Introduction
Radiocarbon dating indicates that farming became established in Britain during the first few centuries of the fourth millennium bc (Bayliss et al., Reference Bayliss, Healy, Whittle, Cooney, Whittle, Healy and Bayliss2011: 833–36; Bradley et al., Reference Bradley, Haselgrove and Webley2015). This change defines the onset of the British Neolithic. The transition to an agricultural subsistence regime was associated with the importation of non-native domesticated species of plants and animals from the European mainland, including sheep and cereals (Tresset & Vigne, Reference Tresset and Vigne2011: 184; Tresset, Reference Tresset, Fowler, Harding and Hofmann2015). This was accompanied by a radical transformation in both technology and culture during the early fourth millennium bc, with the introduction of new traditions into Britain from the European mainland, including pottery production and the construction of burial monuments (Scarre, Reference Scarre, Anderson-Whymark, Garrow and Sturt2015).
There has long been debate as to whether farming was introduced into Britain by the arrival of farmers from the European mainland or through the adoption of domesticates by local British hunter-gatherer communities. Recent comparative analysis of pottery suggests that new traditions and practices were introduced during the early fourth millennium bc from at least two regions of the European mainland: the Picardie/Pas de Calais area of northern France, and Brittany and Lower Normandy in north-western France (Pioffet, Reference Pioffet2015; see also Sheridan, Reference Sheridan, Finlayson and Warren2010a, Reference Sheridan, Vanmontfort, Louwe Kooijmans, Amkreutz and Verhart2010b). Analysis of ancient DNA from preserved skeletal material also indicates a substantial measure of population movement at the onset of the Neolithic throughout western Europe, including Britain (Hofmanová et al., Reference Hofmanová, Kreutzer, Hellenthal, Sell, Diekmann and Díez-del-Molino2016; Brace et al., Reference Brace, Diekmann, Booth, van Dorp, Faltyskova and Rohland2019). Here we use strontium (87Sr/86Sr) isotope and oxygen (δ18O) isotope analysis to evaluate from what region or locality certain individuals buried in Britain during the fourth millennium bc obtained their childhood diets. We compare isotope ratios in tooth enamel from ten individuals buried at the Whitwell long cairn in the East Midlands of England to mapped modern bioavailable values and to previously published data from earlier Neolithic burial populations from other areas within England and Wales. The results support the argument for the movement of individuals over long distances during the Early Neolithic. They also shed light on mobility patterns during the development of the Neolithic in Britain in the early fourth millennium bc.
Site Details
The samples in this study come from the cairn at Whitwell (Figure 1), located some 20 km north of Nottingham in central England in the county of Derbyshire (OS GB Grid SK53207482). It is one of the few Early Neolithic burial monuments in Britain to have been totally excavated to modern archaeological standards. Following its discovery during quarrying activity that had destroyed the south-eastern end of the structure, the remainder of the monument was fully excavated between 1988 and 1991 (Vyner & Wall, Reference Vyner and Wall2011). Burials were found within a linear mortuary structure and a circular cairn, both under a trapezoidal mound. The linear mortuary structure was used for the collective burial of multiple individuals. Over 900 bone fragments and disarticulated skeletal elements were recovered, representing a minimum number of sixteen individuals (twelve adults and four children) (Chamberlain & Witkin, Reference Chamberlain, Witkin, Vyner and Wall2011: 71–73). Radiocarbon dating indicates that burial activity at Whitwell began in the thirty-eighth century bc (Vyner & Wall, Reference Vyner and Wall2011). The remains of a small number of individuals, found stratified under a series of limestone slabs within the linear mortuary enclosure, have been dated to 3780–3700 cal bc (5 per cent probability; Griffiths, Reference Griffiths2011: 85; Vyner & Wall, Reference Vyner and Wall2011). One individual associated with this phase, which is thought to represent the first use of the monument for burial, had dentition that was suitable for sampling (see sample selection protocol within the Materials and Methods section below). Following the placement of the limestone slabs, the linear mortuary enclosure continued to be used for burial. Five individuals associated with this phase of use (phase 4B/5), dated to 3720–3650 cal bc (95 per cent probability; Vyner & Wall, Reference Vyner and Wall2011: 29–30), had dentition suitable for sampling. The remains of three other individuals sampled for the present study were also found within the linear mortuary structure but could not be assigned by the excavators to a specific stratigraphic context. They could have been buried either before or after placement of the limestone slabs (Table 1). Modelled radiocarbon dates, drawing on a sample of stratigraphically unrelated skeletal elements from the linear mortuary structure, suggest that these remains may date to 3760–3660 cal bc (95 per cent probability; Griffiths, Reference Griffiths2011: 85).

