Fluctuations in Zn metabolism throughout the reproductive cycle of sows are a result of physiological processes during gestation and lactation to support fetal growth and development and milk synthesis( Reference Mahan 1 – Reference Caulfield, Donangelo and Chen 4 ).
In particular, placental Zn transport, luminal Zn absorption and Zn mobilisation from the bone and liver are increased at the end of gestation and lactation( Reference Donangelo, Vargas Zapata and Woodhouse 2 – Reference Caulfield, Donangelo and Chen 4 ). This results in varying Zn transport and a changed role of transport proteins in both the fetus and the dam( Reference Perveen, Altaf and Vohra 5 ), thereby increasing the dietary Zn requirement.
Inadequate intake of dietary Zn during gestation and lactation may cause reproductive failures, without clinical signs of maternal Zn deficiency( Reference Smith and Akinbamijo 6 – Reference Vazquez-Armijo, Rojo and Salem 8 ): the sow depletes her body Zn reserves in tissues and fluids before litter size, fetal development or milk composition are affected( Reference Mahan 1 , Reference Smith and Akinbamijo 6 – Reference Vazquez-Armijo, Rojo and Salem 8 ). Scientific data of Zn requirements for sows are limited. Therefore, adequately measuring Zn status is critical, albeit difficult( Reference Apgar and Fritzgerald 9 – Reference Hambidge 11 ).
Zn status may be reflected by biochemical markers (i.e. biomarkers), which are related to the structural, regulatory and catalytic role of Zn. Plasma Zn is the most frequently used biomarker for Zn status. However, plasma Zn alone shows limitations if study conditions are not controlled or if confounding factors are present( Reference Lowe, Woodhouse and Sutherland 12 ). These confounding factors may be infection or diseases, an acute-phase response, stress, feeding state (fasted or postprandial measures), reproductive phase (gestation), and also the method of blood collection, and concomitant blood sample transport, storage and analysis( Reference Hambidge 11 , Reference Fairweather-Tait, Harvey and Ford 13 , Reference Lowe, Fekete and Decsi 14 ). Other biomarkers such as albumin, metallothionein (MT) and alkaline phosphatase (ALP) play a role in Zn metabolism and homeostasis: Zn is bound to albumin after absorption( Reference McDowell 15 , Reference Peters 16 ); MT (a small cysteine-rich protein) is involved in regulating the quantity of Zn absorbed( Reference McDowell 15 , Reference Cousins, Filer and Ziegler 17 , Reference Bikker and Jongbloed 18 ); ALP is a Zn-dependent enzyme in which Zn ions are present in the active centre of the enzyme( Reference Coleman 19 ). Together, they may be useful to assess the body's response to Zn supplementation or depletion. To our knowledge, the normal fluctuation of these biomarkers throughout the reproductive cycle of sows has not yet been determined.
The objective of the present study was to assess the Zn status of primiparous and multiparous sows using different Zn status biomarkers, to identify periods of critical Zn status throughout the reproductive cycle at different parities.
Materials and methods
Animals and diets
The experiment was performed on one group of twenty-three commercial hybrid sows (RA-SE Genetics) from the Institute for Agricultural and Fisheries Research (ILVO). However, one sow was too stressed during blood sampling and therefore excluded from the experiment. Furthermore, four sows were not gravid and three primiparous sows aborted at days 58, 66 and 93 of gestation, and therefore they were not included in the analysis. One primiparous sow developed an abscess under the cheek and required veterinary treatment. This sow remained in the experimental group, because her results were not considered outliers compared with other sows. Consequently, the experiment included ten multiparous and five primiparous sows from one group that were followed during one reproductive cycle. The multiparous sows (parity ranged between two and eleven) weighed 234 (sd 36) kg at weaning of the preceding lactation period (i.e. at the start of the study, day − 5). The primiparous sows (gilts, 233 (sd 12) d old at insemination) weighed 142 (sd 7) kg at day − 5.
The sows were all housed individually during the first 4 weeks of gestation and from 1 week before parturition until weaning. During mid- and end gestation, the sows were housed as one group using an automated feeding system with individual sow recognition through an electronic transponder in the sow's ear.
