Book contents
- Frontmatter
- Contents
- Preface
- 1 Natural extremes
- 2 A basic analytical framework
- 3 Platforms to excite a response
- 4 Tools to monitor response
- 5 Metals
- 6 Brittle materials
- 7 Polymers
- 8 Energetic materials
- 9 Asteroid impact
- Appendix A Relevant topics from materials science
- Appendix B Glossary
- Appendix C Elastic moduli in solid mechanics
- Appendix D Shock relations and constants
- Bibliography
- Index
- References
Bibliography
Published online by Cambridge University Press: 05 May 2013
- Frontmatter
- Contents
- Preface
- 1 Natural extremes
- 2 A basic analytical framework
- 3 Platforms to excite a response
- 4 Tools to monitor response
- 5 Metals
- 6 Brittle materials
- 7 Polymers
- 8 Energetic materials
- 9 Asteroid impact
- Appendix A Relevant topics from materials science
- Appendix B Glossary
- Appendix C Elastic moduli in solid mechanics
- Appendix D Shock relations and constants
- Bibliography
- Index
- References
Summary
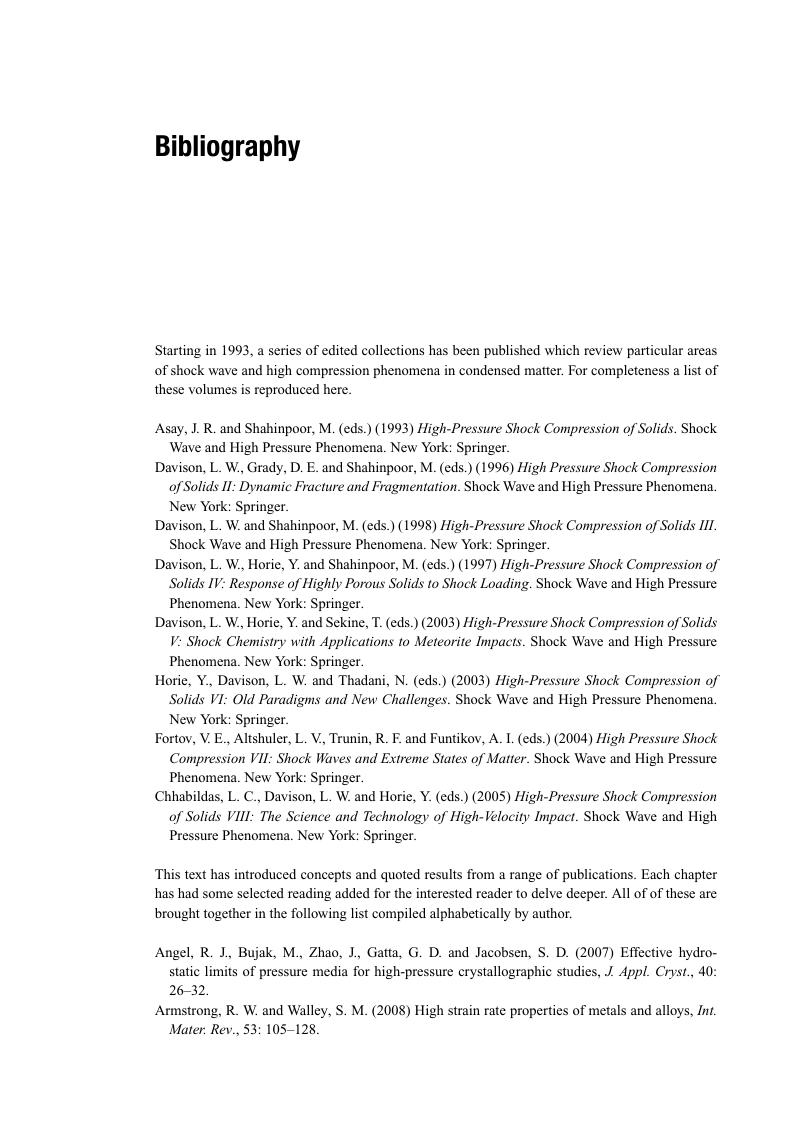
- Type
- Chapter
- Information
- Materials in Mechanical ExtremesFundamentals and Applications, pp. 515 - 523Publisher: Cambridge University PressPrint publication year: 2013