Book contents
- Light Scattering by Ice CrystalsFundamentals and Applications
- Light Scattering by Ice Crystals
- Copyright page
- Epigraph
- Contents
- Preface
- 1 Ice in the Earth's atmosphere
- 2 Fundamentals of light scattering by ice crystals
- 3 Principles of geometric optics for application to light scattering by ice crystals
- 4 Other useful approaches to light scattering by ice particles
- 5 Application of light scattering by ice crystals to remote sensing
- 6 Application of light scattering by ice crystals to climate studies
- References
- Index
- References
References
Published online by Cambridge University Press: 14 November 2016
- Light Scattering by Ice CrystalsFundamentals and Applications
- Light Scattering by Ice Crystals
- Copyright page
- Epigraph
- Contents
- Preface
- 1 Ice in the Earth's atmosphere
- 2 Fundamentals of light scattering by ice crystals
- 3 Principles of geometric optics for application to light scattering by ice crystals
- 4 Other useful approaches to light scattering by ice particles
- 5 Application of light scattering by ice crystals to remote sensing
- 6 Application of light scattering by ice crystals to climate studies
- References
- Index
- References
Summary
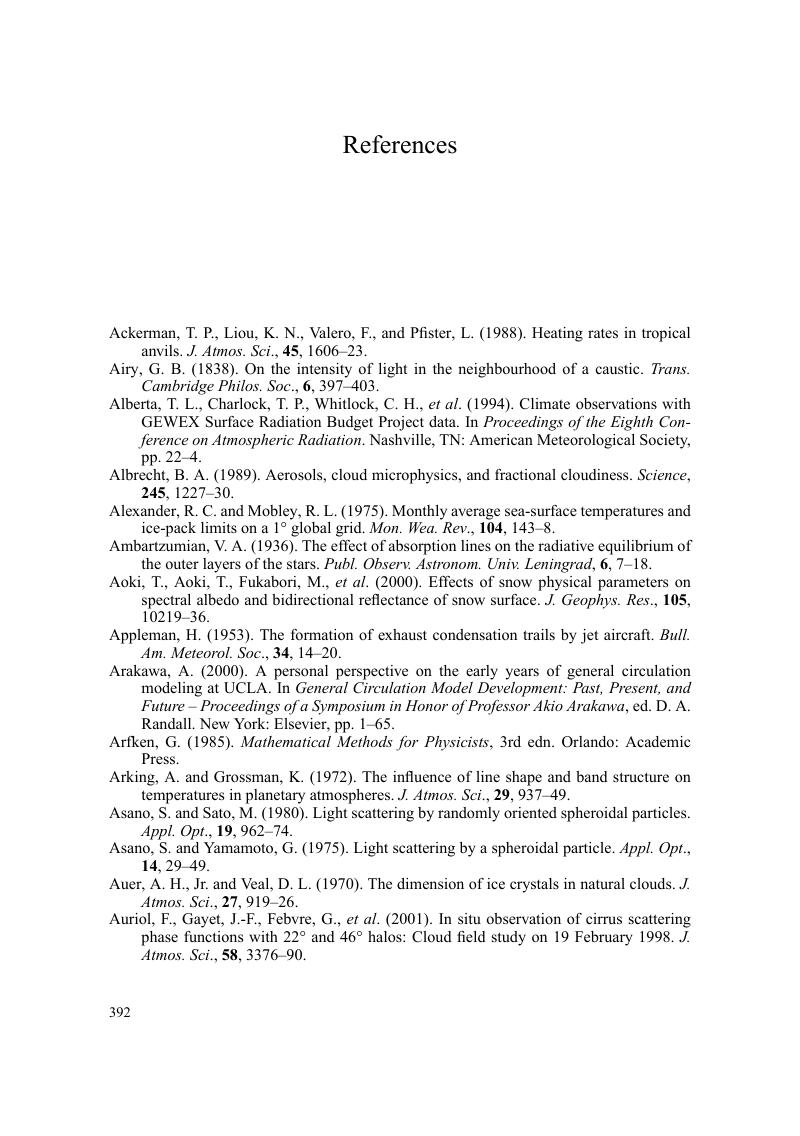
- Type
- Chapter
- Information
- Light Scattering by Ice CrystalsFundamentals and Applications, pp. 392 - 426Publisher: Cambridge University PressPrint publication year: 2016