The sows were fed a gestation diet and a lactation diet according to commercial dietary standards and nutrient requirements( 20 ) (Tables 1 and 2). Feed samples of the gestation and lactation diet were subject to proximate analysis according to international standard methods accredited by ISO 17 025. The gestation diet was provided from the start of the study until 1 week before parturition, whereas the lactation diet was provided 1 week before parturition until weaning. During the first 4 weeks of gestation, the sows were fed twice daily, in total 2·3 kg. During mid- and end gestation, the sows were fed 2·6 kg/d. During lactation, 0·25 kg of feed per piglet was gradually supplemented in addition to 3 kg feed, provided in two equal portions daily (e.g. a sow with twelve piglets received 6 kg of feed daily). There were no feed leftovers. Throughout the experiment, all sows had ad libitum access to drinking-water, except in the first 4 weeks of gestation, in which water was automatically provided through nipple drinkers for 15 min every hour and for 45 min while feeding in order to prohibit water spillage.
Table 1 Ingredient composition of the gestation and lactation diets
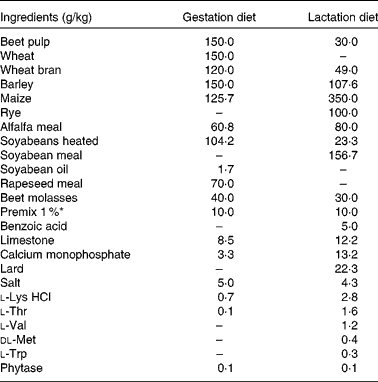
* Premix 1 % included per kg diet: vitamin A, 4·08 mg; vitamin D3, 0·05 mg; vitamin E, 75·0 mg; vitamin K3, 1·01 mg; vitamin B1, 1·5 mg; vitamin B2, 5·0 mg; vitamin B5, 18·0 mg; vitamin B6, 4·0 mg; vitamin B12, 0·03 mg; vitamin B3, 25·0 mg; vitamin B11, 3·0 mg; biotin, 0·3 mg; choline, 432·5 mg; Fe as FeSO4.H2O, 150·0 mg; Cu as CuSO4·5H2O, 16·43 mg; Mn as MnO, 50·0 mg; Zn as ZnO, 92·97 mg; I as Ca(IO3)2, 2·0 mg; Se as Na2O3Se, 0·40 mg; Ca, 0·89 mg; P, 0·05 mg; Mg, 0·16 mg; Na, 3·02 mg; Cl, 0·12 mg; K, 0·08 mg; S, 0·11 mg; lysine, 0·04 mg; methionine, 0·01 mg; threonine, 0·03 mg; tryptophan, 0·01 mg; butylhydroxytoluene, 0·50 mg; ethoxyquine, 0·55 mg; butylated hydroxyanisol, 0·05 mg.
Table 2 Analysed and calculated* nutrient composition of the gestation and lactation diets

ADF, acid-detergent fibre; NDF, neutral-detergent fibre; ADL, acid-detergent lignin; ID, ileal digestible; NEv, net energy for pigs.
* Chemical analyses of ID amino acids and NEv (NEv is calculated according to the feed tables of the Centraal Veevoederbureau (the Netherlands), 2007).
Experimental design
Blood samples were taken before feeding in the morning (between 08.00–10.00 hours), after overnight fasting (17·5 h), at fixed time intervals throughout one reproductive cycle: day − 5 (weaning of the preceding cycle for multiparous sows; ‘start of the study in December 2011’); throughout gestation (days 0 (insemination), 21, 42, 63 and 84); around parturition (days 108, 112, 115 (parturition) and 118); during lactation (days 122, 129 and 143 (weaning)). The days until parturition were predicted from the expected date of parturition (based on a gestation period of 115 d), whereas from parturition onwards, the sows were sampled based on the date of parturition. Blood samples were collected from the jugular vein using stainless-steel needles and plastic syringes, and added to one heparin and one serum vacuum tube (Terumo Europe). At parturition, two piglets per sow were randomly selected for blood sampling from the jugular vein before colostrum intake, and the blood sample was added to one heparin vacuum tube (Terumo Europe).
Within 1 h after the first piglet was born, colostrum was collected. At 1 week after parturition, milk samples were also collected using 1 ml oxytocin (17 μg oxytocin/ml; VMD N.V.) to stimulate milk release. The colostrum and milk samples were collected from all nipples from either the left or right side of the udder, repeatedly from front to back nipples, until 20 ml were collected. At weaning, reproductive performances were documented, taking into account cross-fostering and provision of creep feed (transitional feed) to the piglets from day 10 postpartum.