Figure 1. Plan of the Whitwell cairn. Reproduced with permission, after Vyner & Wall (Reference Vyner and Wall2011: fig. 5).
Table 1. Results of 87Sr/86Sr, δ18Ocarbonate and δ13Ccarbonate analysis of tooth enamel from the Whitwell cairn. All teeth are from the mandibular dentition: L = left; R = Right; M1 = permanent first molar; M2 = permanent second molar; M3 = permanent third molar; C = canine. Phasing and contexts for skeletal remains are as documented by the excavators (Vyner & Wall, Reference Vyner and Wall2011). U = stratigraphically unassociated skeletal remains excavated from the linear mortuary structure. Radiocarbon dating of a sample of stratigraphically unassociated remains from the linear mortuary structure estimates that they date to between 3760–3660 cal bc (95 per cent probability; Griffiths, Reference Griffiths2011: 85).
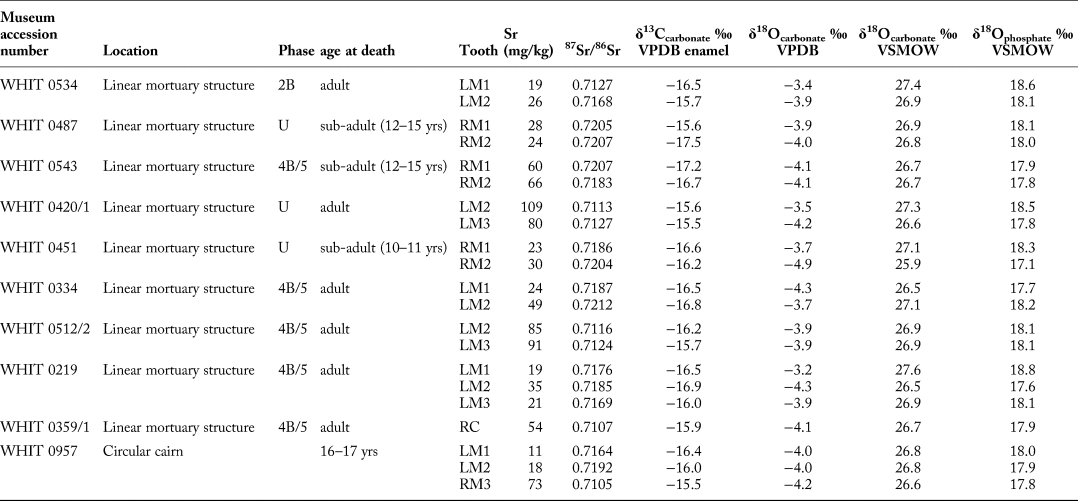
The oval cairn next to the linear mortuary structure within the long mound (Figure 1) contained the burial of a single individual, a young woman (0957) who died at approximately 16–17 years of age (Chamberlain & Witkin, Reference Chamberlain, Witkin, Vyner and Wall2011: 74–75) in 3770–3670 cal bc (95 per cent probability; Griffiths, Reference Griffiths2011: 85).
Isotope Constraints on Location and Surrounding Area
The Whitwell cairn is located in central England where the geology is dominated by Permian, Carboniferous, and Triassic-age sediments. The Permian comprises marls, sandstone, and the Cadeby Formation, on which the cairn was constructed, and these give interquartile ranges respectively of 0.7096–0.7106 (n = 4), 0.7096–0.7106 (n = 15), and 0.7087–0.7096 (n = 7). There are overlying Quaternary loess deposits in places. Biosphere samples taken on Triassic rocks generate an interquartile range of 0.7095–0.7107 (n = 55). Carboniferous-age rocks include limestone, gritstone, and coal measures. The Carboniferous limestone is well defined in terms of biosphere value, giving a plant interquartile range of 0.7090–0.7098 (n = 22). The gritstones that crop out in the Pennines give biosphere values between 0.7107 and 0.7114 (n = 11); a recent study which determined the bio-accessible Sr isotope composition from the Millstone Grit gives a value of 0.7115 (Johnson et al., Reference Johnson, Montgomery, Evans and Hamilton2019). Clays associated with coal measures give bioavailable values between 0.7095 and 0.7113 (n = 10). Taken overall, the predicted range of biosphere values for the lithologies described above would fall predominantly within the range of 0.7087 to 0.7115 (data from Evans et al., Reference Evans, Chenery, Mee, Cartwright, Lee, Marchant and Hannaford2018).
