, Wagle, Brand, Matthew and Stein2020). Although the coming era will provide considerable uncertainty for coastal communities and their natural resources, it is crucial that coastal scientists continue to develop relevant information and understanding about our changing coasts.
Open peer review
To view the open peer review materials for this article, please visit http://doi.org/10.1017/cft.2022.1.
Data availability statement
The data that support the findings of this study are openly available from scientific reports and publications (from Kuenzi et al., Reference Kuenzi, Horst and McGehee1979; Wang et al., Reference Wang, Yang, Saito, Liu, Sun and Wang2007; Barnard and Warrick, Reference Barnard and Warrick2010; Lee et al., Reference Lee, Huang, Lee, Jien, Zehetner and Kao2015; Montanher et al., Reference Montanher, de Morais Novo and de Souza Filho2018; Warrick et al., Reference Warrick, Vos, East and Vitousek2022), the imagery database contained within Google Earth Pro at https://www.google.com/earth/versions/#earth-pro, and the CoastSat shoreline position database at http://coastsat.wrl.unsw.edu.au/.
Acknowledgements
We are thankful for the support of the U.S. Geological Survey (USGS) of this work, including the USGS Coastal and Marine Hazards and Resources Program and a USGS Mendenhall postdoctoral fellowship to H.D. Peter Swarzenski provided comments on an earlier version of the manuscript.
Author contributions
All authors provided contributions to the development, organisation and writing of this article. J.A.W. compiled data and imagery and drafted the figures.
Financial support
This work was supported by the U.S. Geological Survey’s (USGS) Coastal and Marine Hazards and Resources Program and the USGS’s Mendenhall Research Fellowship Program.
Competing interests
The authors declare no competing interests exist.
Comments
Dear Editors--
We are pleased to provide the invited review article attached for the new journal Coastal Futures. Thank you for this opportunity, and we look forward to the review process.
Sincerely,
--Jonathan Warrick