Body weight and back-fat thickness were measured at the start of the study (i.e. weaning of the preceding lactation period for the multiparous sows (day − 5)), at days 63, 84 and 108 of gestation, and at weaning (day 143). Back-fat thickness was measured between the 3rd and 4th last rib, 7 cm at the left and right side of the vertebrae (P2). After P2 was lubricated, back-fat measurements were repeated three times, alternately at the left and right side (PIGLOG 105; SFK Technology). The average thickness was used for further calculations.
The present observational study was conducted according to the institutional and national guidelines for the care and use of animals, and all experimental procedures involving these animals were approved by the ILVO's ethical committee for animal experiments.
Preparation of blood samples
A heparinised blood sample (1 ml) was used for haematocrit assay and centrifuged at 2749 g for 30 min at 20°C. The remainder was centrifuged at 1500 g for 10 min at 4°C, divided over different 5 ml disposable polystyrene tubes, and stored for 24 h at − 20°C, thereafter at − 80°C until analysis. Plasma was analysed for Zn and Cu concentrations.
After overnight incubation at 4°C, serum was separated by centrifugation at 1500 g for 10 min at 4°C and stored for 24 h at − 20°C, thereafter at − 80°C until analysis. Serum was analysed for ALP, albumin and MT concentrations. Colostrum, milk and the piglets' plasma were analysed for Zn and Cu concentrations. Plasma Cu concentration was not measured as a Zn status biomarker; however, because it uses some of the same transporter mechanisms, it may provide information on the affected pathways( Reference McDowell 15 , Reference Bikker and Jongbloed 18 , Reference Milne, Davies and Nielsen 21 , 22 ).
Analysis of zinc status biomarkers
Plasma samples were deproteinated (Randox ZN2607; Randox Laboratories Limited) by mixing with an equal volume of TCA and centrifuged at 10 000 g for 10 min. The remaining supernatant was used within 2 h to determine plasma Zn and Cu concentrations.
For plasma Zn analysis, the deproteinated plasma was diluted five times with a colour reagent (Randox Kit, ZN2341; Randox Laboratories Limited), and incubated for 5 min at 25°C. Absorbance was measured at a wavelength of 570 nm with a reference wavelength of 620 nm using a microplate reader (EZ reader 400; Biochrom Limited). Plasma Zn concentration was interpolated from the standard multipoint calibration curve. The inter- and intra-assay CV were 2·19 and 3·61 %, respectively. For quality control, pooled porcine plasma samples were spiked using ZnCl2 to calculate the rate of Zn recovery. The regression line of the calibration curve to compare the measured and expected Zn concentrations was as follows: y= 0·0003x+0·0252, R 2 0·9966. The minimum and maximum recovery rates were 99·5 and 119·8 %, respectively.
For plasma Cu analysis, a reagent (Randox Kit, CU2340; Randox Laboratories Limited) was added to the deproteinated plasma, and this solution was incubated for 60 s at 37°C (Memmert Elanco incubation oven; Memmert GmbH). Absorbance was measured at a wavelength of 580 nm (Ultrospec IIE; LKB Biochrom Limited). A colour reagent was added and the solution was mixed. After 5 min of incubation at 37°C, absorbance was measured at the same wavelength. Plasma Cu concentration was interpolated from the standard multipoint calibration curve. The inter-assay CV was 2·83 %. For quality control, pooled porcine plasma samples were spiked using CuCl2.2(H2O) to calculate the rate of Cu recovery. The regression line of the calibration curve to compare the measured and expected Cu concentrations was as follows: y= 0·0006x+0·0806, R 2 0·9912. The minimum and maximum recovery rates were 96·4 and 103·7 %, respectively.
Albumin was determined colorimetrically (Cobas Integra Albumin Gen.2; Roche Diagnostics GmbH), by binding to an anionic dye (bromocresol green) to form a blue–green complex. The total albumin concentration was proportional to the colour intensity and determined by an increase in absorbance at 583 nm. Serum albumin concentrations were within the expected range of the kit (3–96 g/l), and the recovery rate was within 10 % of the initial value at an albumin concentration of 35 g/l. The observed albumin concentrations were daily controlled using an independent human control serum with a high and low known concentration.