Materials and Methods
Sample selection
As the burial assemblage primarily consists of disarticulated and co-mingled human remains, care had to be taken to avoid possible duplication of results through the inadvertent sampling of mandibular and maxillary teeth that could belong to the same individual. To ensure that all samples originated from discrete individuals, only teeth which remained in situ in mandibles were used for analysis. Loose teeth and teeth from maxillary fragments were not sampled. To avoid the possibility of sampling antimeres, where mandibular dentition was incomplete and fragmentary, only teeth from left-side mandibular fragments were sampled. Teeth in right-side mandibular fragments were not analysed unless the cross-matching left-side fragment of the mandible was present.
Where possible, adjacent permanent molars, which mineralize at different stages of childhood and adolescence, were selected in order to compare isotope ratios within the enamel of teeth that form at successive stages of early life. The development of the crown of the first permanent adult molar tooth commences in utero, just prior to birth, and completes by approximately 4.5 ± 0.5 years of age, whilst the second molar crown forms between approximately 2.5 ± 0.5 years and 8.5 ± 0.5 years of age (AlQahtani et al., Reference AlQahtani, Hector and Liversidge2010; Hillson, Reference Hillson2014: 31, 55–56). The timing of human third permanent molar formation is most variable (Liversidge, Reference Liversidge2008: 313): initial cusp formation begins from approximately 8.5 ± 0.5 years of age and formation of the crown completes by approximately 14.5 ± 0.5 years (AlQahtani et al., Reference AlQahtani, Hector and Liversidge2010). In several cases, sampling of all three of the permanent molars was precluded by ante- or post-mortem tooth loss. In the case of individual 0359/1, all permanent molar teeth on both sides of the mandible had been lost ante-mortem: only one tooth, the right canine, remained in situ in the mandible, which was therefore chosen for sampling: the crown of this tooth begins to form between approximately 10.5 months and 7.5 ± 0.5 years of age (AlQahtani et al., Reference AlQahtani, Hector and Liversidge2010).
Aging and sexing
The approximate age at death of individuals was determined through visual assessment of the stage of tooth eruption and root development following AlQahtani et al. (Reference AlQahtani, Hector and Liversidge2010). Individuals with fully erupted permanent dentition are denoted as ‘adult’ in Table 1. Due to disarticulation of skeletal elements within the assemblage in the linear mortuary structure and the inability to associate mandibles with other skeletal elements such as the pelvis, the sex of most of the individuals who were sampled cannot be determined with confidence using conventional osteological methods. Only individual 0957 was found in a fully articulated state and osteological examination by Chamberlain and Witkin (Reference Chamberlain, Witkin, Vyner and Wall2011: 74–75) suggests this individual was a female who died at approximately 16–17 years of age (see above).
Methods
Teeth were processed following procedures developed by Montgomery (Reference Montgomery2002). Surface enamel was thoroughly abraded using a tungsten carbide dental burr. Enamel chips were then cut using a flexible diamond-edged rotary saw and surfaces again mechanically cleaned using a tungsten carbide dental burr to remove any adhering dentine. An enamel chip of approximately 20–30 mg in weight from each tooth was taken for strontium isotope analysis and another of approximately 10 mg in weight for oxygen isotope analysis. Dental saws and burrs were cleaned ultrasonically for five minutes and rinsed three times in high purity de-ionized water between preparation of samples.
