Introduction
Modafinil (2-[(diphenylmethyl)sulfinyl]acetamide) is an FDA-approved wake-promoting drug that has found widespread usage for remediation of cognitive dysfunction in neuropsychiatric disorders (Gerrard and Malcolm, Reference Gerrard and Malcolm2007; Wise et al., Reference Wise, Arand, Auger, Brooks and Watson2007; Minzenberg and Carter, Reference Minzenberg and Carter2008). A broad range of studies on rodents, adults and across various psychiatric disorders have shown that well-tolerated doses of modafinil improve cognitive function, especially working memory, episodic memory and processes requiring cognitive control (Minzenberg and Carter, Reference Minzenberg and Carter2008). Modafinil shows its therapeutic efficacies in the treatment of attention deficit hyperactivity disorder, Parkinson’s disease, drug addiction and depression owing to its positive effects on locomotor activity, wakefulness and cognitive ability (Kuczenski et al., Reference Kuczenski, Segal and Aizenstein1991; Edgar and Seidel, Reference Edgar and Seidel1997; Kuczenski and Segal, Reference Kuczenski and Segal2001; Wisor et al., Reference Wisor, Nishino, Sora, Uhl, Mignot and Edgar2001; Barch and Carter, Reference Barch and Carter2005; Ishizuka et al., Reference Ishizuka, Murakami and Yamatodani2008; Kumar, Reference Kumar2008). While most of the therapeutic psychostimulants, such as methylphenidate, have the potential for abuse, modafinil does not show the side effects commonly experienced with amphetamine-like drugs and exhibits low propensity to induce dependency (Martinez-Raga et al., Reference Martínez-Raga, Knecht and Cepeda2008). This unique pharmacological feature of modafinil enhances the motivation of its usage among healthy individuals such as on-call physicians and people within academia to promote vigilance under hard working conditions (Greely et al., Reference Greely, Sahakian, Harris, Kessler, Gazzaniga, Campbell and Farah2008; Franke et al., Reference Franke, Bagusat, Dietz, Hoffmann, Simon, Ulrich and Lieb2013).
Modafinil appears to improve cognitive function by enhancing the activity of glutamate, norepinephrine, serotonin, histamine, orexin and dopamine while inducing a reduction in GABA content in the central nervous system (Ferraro et al., Reference Ferraro, Fuxe, Tanganelli, Fernandez, Rambert and Antonelli2000; Willie et al., Reference Willie, Renthal, Chemelli, Miller, Scammell, Yanagisawa and Sinton2005; Madras et al., Reference Madras, Xie, Lin, Jassen, Panas, Lynch, Johnson, Livni, Spencer and Bonab2006; Huang et al., Reference Huang, Zhang, Tang, Wang and Wang2008; Ishizuka et al., Reference Ishizuka, Murotani and Yamatodani2010; Haris et al., Reference Haris, Singh, Cai, Nath, Verma, Nanga, Hariharan, Detre, Epperson and Reddy2014). However, the exact mechanism by which modafinil promotes cognition has yet to be fully understood. One of the most accepted theories concerning the mechanism of modafinil intervention in the catecholamine system of the brain is its binding to the dopamine transporter (DAT) and norepinephrine transporter (Madras et al., Reference Madras, Xie, Lin, Jassen, Panas, Lynch, Johnson, Livni, Spencer and Bonab2006; Kalaba et al., Reference Kalaba, Aher, Ilic, Dragacevic, Wieder, Miklosi, Zehl, Wackerlig, Roller and Beryozkina2017). This directly enhances extracellular dopamine and norepinephrine, respectively, and indirectly raises the levels of serotonin, glutamate and histamine, while simultaneously attenuating GABAergic neurotransmission (Minzenberg and Carter, Reference Minzenberg and Carter2008; Mereu et al., Reference Mereu, Bonci, Newman and Tanda2013). Wisor et al. (Reference Wisor, Nishino, Sora, Uhl, Mignot and Edgar2001) showed that the wake-promoting effects of modafinil are abolished in DAT knockout mice, suggesting that modafinil needs DATs to achieve its wake-promoting action. Several studies suggested that modafinil also regulates the activity of histamine, hypocretin, adrenergic and glutamate receptors, respectively (Ferraro et al., Reference Ferraro, Antonelli, Tanganelli, O’Connor, de la Mora, Mendez-Franco, Rambert and Fuxe1999). However, modafinil has also been shown to demonstrate a weak selective affinity to DAT, and does not bind at all to other binding sites and receptors tested in vitro (Mignot et al., Reference Mignot, Nishino, Guilleminault and Dement1994). This paradox of reports about the modafinil function on DAT enhances the curiosity about its mechanism of action in increasing the dopamine level in the brain.