MT was determined using competitive ELISA (Porcine Metallothionein (MET) Elisa Kit, E07M0030; BlueGene Biotech Company), including duplicate standards and controls from human origin for each run. The product of the enzyme–substrate reaction forms a blue-coloured complex and turns into yellow after the reaction was stopped by adding a stop solution. The intensity of the colour was measured spectrophotometrically at 450 nm (BEP 2000; Siemens AG). MT concentration was interpolated from the standard curve. The certificate of analysis reported an inter- and intra-assay CV of 6·6–8·2 % and 4·4–5·6 %, respectively. The recovery rates ranged between 94 and 103 %.
ALP concentration was determined colorimetrically (Cobas c systems ALP2; Roche Diagnostics GmbH). In the presence of Mg and Zn, p-nitrophenyl phosphate was cleaved into phosphate and p-nitrophenol. The released p-nitrophenol was directly proportional to the catalytic ALP activity, which is determined by an increase in absorbance at 480/450 nm. The device was calibrated with a known concentration before the analysis to measure the concentration of ALP. Serum ALP concentrations were within the expected range of the kit (5–1200 units/l), and the recovery rate was within 10 % of the initial value at an ALP concentration of 100 units/l. The observed ALP concentrations were daily controlled using an independent human control serum with a high and low known concentration.
Concentration of zinc and copper in colostrum and milk
Colostrum and milk samples (1·5 g) were digested in a microwave oven (MarsX; CEM), using HNO3/H2O2, and analysed for Zn and Cu concentrations by inductively coupled plasma optical emission spectrometry (ICP-OES, Vista MPX; Varian, Inc.). For quality control, two cows' milk samples were spiked, both with 50 and 100 μg/l of Cu and Zn. The recovery rates of spiked Cu and Zn varied between 81·2 and 88·9 %, and between 83·7 and 91·2 %, respectively.
Statistical analysis
The evolution over time during gestation (period between day 0 (insemination) and day 115 (parturition)) and lactation (period between day 118 and day 143 (weaning)) of all biomarkers were analysed using a linear mixed model for each reproductive phase (gestation and lactation), with the biomarker as the outcome and parity, haematocrit, time, interaction between parity and time, and a quadratic time effect as fixed effects. Inclusion of a quadratic time effect allows the identification of a non-linear evolution over time. In case of repeated measures, a random effect for sows was introduced in the model.
Biomarkers in colostrum, milk and the piglets' plasma were analysed using a linear mixed model, with the biomarker as the outcome and all sows' biomarkers at parturition or the average during gestation, and parity as fixed effects. The non-significant biomarkers were removed from the model. Sow was introduced as a random effect to correct for clustering of piglets within sows.
The analysed data were considered sufficiently normally distributed based on the graphical evaluation (histogram and QQ plot) of the residuals. In case of post hoc pairwise testing, P values were corrected with the Tukey–Kramer adjustment for multiple comparisons. All analyses were performed using SAS 9.4 (SAS Institute, Inc.).
Results
The body weight of multiparous sows increased during gestation and decreased after parturition to similar values at weaning of the preceding cycle (Table 3). Primiparous sows showed a lower body weight compared with multiparous sows throughout the reproductive cycle, and had a higher body weight at weaning compared with the start of the study (Table 3).
Table 3 Body weight and back-fat thickness throughout the reproductive cycle of sows (n 15; five primiparous and ten multiparous sows) (Mean values with their standard errors)

a,bMean values within a column with unlike superscript letters were significantly different between different days of the reproductive cycle (P< 0·050).
A,BMean values within a row with unlike superscript letters were significantly different between the primiparous and multiparous sows for the specific day of the reproductive cycle (P< 0·050).
* Day − 5 represents the start of the study, days 63, 84 and 108 represent gestation and day 143 represents weaning.
† Interaction between day (D) and parity (P).
Back-fat thickness decreased during lactation (P< 0·001), independently of parity.
No significant parity effect was found in reproductive performances (Table 4).
Table 4 Reproductive performance of primiparous (n 5) and multiparous (n 10) sows* (Mean values with their standard errors)

* No significant differences were found between the primiparous and multiparous sows (P>0·050).