So far, most studies have been done at extracellular localised sites of action for modafinil in the brain (Keating and Raffin, Reference Keating and Raffin2005; Gerrard and Malcolm, Reference Gerrard and Malcolm2007; Minzenberg and Carter, Reference Minzenberg and Carter2008). The effects of modafinil on the alteration of the processes at single-cell levels also need to be investigated to understand the modafinil’s primary mechanism of action. During exocytosis, the synaptic vesicles in the neurons mostly partially release their contents into a synaptic gap (Oleinic et al., Reference Oleinick, Hu, Ren, Tian, Svir and Amatore2015; Li et al., Reference Li, Dunevall and Ewing2016a, Reference Li, Dunevall and Ewing2016b; Ren et al., Reference Ren, Mellander, Keighron, Cans, Kurczy, Svir, Oleinick, Amatore and Ewing2016; Dunevall et al., Reference Dunevall, Majdi, Larsson and Ewing2017; Phan et al., Reference Phan, Li and Ewing2017; Ranjbari et al., Reference Ranjbari, Majdi and Ewing2019; Zhu et al., Reference Zhu, Gu, Dunevall, Ren, Zhou and Ewing2019; Larsson et al., Reference Larsson, Majdi, Oleinick, Svir, Dunevall, Amatore and Ewing2020). In the partial release model of exocytosis, only a fraction of vesicular content is released in the synaptic cleft per individual event, unlike the traditional model that suggests a full opening of the vesicle membrane and full release. The concept of partial release is important for understanding the regulation of neurotransmitter secretion during individual exocytosis events in intercellular communication and might be used to influence development of pharmaceutical targets or to develop models of cellular plasticity in cognition, learning and disease.
The exocytosis process is very rapid and thus, the analytical techniques with high temporal resolution are required for its in vivo investigation. So far, several techniques, including optical spectroscopy, patch-clamp capacitance detection, as well as amperometry methods have been developed to study the exocytosis (Neher and Marty, Reference Neher and Marty1982; Oberhauser et al., Reference Oberhauser, Robinson and Fernandez1996; Albillos et al., Reference Albillos, Dernick, Horstmann, Almers, De Toledo and Lindau1997; Omiatek et al., Reference Omiatek, Cans, Heien and Ewing2010; Hoffman et al., Reference Hoffman, Spivak and Lupica2016; Oomen et al., Reference Oomen, Aref, Kaya, Phan and Ewing2018). Among these, single-cell amperometry (SCA) offers the unique advantage of providing quantitative information about the number of catecholamines expelled and precise dynamic characteristics with high sensitivity and sub-millisecond time resolution (Ren et al., Reference Ren, Mellander, Keighron, Cans, Kurczy, Svir, Oleinick, Amatore and Ewing2016; Oomen et al., Reference Oomen, Aref, Kaya, Phan and Ewing2018; Ranjbari et al., Reference Ranjbari, Majdi and Ewing2019). Intracellular vesicle impact electrochemical cytometry (IVIEC), another amperometric method developed by our group using conical nanotip electrodes, allows quantification of individual vesicle content inside the natural environment of the cell (Oleinic et al., Reference Oleinick, Hu, Ren, Tian, Svir and Amatore2015; Li et al., Reference Li, Dunevall and Ewing2016a, Reference Li, Dunevall and Ewing2016b).
The membrane fusion step in the exocytosis process occurs through a lipid-dominated mechanism initiated and controlled by proteins. The lipid composition of the cell membrane affects many mechanisms of cell function including controlling the dynamics of intercellular communication via exocytosis. Time-of-flight secondary ion mass spectrometry (ToF-SIMS) is a high throughput, label-free and semiquantitative imaging technique. ToF-SIMS has recently attracted increasing interest in the detection of many major classes of biological substances including small molecules, small peptides, as well as lipid species with mass to charge ratio m/z < 1,500 Da (Lanekoff et al., Reference Lanekoff, Sjövall and Ewing2011; Pauloehrl et al., Reference Pauloehrl, Welle, Oehlenschlaeger and Barner-Kowollik2013; Dowlatshahi Pour et al., Reference Dowlatshahi Pour, Jennische, Lange, Ewing and Malmberg2016).
In this article, we took advantages of both powerful amperometry techniques, SCA and IVIEC, to investigate the influence of modafinil in the exocytosis process. Combining the results of these two techniques offers the fraction of electroactive transmitters released during exocytosis. Pheochromocytoma (PC12) cells as models for exocytosis were employed to investigate the mechanism of modafinil action on this process. We also employed ToF-SIMS to investigate the extent of different lipid depletion or insertion into the membrane of modafinil-treated cells. Correlation of the results obtained by ToF-SIMS analysis to the amperometry results provides us with a potentially novel mechanism for how modafinil influences membrane phospholipid levels and this chemical composition mediates plasticity at the presynaptic cell.
Results and discussion
Modafinil upregulates both catecholamine release and vesicular storage
The effect of modafinil on localised sites of action in the brain has been reported in the literature (Ferraro et al., Reference Ferraro, Fuxe, Tanganelli, Fernandez, Rambert and Antonelli2000; Willie et al., Reference Willie, Renthal, Chemelli, Miller, Scammell, Yanagisawa and Sinton2005; Madras et al., Reference Madras, Xie, Lin, Jassen, Panas, Lynch, Johnson, Livni, Spencer and Bonab2006; Huang et al., Reference Huang, Zhang, Tang, Wang and Wang2008; Ishizuka et al., Reference Ishizuka, Murotani and Yamatodani2010; Haris et al., Reference Haris, Singh, Cai, Nath, Verma, Nanga, Hariharan, Detre, Epperson and Reddy2014); however, there is no study that specifically investigates the effect of modafinil at the cellular and sub-cellular levels. We performed SCA on PC12 cells (Fig. 1A) to directly measure the vesicular release of catecholamines during exocytosis after incubating for 3 h with different concentrations of modafinil. A schematic illustration of the SCA experiment and some typical traces for control and modafinil-treated cells are shown in Supplementary Fig. S1. The number of catecholamine molecules extruded from PC12 cells treated with (10 μM) or without modafinil (control) is plotted as a normalised frequency histogram in Fig. 1B. The proportion of large release events increases after modafinil treatment. As the number of molecules released per exocytotic event deviates from a normal distribution in each cell, for comparisons the median of the data was pooled from each cell and the mean of the pooled medians was used. This minimises the impact of cell-to-cell variation. Fig. 1C indicates that the number of molecules released during exocytosis is significantly increased at 10 and 100 μM modafinil concentration and it seems to be a dose-dependent change from 0 to 10 μM.

Fig. 1. (A) Microscope view during the single-cell amperometry (SCA) experiment (scale bar = 20 μm). (B) Normalised frequency histogram for the molecules released from control cells (blue) and modafinil (10 μM)-treated cells (red). The distribution fits are shown for control cells (dashed line) and modafinil-treated cells (solid line). (C–G) SCA results for exocytosis events after incubation of PC12 cells in modafinil at different concentrations [0 (control), 1, 10 and 100 μM]. (C) Number of molecules released per exocytosis events, (D) time of fusion pore opening (t rise), (E) the time over which the pore remains open (t half), (F) time for fusion pore closing (t fall) and (G) maximum current (I max) caused by oxidation of catecholamines at the surface of the electrode. Number of analysed cells: control (15 cells), modafinil 1 μM (14 cells), modafinil 10 μM (14 cells) and modafinil 100 μM (16 cells). Each treatment result was compared with control by a two-tailed Mann–Whitney rank-sum test, *p < 0.05, **p < 0.01 and ***p < 0.001. (H) Representative average transient amperometric spikes for a control (black), and a 10 μM modafinil-treated cell (red).
To determine the effects of modafinil on exocytosis dynamics, we evaluated the time course of spikes, rise time (t rise), halfwidth (t half) and fall time (t fall) time. The values of t rise, t half and t fall are characteristics of the pore opening time, pore survival duration and the pore decay time, respectively (Li et al., Reference Li, Dunevall and Ewing2016a, Reference Li, Dunevall and Ewing2016b). The results illustrated in Fig. 1D–Freveal that modafinil appears to slow the exocytosis dynamics by lengthening the release events. The value I max corresponds to the membrane pore size during the release event and displays no significant difference for modafinil-treated cells (Fig. 1G). Modafinil, therefore, promotes exocytotic release at higher concentrations (10 and 100 μM) by lengthening the time (Fig. 1H) for molecules to diffuse from the inner vesicle through the fusion pore to the extracellular environment.
We employed IVIEC to measure the vesicular cargo of the PC12 cells with or without modafinil treatment. In IVIEC experiments, the tip of the nanoelectrode is slowly placed on top of the cell, then gently penetrates through the cell membrane into the cytoplasm where vesicles can diffuse to the electrode surface (Fig. 2) and their content quantified. A potential of +700 mV at the electrode results in vesicles opening via an electroporation mechanism (Lovrić et al., Reference Lovrić, Najafinobar, Dunevall, Majdi, Svir, Oleinick, Amatore and Ewing2016; Ranjbari et al., Reference Ranjbari, Taleat, Mapar, Aref, Dunevall and Ewing2020). Typical traces for control and modafinil-treated cells as well as schematic illustration of the IVIEC are shown in Supplementary Fig. S2. Oxidation of the neurotransmitter content is observed as current transients and the integration of these transients gives the total number of catecholamine molecules stored in each individual vesicle. The pooled numbers of molecules showing the vesicular cargo are shown as a normalised frequency histogram and a bar graph in Fig. 2B and Fig. 2C, respectively. Cells exposed to modafinil are observed to have a 45% enhancement in vesicle content compared to control cells (Fig. 2c).

Fig. 2. (A) Microscope view during the intracellular vesicle impact electrochemical cytometry experiment (scale bar = 20 μm). (B) Normalised frequency histogram for the number of molecules stored in vesicles in control (blue) and modafinil-treated cells (red). The distribution fits are shown for control cells with a dashed line and modafinil-treated cells with a solid line. (C) Average of the number of molecules stored per vesicle in PC12 cells treated without (control) or with 10 μM modafinil. Number of analysed cells: control cells (15 cells) and modafinil-treated cells (18 cells). The results were compared by a two-tailed Mann–Whitney rank-sum test, *p < 0.05, **p < 0.01 and ***p < 0.001.
The fraction of release, calculated by dividing the number of molecules released during exocytosis by the number of molecules stored in the vesicles, shows an increase from 67% for control cells to 74% for modafinil-treated PC12 cells. The larger fraction released despite the increased vesicular content results from a slower event time (t rise, t 1/2 and t fall) where the exocytotic fusion pore is open for a longer time, compared to the control cells. The calculated fraction of release for both control and modafinil-treated cells also supports the partial release hypothesis of individual exocytosis events, confirming that only a fraction of the vesicle’s catecholamine content is released during exocytosis (Ren et al., Reference Ren, Mellander, Keighron, Cans, Kurczy, Svir, Oleinick, Amatore and Ewing2016; Ranjbari et al., Reference Ranjbari, Majdi and Ewing2019).