The haematocrit level (Fig. 1) did not fluctuate significantly throughout gestation (β = 0·019, P= 0·955) and lactation (β = −0·107, P= 0·581), despite the numerically increased percentage at day 21 of gestation. There was also no parity effect for haematocrit during gestation (P= 0·458) and lactation (P= 0·955). The concentrations of plasma Zn (P =0·173 for gestation and P =0·389 for lactation), serum MT (P= 0·127 for gestation and P= 0·514 for lactation), plasma Cu (P= 0·314 for gestation and P= 0·259 for lactation) and serum ALP (P= 0·346 for gestation and P= 0·738 for lactation) were not associated with the haematocrit level. Fluctuation in albumin levels tended to be positively associated with the haematocrit level during gestation (P= 0·086) and lactation (P= 0·049).

Fig. 1 Haematocrit levels throughout gestation and lactation in sows (n 15; five primiparous and ten multiparous sows). Values are means, with their standard errors represented by vertical bars. During gestation and lactation, no fluctuation was observed (P= 0·955 and P= 0·581, respectively).
Despite the parity effect for body weight and a tendency for an interaction between parity and serum albumin concentration during gestation, the fluctuations of the other biomarkers did not differ between the primiparous and multiparous sows. Therefore, average biomarker fluctuations are presented in the figures.
Plasma Zn concentration decreased linearly after insemination (β = −1·934, P< 0·001) and increased quadratically towards parturition (β = 0·012, P< 0·001) (Fig. 2). After parturition, plasma Zn concentration seemed to first increase, then decrease and increase again during the last 14 d of the lactation period. However, there was only a tendency for a quadratic time effect for the last 14 d of lactation (β = 0·118, P= 0·063). The variation between the sows was high, especially at days − 5, 42 and 112 of gestation, and at weaning (day 143).

Fig. 2 Plasma zinc concentrations throughout gestation and lactation in sows (n 15; five primiparous and ten multiparous sows). Values are means, with their standard errors represented by vertical bars. During gestation, plasma zinc concentration decreased linearly after insemination (P< 0·001) and increased quadratically towards parturition (P< 0·001). During lactation, plasma zinc concentration tended to increase quadratically at the end of lactation (P= 0·063). To convert values from μg/dl to μmol/l, multiply by 0·153.
A distinct fluctuation in plasma Cu concentration during gestation was not found (β = 0·408, P= 0·127; Fig. 3). Plasma Cu concentration decreased linearly during lactation (β = −6·716, P< 0·001) and increased quadratically towards weaning (β = 0·126, P= 0·024).

Fig. 3 Plasma copper concentrations throughout gestation and lactation in sows (n 15; five primiparous and ten multiparous sows). Values are means, with their standard errors represented by vertical bars. During gestation, no fluctuation was observed for plasma copper concentration (P= 0·127). During lactation, plasma copper concentration decreased linearly (P< 0·001) and increased quadratically towards weaning (P= 0·024). To convert values from μg/dl to μmol/l, multiply by 0·157.
The total serum albumin concentration tended to decrease linearly during the beginning of gestation, though it tended to decrease less for primiparous sows (tendency for an interaction between parity and day of gestation, P= 0·055). A quadratic time effect was found towards the end of gestation (β = 0·000, P< 0·001; Fig. 4). During lactation, serum albumin concentration decreased linearly (β = −0·039, P= 0·018).

Fig. 4 Total serum albumin concentrations throughout gestation and lactation in sows (n 15; five primiparous (–○–) and ten multiparous (–●–) sows). Values are means, with their standard errors represented by vertical bars. During gestation, serum albumin concentration tended to decrease linearly at the beginning of gestation, though tended to decrease less for primiparous sows (tendency for an interaction between parity and day of gestation, P= 0·055) and increased quadratically towards the end of gestation (P< 0·001). During lactation, serum albumin concentration decreased linearly (P= 0·018). To convert values from g/dl to μmol/l, multiply by 10.
During gestation, serum MT concentration did not change significantly (β = −0·016, P= 0·162) (Fig. 5). Shortly after parturition, MT concentration decreased linearly (β = −0·244, P= 0·001) and increased quadratically towards the end of lactation (β = 0·007, P< 0·001).