The increased vesicle storage following treatment with modafinil might be interpreted as resulting from the function of vesicular monoamine transporter-2 (VMAT-2). This integral membrane counter-transporter protein uses the pH gradient and V-ATPase to allow two protons to exit the vesicle while simultaneously translocating a single monoamine molecule into the vesicle (Guillot et al., Reference Guillot and Miller2009). ATP is required for loading the vesicles with catecholamines. Larsson et al. (Reference Larsson, Majdi, Borges and Ewing2019) previously reported that extracellular exposure of chromaffin cells to both norepinephrine and ATP significantly increased both vesicular transmitter storage and exocytotic release, which is similar to the result shown here. Interestingly, it has been suggested by Gerrard and Malcolm that modafinil might increase ATP production by targeting the mitochondria and enhancing the ability of cytochrome c to accept and donate electrons by allosteric modification or a catalytic mechanism. This mechanism reduces net hydrogen peroxide levels and superoxide production with a concomitant increase in ATP production (Gerrard and Malcolm, Reference Gerrard and Malcolm2007).
Furthermore, inspecting the H+-monoamine exchange activity of VMAT-2 suggests another mechanism by which modafinil might affect ATP dependent activities. It has been reported in previous studies that when HT‐22 hippocampal neuronal cells were treated with modafinil, vesicular organelles were less acidic (Cao et al., Reference Cao, Li, Liu, Wu, Huang, Wang, Lan, Zheng, Xing and Zhou2019). Blackmore et al. (Reference Blackmore, Varro, Dimaline, Bishop, Gallacher and Dockray2001) previously showed that the increased pH of secretory vesicles can be attributed to the transport activity of the protein VMAT-2. They indicated that incubation with reserpine, a VMAT inhibitor, and vesicular content suppressor, significantly lowered intra-vesicular pH in VMAT-2-expressing cells (Blackmore et al., Reference Blackmore, Varro, Dimaline, Bishop, Gallacher and Dockray2001). Hence, it is possible that the incubation of the PC12 cells with modafinil increases VMAT-2 exchange of intra-vesicular protons with extra-vesicular monoamines, thereby enhancing vesicular catecholamine storage. This hypothesis is also consistent with the neuroprotective action of modafinil against aberrant autophagy and apoptosis (Fuxe et al., Reference Fuxe, Janson, Rosen, Finnman, Tanganelli, Morari, Goldstein and Agnati1992; Blackmore et al., Reference Blackmore, Varro, Dimaline, Bishop, Gallacher and Dockray2001).
As the time course is increased for exocytosis events observed in the modafinil-treated PC12 cells (Fig. 1D–F) the rates of both dilation and constriction of the fusion pore are longer. In contrast to modafinil, previous reports examining cocaine, a psychostimulant associated with cognitive dysfunction, showed it causes faster events and a lower fraction released (Zhu et al., Reference Zhu, Gu, Dunevall, Ren, Zhou and Ewing2019). Actin polymerisation plays a crucial role in the exocytotic release dynamics (Trouillon and Ewing, Reference Trouillon and Ewing2014). Elevation of cytoplasmic ATP level stiffens the actin as a cytoskeletal protein that forms a sub-membrane filamentous network (Scheme 1) (Janmey et al., Reference Janmey, Hvidt, Oster, Lamb, Stossel and Hartwig1990). This physical change in the cytoskeleton might result in the longer exocytotic fusion pore opening observed for t rise and t 1/2 (Majdi et al., Reference Majdi, Larsson, Najafinobar, Borges and Ewing2019). Taken together, we suggest that modafinil might affect the mitochondria as a target in the cell, increasing the ability of cytochrome c for electron exchange, enhancing ATP production to eventually increase both the vesicular catecholamine storage and exocytotic release.

Scheme 1. Suggested scheme for the different effects of modafinil on vesicle content and exocytosis. Control cell (A) and modafinil-treated cell (B). In the modafinil-treated cell, the combination of higher phosphatidylinositol (PI) and phosphatidylethanolamine (PE) in the membrane and the PE elevation leading to enhanced ATP levels change the cellular actin scaffold and allow the pore to stay open longer allowing a higher fraction of messenger to be released.
Another explanation for the enhancement of vesicular storage might be that modafinil triggers the biosynthesis of the catecholamines. Tyrosine hydroxylase catalyses the formation of L-DOPA from tyrosine. It has been reported that modafinil counteracts the reduction of tyrosine hydroxylase immunoreactivity and dopamine storage in nigrostriatal dopamine neurons in the male rat (Ueki et al., Reference Ueki, Rosen, Andbjer, Finnman, Altamimi, Janson, Goldstein, Agnati and Fuxe1993). Thus, modafinil might indirectly enhance the biosynthesized catecholamines to eventually improve the content of vesicles. Although modafinil appears to increase the vesicular content of the PC12 cells, this finding needs further investigations to find the exact mechanism by which modafinil increases the vesicular content.