Fig. 5 Serum metallothionein (MT) concentrations throughout gestation and lactation in sows (n 15; five primiparous and ten multiparous sows). Values are means, with their standard errors represented by vertical bars. During gestation, no fluctuation was observed for serum MT concentration (P= 0·162). During lactation, serum MT concentration decreased linearly (P= 0·001) and increased quadratically towards the end of lactation (P< 0·001).
Serum ALP concentration increased linearly at the beginning of gestation (β = 0·395, P= 0·002) and decreased quadratically towards parturition (β = −0·003, P= 0·001) (Fig. 6). During the lactation period, no significant fluctuations were observed in ALP concentration (β = 0·859, P= 0·140). No parity effect was found, despite the numerically higher serum ALP concentrations of primiparous sows throughout the reproductive cycle (P= 0·102 for gestation and P= 0·473 for lactation).

Fig. 6 Serum alkaline phosphatase (ALP) concentrations throughout gestation and lactation in sows (n 15; five primiparous and ten multiparous sows). Values are means, with their standard errors represented by vertical bars. During gestation, serum ALP concentration first increased linearly (P= 0·002) and decreased quadratically towards parturition (P= 0·001). During lactation, no fluctuation was observed for serum ALP concentration (P= 0·140).
Biomarkers in piglets' plasma, colostrum and milk
No significant parity effects were found for piglet body weight, haematocrit level, plasma Zn and Cu concentrations, placental weight, colostrum Zn and Cu concentrations, and milk Zn concentration (P>0·050; Table 5). Milk Cu concentration tended to be lower for primiparous sows than for multiparous sows (β = −0·378, P= 0·099).
Table 5 Piglets' indicatives* (n 30) and colostrum and milk zinc and copper concentrations (Mean values with their standard errors)

BW, body weight; HCt, haematocrit.
* Piglets' BW, HCt, and plasma Zn and Cu concentrations were from two randomly selected piglets per sow at parturition (day 115) before colostrum intake.
At parturition, serum albumin concentration of the sow tended to be associated with the average body weight of her piglets born alive (β = 0·297, P= 0·060).
Plasma Zn concentration of the piglets was associated with serum MT concentration of the sow during gestation and at parturition (β = 11·891, P= 0·036 and β = 14·261, P= 0·028, respectively) and associated with the haematocrit level of piglets (β = −1·732, P= 0·032).
Discussion
Fluctuations of Zn status biomarkers during gestation and lactation differed distinctly between biomarkers and were independent of parity (except for serum albumin). These differences are probably related to the respective roles of these biomarkers in Zn homeostasis throughout the different phases of the reproductive cycle, as has been shown in other animal species and human subjects. However, the observed evolution over time might have been partly influenced by random period effects, such as temperature.
During gestation, Zn requirements are increased to support embryogenesis and fetal growth and development( Reference Mahan 1 – Reference Caulfield, Donangelo and Chen 4 , Reference King 23 , Reference Donangelo and King 24 ), resulting in homeostatic adjustments to (re)distribute more Zn to maternal tissues (e.g. placenta, liver and bone) and to the fetus( Reference King 23 – Reference Krebs 26 ). This Zn sequestration to maternal tissues and the fetus may decrease the total circulating Zn concentration( Reference Donangelo and King 24 ).
In the present study, plasma Zn concentration decreased during gestation as hypothesised based on other studies in sows( Reference Hill, Miller and Stowe 27 – Reference Girard, Robert and Matte 29 ). This conceivably reflects the increased intracellular Zn need to protect maternal erythrocytes from oxidative stress, thereby increasing erythrocyte Zn and MT concentrations (due to the synthesis of carbonic anhydrase) and decreasing plasma Zn concentrations( Reference Donangelo and King 24 , Reference Tamura and Goldenberg 25 , Reference Caulfield, Zavaleta and Figueroa 30 ).
Zn is predominantly bound to albumin after absorption and has a high affinity to albumin( Reference McDowell 15 , Reference Peters 16 ). A lower Zn fraction bound to albumin or a lower affinity of Zn for albumin was mentioned to explain the decreased plasma Zn concentration. Interestingly, the total serum albumin concentration in the present study increased during gestation, hence not reflecting the decline in Zn concentration, as expected and as has been found in other studies in women( Reference Maia, Figueiredo and Anastácio 3 , Reference Hambidge, Krebs and Jacobs 31 ).