Modafinil alters the lipid composition of the PC12 cell membrane
Although many proteins are involved in exocytosis, membrane phospholipids are directly involved in the physical processes and likely play a vital role in changing membrane fusion dynamics between secretory vesicles and the surrounding cell membrane (Uchiyama et al., Reference Uchiyama, Maxson, Sawada, Nakano and Ewing2007; Aref et al., Reference Aref, Ranjbari, Romiani and Ewing2020). Lipids have intrinsic shapes depending on the relatively different sizes of their headgroups as well as their acyl chain length and saturation (McMahon et al., Reference McMahon and Boucrot2015). For example, phosphatidylcholine (PC) has a cylindrical shape and therefore forms a flat monolayer. A negative curvature, where a monolayer of the lipid headgroup bends closer together, is generated from conically shaped lipids, such as phosphatidylethanolamine (PE), diacylglycerol, phosphatidic acid or cardiolipin. In contrast, inverted conical lipids with larger headgroups, including lysophosphatidylcholine and phosphatidylinositol (PI), form positive curvatures. The changes in lipid composition cause the alteration of membrane curvature, which fasten or slow down the exocytotic release. Previously, Uchiyama et al. (Reference Uchiyama, Maxson, Sawada, Nakano and Ewing2007) and Aref et al. (Reference Aref, Ranjbari, Romiani and Ewing2020) showed that an enhancement in either extracellular or intracellular PC, a low-curvature lipid, induces a reduction in the number of molecules released during exocytosis. Also, it has been reported that an increase of PE, a high curvature lipid, in the inner leaflet causes an enhancement in the time courses of exocytosis (t rise, t 1/2 and t fall) (Aref et al., Reference Aref, Ranjbari, Romiani and Ewing2020). Understanding the effect of modafinil on the lipid composition and molecular biophysics of the cell membrane, therefore, might give us more insight into the chemical mechanism of how modafinil regulates exocytosis dynamics.
ToF-SIMS analysis was employed to measure and correlate lipid alteration in the cell membrane following modafinil treatment. The TOF.SIMS 5 instrument (ION-TOF GmbH, Germany) was equipped with a 25 keV bismuth primary ion beam. Owing to the high energy of the primary ion beam, the molecular information in ToF-SIMS was limited to fragments. Signals for the PC’s peak fragments were obtained in the positive ion mode, while the negative ion mode was used to identify PE and PI. The fragments [C5H13PO3N]+ at m/z 166, [C5H15PO4N]+ at m/z 184 and [C8H19PO4N]+ at m/z 224 represent PC species (Phan et al., Reference Phan, Munem, Ewing and Fletcher2017). The peaks at m/z 140 ([C2H7PO4N]−) and 180 ([C5H11PNO4]−) are characteristic of PE species. Additionally, the peaks at m/z 153 ([C3H6PO5]−) and m/z 241 ([C6H10PO8]−) originate from PI species. The normalised intensities of fragment peaks were compared by t test among the control and cells treated with different concentrations of modafinil. Fig. 3 reveals a dramatic depletion of PC fragments at m/z 166, 184 and 224 following modafinil treatment, and this depletion effect is larger at higher concentration of modafinil showing dose-dependence. In contrast to PC, there is a significant elevation in the abundance of PE fragments at m/z 140 and 180 and PI fragments at m/z 153 and 241 in the cells treated with high modafinil concentrations of 10 and 100 μM (Fig. 4). Again, the effect of modafinil is dose-dependent from 0 to 10 μM of modafinil while after that the changes in the intensity are not significant. Although, results in negative ion mode revealed an enhancement in the high curvature lipids following the treatment of the cells with modafinil, but some other lipids such as FA (16:0) and FA (18:1) decreased in their signal intensity in the ToF-SIMS at negative polarity (Supplementary Fig. S3A). Moreover, in the positive ion mode, we observed some lipids such as cholesterol and vitamin E that remained statistically unchanged (Supplementary Fig. S3B).
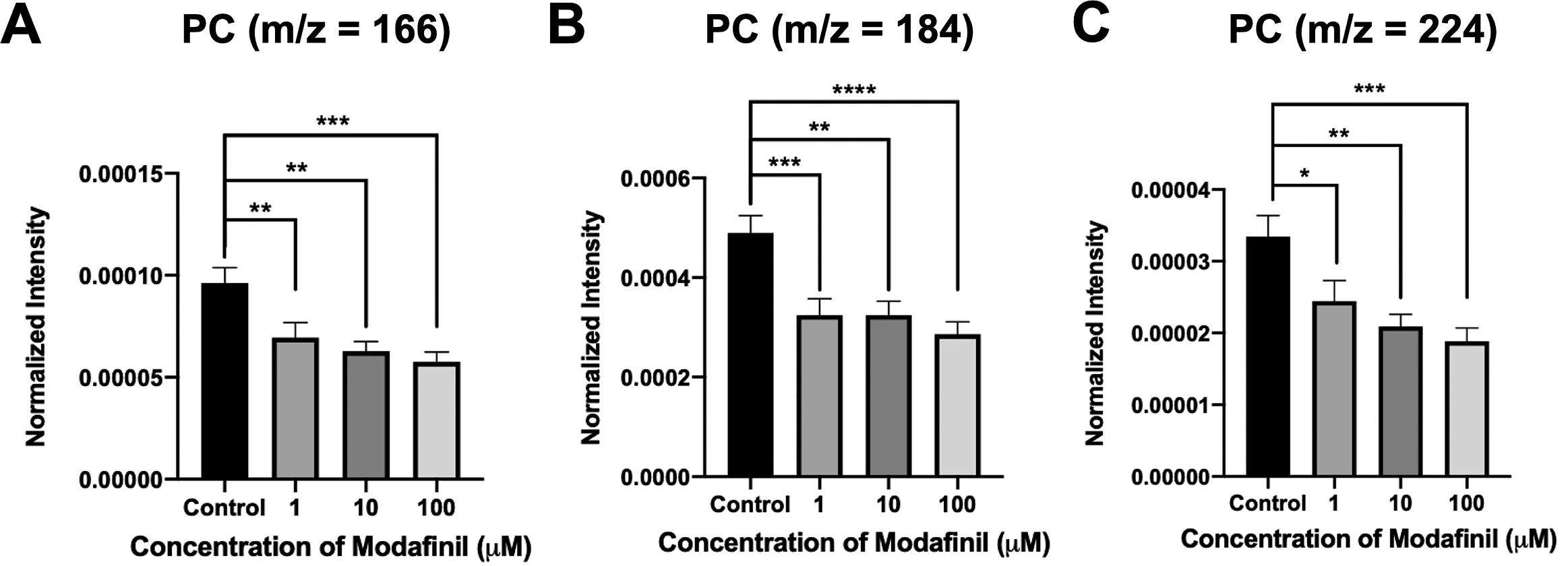
Fig. 3. Normalised intensity for positive lipid head groups at (A) m/z 166 (PC: [C5H13PO3N]+), (B) m/z 184 (PC: [C5H15PO4N]+) and (C) m/z 224 (PC: [C8H19PO4N]+) in the cell membrane as a function of modafinil concentration. Each treatment result was compared with control by a two-tailed Mann–Whitney rank-sum test, *p < 0.05, **p < 0.01 and ***p < 0.001.