Whereas albumin serves as an important Zn transporter, MT is involved in Zn transfer within the intestinal mucosal cell, regulating the quantity of Zn absorbed( Reference McDowell 15 , Reference Cousins, Filer and Ziegler 17 , Reference Bikker and Jongbloed 18 ). When Zn is bound to albumin in plasma and entering the liver, MT is synthesised. MT in the liver removes Zn from the plasma and redistributes it to various tissues( Reference McDowell 15 , Reference King 23 , Reference Bremner, Anke, Meissner and Mills 32 ). Hepatic MT synthesis is influenced by dietary Zn intake as well as plasma Zn concentrations( Reference McDowell 15 , Reference Cousins, Filer and Ziegler 17 , Reference Richards 33 , Reference Gibson 34 ), suggesting that serum MT concentration reflects Zn absorption. This is confirmed in the present study, where serum MT concentration fluctuated similarly to plasma Zn concentrations during gestation but not at parturition when dietary Zn intake was low.
Plasma Cu can be bound to MT as well, but fluctuations in plasma Cu concentration in the present study are comparable with other studies in sows( Reference Girard, Robert and Matte 29 , Reference Richards 33 ), women( Reference Álvarez, Castañón and Ruata 35 ) and ewes( Reference Elnageeb and Adelatif 36 ), and therefore suggests that plasma Cu was not interfering with the fluctuation of serum MT. This was also observed in the study by López-Alonso et al. ( Reference López-Alonso, Benedito and Garcia-Vaquero 37 ), where liver and kidney MT concentrations were highly dependent on the Zn status of the pig, and neither Cu nor Cd displaced Zn from MT.
ALP is found in the blood and bone and is involved in the formation of hydroxyapatite( Reference Peters 16 , Reference van Riet, Millet and Aluwé 38 ), which is important for regulating ordered mineral deposition during bone formation( Reference van Riet, Millet and Aluwé 38 – Reference Clarke 40 ). ALP concentration increased during gestation and decreased towards parturition in the present study. This fluctuation may indicate that ALP is related to bone formation in the process of bone remodelling.
During lactation, Zn requirements are increased to support milk synthesis and secretion( Reference Donangelo and King 24 ), resulting in a (re)distribution of Zn to the mammary gland. This occurs by mobilising Zn from involuting tissues (uterus) and maternal blood after parturition, mobilisation from the bone, and by increased efficiency of Zn absorption in the intestine( Reference Donangelo and King 24 – Reference Krebs 26 , Reference Swanson and King 41 , Reference Moser-Veillon 42 ). The distribution of Zn to the mammary gland may affect Zn-related blood parameters differently than during gestation( Reference Donangelo and King 24 ).
Towards the end of lactation, plasma Zn concentrations in the present study seemed to return to initial values at early gestation, probably resulting from increased absorption or decreased nutrient requirements( Reference Donangelo and King 24 , Reference Liesegang, Risteli and Wanner 43 ). These plasma Zn fluctuations during lactation correspond to other studies in sows( Reference Girard, Robert and Matte 29 ), women( Reference Donangelo, Vargas Zapata and Woodhouse 2 ) and sheep( Reference Gürdoğan, Yildiz and Balikci 44 ), except for the study by Kalinowski & Chavez( Reference Kalinowski and Chavez 28 ), who did not observe an increase in plasma Zn concentration during lactation in sows. However, in that study, no blood samples were analysed after 2 weeks of lactation.
Similarly to fluctuation in serum albumin concentrations during gestation, total serum albumin concentrations did not reflect the changes in plasma Zn concentration during lactation in the present study: the increase in plasma Zn concentrations coincided with an increase in serum albumin concentrations. Zn is then rapidly removed from albumin and transferred to other tissues, thereby ensuring the capacity to maintain Zn homeostasis. However, this was not demonstrated in the present study or other studies in women( Reference Maia, Figueiredo and Anastácio 3 , Reference Hambidge, Krebs and Jacobs 31 ).
Serum MT concentrations increased at the end of lactation in the present study. This increase agrees with increased Zn absorption, in which more plasma Zn enters the liver where hepatic MT is synthesised to sequester Zn from the plasma to the tissues.