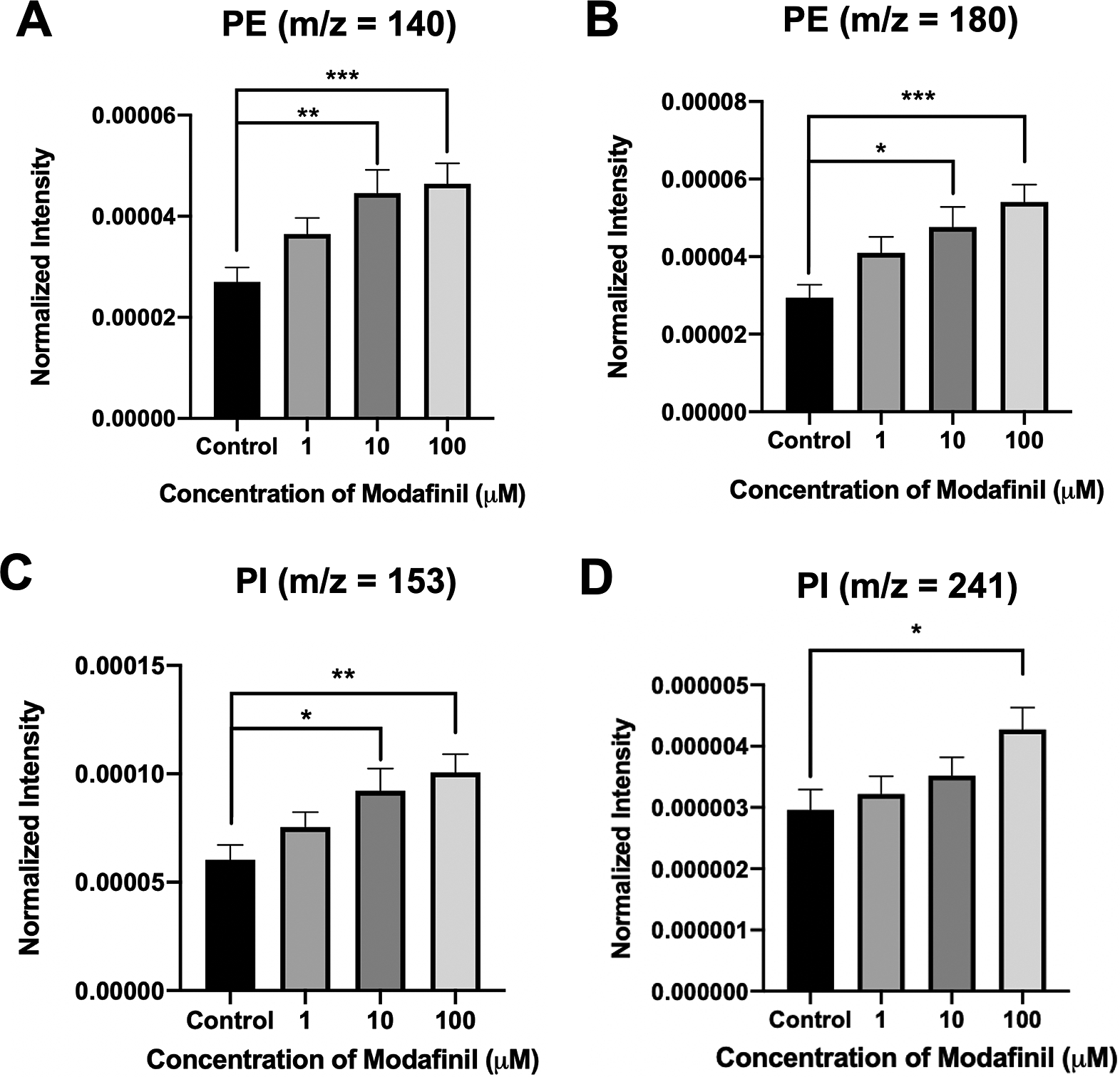
Fig. 4. Normalised intensity for negative lipid head groups at (A) m/z 140 (PE: [C2H7PO4N]ˉ), (B) m/z 180 (PE: [C5H11PNO4]ˉ), (C) m/z 153 (PI: [C3H6PO5]ˉ) and (D) m/z 241 (PI: [C6H10PO8]−) in the cell membrane as a function of modafinil concentration. Each treatment result was compared with control by a two-tailed Mann–Whitney rank-sum test, *p < 0.05, **p < 0.01 and ***p < 0.001.
These data show that modafinil induces a decrease in low-curvature lipids and an increase in high curvature lipids in all over the cell membrane. It is thought that the increase in high curvature lipids, PEs and PIs, promotes the fusion pore stabilisation during the exocytosis process due to the new configuration of the phospholipids at the exocytotic sites. Consequently, the pore can open for a longer time resulting in the elevation of the release fraction. To better understand these observations, we model the lipid structure of the membrane as presented in Scheme 1. PE has a conical shape, and its augmentation in the inner leaflet of the cell membrane induces a more favourable curvature (negative curvature) for the formation of a stable fusion pore needed for exocytosis. Furthermore, the reverse cone shape of the phosphorylated PIs induces a positive curvature needed for the final configuration of the exocytosis fusion pore. Such a favourable curvature provided by PE and PI in the cells treated with modafinil makes a stable pore that resists or delays closing, leading to prolonged t 1/2 and t fall. These effects collectively result in the enhancement of neurotransmitter release (N) by lengthening the time of release.
The observed alterations in the cell membrane lipids, induced by modafinil, are similar to those published for methylphenidate, a cognitive-enhancing drug, and opposite to those reported for cocaine, a cognition impairing drug (Phan et al., Reference Phan, Fletcher and Ewing2015; Philipsen et al., Reference Philipsen, Phan, Fletcher, Malmberg and Ewing2018). Previous work with methylphenidate showed an enhancement in the exocytotic fraction of release resembling that for modafinil shown here (Zhu et al., Reference Zhu, Gu, Dunevall, Ren, Zhou and Ewing2019). The observation that both these cognitive-enhancing drugs increase the fraction of release for exocytosis events implies this fraction is related to improved cognition. Modafinil has also been proposed as a potential treatment for cocaine abuse owing to its low abuse potential, good tolerability, positive effects on cognition and mood and different neurochemical effects (Turner et al., Reference Turner, Clark, Dowson, Robbins and Sahakian2004; Taneja et al., Reference Taneja, Haman, Shelton and Robertson2007; Martinez-Raga et al., Reference Martínez-Raga, Knecht and Cepeda2008). Cocaine abuse displays cognitive deficits in attention, working memory, declarative memory and executive functions (Vonmoos et al., Reference Vonmoos, Hulka, Preller, Jenni, Baumgartner, Stohler, Bolla and Quednow2013). The cognitive impairment, however, is persistent after long-term cocaine use (Ladron et al., Reference Ladron de Guevara-Miranda, Millon, Rosell-Valle, Perez-Fernandez, Missiroli, Serrano, Pavon, Rodriguez de Fonseca, Martinez-Losa, Alvarez-Dolado, Santin and Castilla-Ortega2017). Modafinil not only helps to reduce cocaine craving and withdrawal symptoms, but also improves the cognitive performances for cocaine-dependence treatment (Anderson et al., Reference Anderson, Reid, Li, Holmes, Shemanski, Slee, Smith, Kahn, Chiang, Vocci, Ciraulo, Dackis, Roache, Salloum, Somoza, Urschel and Elkashef2009; Kalechstein et al., Reference Kalechstein, Mahoney, Yoon, Bennett and De la Garza2013). Interestingly, modafinil’s effects on the lipid composition are opposite to the effects induced by cocaine administration (Philipsen et al., Reference Philipsen, Phan, Fletcher, Malmberg and Ewing2018). Modafinil induces a depletion in the membrane level of PCs, whereas cocaine increases PC concentration. Modafinil causes an elevation of PE and PI, whereas the levels of the same species decrease after cocaine exposure. Cocaine induces a less stable and smaller release pore due to the decrease in high curvature lipids. Modafinil enhances the number of neurotransmitters stored in the vesicles, while cocaine decreases. The possible mechanism through which the modafinil-treated cells increase their vesicle content has been discussed in the previous section by speculating modafinil’s effect on the mitochondria and cytochrome c. However, there might be an indirect effect as well for modafinil to increase the vesicular storage that needs further investigation, such as effecting the catecholamine biosynthesis pathway. This increase can be related somehow to the enhancement of the PE cellular level (Aref et al., Reference Aref, Ranjbari, Romiani and Ewing2020). Recently, Aref et al. (Reference Aref, Ranjbari, Romiani and Ewing2020) observed that intracellularly injected PE significantly (at 90% confidence interval) increases the vesicular catecholamine content in chromaffin cells. In the present work, the enhancement of PE in the cell membrane might result from intracellular PE elevation following modafinil treatment. Tasseva et al. (Reference Tasseva, Bai, Davidescu, Haromy, Michelakis and Vance2013) showed that PE contributes to mammalian mitochondrial function and ATP production. Hence, PE might promote ATP dependent activity of the VMAT-2 to increase vesicular storage. These reports are consistent with our ToF-SIMS and IVIEC results, showing the enhancement of PE following treatment with modafinil and the subsequent enhancement of vesicular storage in PC12 cells. Unlike modafinil, suppression of the PE and vesicular storage has been reported for cocaine-treated PC12 cells (Philipsen et al. Reference Philipsen, Phan, Fletcher, Malmberg and Ewing2018; Zhu et al., Reference Zhu, Gu, Dunevall, Ren, Zhou and Ewing2019). Thus, it is enticing to speculate a correlation between elevation of the PE cellular level and vesicular storage enhancement, which would explain the neuroprotective effect of modafinil by translocating cytosolic dopamine to the vesicles.