The fact that plasma Cu concentrations decreased towards the end of lactation, as opposed to plasma Zn concentrations, demonstrates that plasma mineral concentrations probably reflect dietary intakes. Zn is an important antagonist of Cu, hence the rise in plasma Zn concentrations towards the end of lactation combined with a decrease in plasma Cu concentrations seems logical and corresponds to other studies in sows( Reference Kalinowski and Chavez 28 , Reference Girard, Robert and Matte 29 ) and ewes( Reference Elnageeb and Adelatif 36 ).
The mobilised and absorbed Zn will be used for milk production to provide Zn to the piglets. Plasma Zn concentration in piglets before colostrum intake may reflect the dietary Zn intake levels of sows during gestation( Reference Mahan 1 , Reference Peters 16 , Reference Henkin, Marshall and Meret 45 – Reference Hill, Miller and Whetter 47 ). However, in the present study, maternal plasma Zn concentration was not associated with the piglets' plasma Zn concentration. Interestingly, maternal serum MT concentration was significantly associated with the piglets' plasma Zn concentration: higher MT concentrations in sows during gestation and at parturition increased plasma Zn concentration in the piglets' plasma.
Additionally, fluctuations of Zn status biomarkers may identify critical periods throughout the reproductive cycle during which high Zn requirements are not met by dietary Zn intake. It has been shown that Zn deficiency during gestation may affect the growth and development of the fetus, induce complications at birth, and limit the metabolic adaptation capacity of the sow( Reference Álvarez, Castañón and Ruata 35 , Reference Cherry, Bemnett and Bazzano 48 – Reference Apgar and Fitzgerald 50 ).
In the literature, marginal values for plasma/serum Zn concentrations for pigs have been suggested( Reference Suttle 51 ), with a lower limit of normality of 60 μg/dl (9·2 μmol/l) and a normal marginal range for mean serum concentrations between 40 and 70 μg/dl (6·1–10·7 μmol/l). In the present study, the average plasma Zn concentration was 71·5 μg/dl (10·9 μmol/l) during gestation and 66·9 μg/dl (10·2 μmol/l) during lactation. The sows in the present study had on average sufficient plasma Zn levels. However, at days 42, 84, 108, 112, 118, 122 and 129, the average plasma Zn concentrations were below the normal range defined by Suttle( Reference Suttle 51 ), though sows can replace their Zn stores towards weaning. The dietary Zn concentration was above the Zn requirements, and no conclusion can be drawn for the presence of marginal Zn deficiency. Furthermore, plasma Zn concentrations varied widely among sows, which also hampers sound conclusions in individual sows.
Conclusion
Zn status shows distinct fluctuations throughout the reproductive cycle of sows, independently of parity. However, this Zn status pattern differs according to the chosen Zn status biomarker. Therefore, assessing Zn status in breeding sows should best be determined with multiple biomarkers. For the present study, a combination of plasma Zn and serum MT seemed most suitable to assess the Zn status.
Sound conclusions regarding critical periods for Zn status throughout the reproductive cycle in sows could not be distinguished in the present study. For future studies, it is good to take into account that fluctuations in plasma Zn concentrations over a reproductive cycle are noticeable. After periods of lower plasma Zn concentration at the end of gestation and throughout lactation, plasma Zn concentrations increase towards weaning.
Acknowledgements
The authors thank the technicians M. van Yperen and T. Martens, animal caretakers of the ILVO experimental farm, and colleagues who were ‘sow sitting’ for the wonderful assistance and support. The authors also thank M. Levenson for English-language editing.
The present study, part of the postgraduate study of the first author, was supported by the Institute for Promotion of Innovation through Science and Technology in Flanders (IWT, grant no. 090938), and co-funded by Orffa, VDV Beton, Boerenbond, AVEVE, INVE and Boehringer Ingelheim. These funders had no role in the design and analysis of the study or in the writing of this article.
The authors' contributions are as follows: G. P. J. J. and S. M. were supervisors; G. P. J. J., S. M., F. A. M. T. and D. M. were involved in the writing of the research proposal; K. C. M. L., E. N., A. C. and G. D. L. contributed to the practical work, including the analyses of some biomarkers; B. A. provided guidance for the statistical analyses. All authors examined the article critically and added insightful and useful comments to the final version of the manuscript.
None of the authors has any conflict of interest to declare.