Conclusions
In summary, both amperometry and mass spectrometry imaging were used to examine the effect of modafinil as a cognition-enhancing drug at the single-cell and sub-cellular levels. The amperometric results revealed that both the quantal release and vesicular storage of catecholamines are upregulated in modafinil-treated PC12 cells. Slowing down the exocytosis dynamics induced by modafinil causes the exocytotic fusion pore to remain open for a longer time, leading to an increased number of released catecholamines and a higher fraction of release during the predominantly partial exocytosis process. This result provides valuable information about the dynamic effect of modafinil on the operation of vesicle fusion machinery, shaping our present understanding of the mechanisms underlying secretory neurotransmission in modafinil-treated cells. As exocytotic vesicular fusion with the plasma membrane is a process incorporating vesicular lipid membrane into the plasma membrane, mass spectrometry imaging was used to inspect the lipid composition alterations in the PC12 cell membrane mediated by modafinil treatment. We found modafinil decreases the low-curvature lipid, PC, in the plasma membrane while increasing the high curvature lipids, PE and PI. These findings, combined with our SCA results, suggest that the unequal head-to-tail group size of PE and PI, improves their thermodynamic fit in membrane regions of high curvature, forming a stabilised exocytotic fusion pore in modafinil-treated cells. The other possible role for PE is its intervention in mitochondrial function to increase the ATP production, resulting in vesicles with higher transmitter load. Moreover, the PE elevation and the subsequent enhancement of ATP may stiffen the cell membrane actin scaffold, increasing the exocytotic fusion pore opening time and closing time, so that the cells treated with modafinil expel a larger fraction of transmitters stored in the vesicle. These enhancements in the fraction of neurotransmitter release in the cells treated with modafinil and their vesicles loading capacity shed light on the convergence point of modafinil’s stimulant and neuroprotective effects. As phospholipids are the most abundant component of the cells that are directly involved in the exocytosis process, we suggest that the altered balance of high- to low-curvature lipid ratio induced by modafinil plays a significant role in modulating the exocytosis process, biophysically and biochemically. While the bulk of previous research has focused mostly on modafinil’s possible extracellular activities, this work by combining amperometry, electrochemical cytometry and mass spectrometry techniques provides fundamental molecular knowledge on how modafinil may modulate synaptic strength and plasticity.
Supplementary Materials
To view supplementary material for this article, please visit http://dx.doi.org/10.1017/qrd.2021.4.
Open Peer Review
To view the open peer review materials for this article, please visit http://dx.doi.org/10.1017/qrd.2021.4.
Financial support
This work was supported by the European Research Council (A.E., grant number 787534), Knut and Alice Wallenberg Foundation (A.E), and the Swedish Research Council (A.E., grant number 2017-04366), the Human Frontier Science Program Organization (E.R., grant number LT 000407/2017C).
Conflict of interest
The authors declare no conflicts of interest.
Author contributions
A.E. proposed the experiments and supervised the study. E.R. planned the experiments, performed cell culture, cell treatment, SCA and IVIEC. E.R. and Z.W. prepared samples for ToF-SIMS and performed ToF-SIMS. M.H.P., Z.W. and E.R. carried out data analysis of the ToF-SIMS. E.R., M.H.P., Z.W. and A.E. wrote and edited the manuscript.
Comments
Comments to Author: This manuscript describes the effects of the drug modafinil on exocytotic vesicle release, the amount of the neurotransmitter catecholamine molecules released by PC12 cells, and the lipid composition in the cell membrane as detected by single-cell amperometry (SCA), intracellular vesicle impact electrochemical cytometry (IVIEC), and time-of-flight secondary ion mass spectrometry (TOF-SIMS).The results show that modafinil treatment significantly increased the number of catecholamine molecules released from the PC12 cells and slowed the kinetics of vesicle fusion pore opening. The authors concluded the higher number of catecholamine molecules released after modafinil treatment was due to longer time that the fusion pore remained open. The TOF-SIMS analysis of treated and untreated cells revealed increases in phosphatidylethanolamine and phosphatidylinositol, and an increase in phosphatidyl ethanolamine.These results were then discussed in the context of possible mechanisms for the drug’s mode of action.This manuscript has many strengths and very few weaknesses (if any).The strengths include the clarity of the manuscript, the care taken in performing the study, the statistical tests are sufficient, and the interpretation of the results are especially clear. Another strength of the manuscript is the clarity of the discussion of what the results might imply about the drugs effect on the cell. The closest thing that I could identify for a weakness is that the description of the SIMS analysis left me with a few questions, which are listed below.The authors may consider addressing these questions in the manuscript.
1.The lipids that were detected in positive ion mode decreased with increasing modafinil concentration, and the lipids that were detected in negative ion mode increased with increasing modafinil concentration.Is it possible that the drug treatment enhanced the ionization of the negative ions and decreased detection of the positive ions (perhaps an ionization effect that might be caused by residual drug on the cell surface or drug-induced changes in charged molecules within the cell membrane) as opposed to changing the concentration of the parent molecule?
2.Were the changes in lipid composition detected with TOF-SIMS restricted to the site of vesicle exocytosis, or were these changes reflective of the entire cell membrane?In other words, were the TOF-SIMS analyses restricted to the sites of vesicle exocytosis?If the changes may reflect the entire cell membrane, is it possible that the changes in lipid composition that were detected occur at all sites of exocytotic vesicle release, but the longer lifetime of these exocytotic events on the drug-treated cells makes them more abundant, and thus easier to detect on the drug-treated cells?