87Sr/86Sr analysis
Samples were transferred in clean sealed containers to the Class 100, HEPA-filtered laboratory facilities at the National Environmental Isotope Facility (NEIF) (Keyworth, Nottingham, England). Enamel chips were cleaned ultrasonically and rinsed in high purity water (Millipore Alpha Q). They were then dried, weighed into pre-cleaned Teflon beakers, and spiked with a known amount of 84Sr tracer solution to obtain strontium concentrations. Each sample was dissolved in Teflon distilled 8M HNO3. Samples were converted to chloride using 6M HCl, taken up in titrated 2.5M HCl and pipetted onto ion-exchange chromatography columns. Strontium was separated with Eichrom AG50- X8 resin (200–400 mesh). Procedural blanks were below 80 pg. Samples were loaded on to Re filaments using a method adapted from Birck (Reference Birck1986). Strontium isotope composition and concentrations were then determined by Thermal Ionization Mass Spectrometry using a ThermoTriton automated multi-collector mass spectrometer. To correct for fractionation during the process of mass spectrometry, 87Sr/86Sr values are normalized to the accepted value for 88Sr/86Sr = 0.1194. During the period of this study, the machine gave a value for the international standard for 87Sr/86Sr (NBS 987) of 0.710253 ± 0.000012 (2σ, n = 350). An estimate of the reproducibility of strontium concentration (Sr ppm) is provided by replicate analysis of an aliquot of bone standard solution (NIST1486), which gave 7.22 ± 0.27 ppm (± 3.75%, 1σ, n = 16).
δ18O and δ13C analysis
Initial preparation of core enamel chips for δ18O and δ13C analysis was undertaken using the same methods employed above for strontium isotope analysis. Samples were then transferred as clean core enamel chips to the NEIF, where they were powdered. Oxygen (δ18Ocarbonate) and carbon (δ13Ccarbonate) isotope ratios in the carbonate fraction of enamel were determined using approximately 3 mg of clean powdered enamel following the method outlined in Chenery et al. (Reference Chenery, Müldner, Evans, Eckardt and Lewis2010). Isotope ratios are reported as delta (δ) values, in parts per thousand (per mil; ‰) normalized to the VPDB scale using an in-house carbonate reference material, Keyworth Carrera Marble (KCM), which is calibrated against NBS19 certified reference material. Analytical reproducibility for this run of KCM was ± 0.09‰ (1σ, n = 14) for δ18O and for δ13C ± 0.04‰ (1σ, n = 14). δ18Ocarbonate values were normalized to the VSMOW scale using the equation of Coplen et al. (Reference Coplen1988) (VSMOW = 1.03091 × δ18O VPDB + 30.91). Conversion between δ18Ocarbonate to δ18Ophosphate was then undertaken using the regression equation of Chenery et al. (Reference Chenery, Müldner, Evans, Eckardt and Lewis2010) (δ18Ophosphate =1.0322 × δ18Ocarbonate − 9.6849). The uncertainty involved in calculating δ18Ophosphate is considered to be low (0.28‰, 1σ, Chenery et al., Reference Chenery, Müldner, Evans, Eckardt and Lewis2010). All data are presented in Table 1.
Oxygen Isotope Results
Values of δ18Ocarbonate (VSMOW) of enamel from second and third permanent molars with strontium isotope ratios give a mean of 26.8 ± 0.3‰, 1σ (n = 13). First molar teeth were excluded from this calculation, since, with deciduous teeth, formation of the first molar crown begins in utero and continues during the months following birth and values may therefore be influenced by consumption of breast milk (Roberts et al., Reference Roberts, Coward, Ewing, Savage, Cole and Lucas1988: 625; Wright & Schwarcz, Reference Wright and Schwarcz1998: 14; AlQahtani et al., Reference AlQahtani, Hector and Liversidge2010; Hillson, Reference Hillson2014: 31, 55–56; Britton et al., Reference Britton, Fuller, Tütken, Mays and Richards2015: 8). However, at Whitwell the incorporation of δ18OcarbonateVSMOW values of enamel from first molars does not change the mean value and only increases the uncertainty slightly to 26.8 ± 0.4‰ (1σ, n = 21). If converted to δ18OphosphateVSMOW the full data set (n = 21) gives 18.0 ± 0.4‰, 1σ.
Oxygen isotope ratios recorded in enamel at Whitwell are comparable to those found within human archaeological populations buried in temperate regions of mainland Europe (Lightfoot & O'Connell, Reference Lightfoot and O'Connell2016). Values of δ18Ophosphate exhibited by archaeological populations buried in Britain may overlap with those found on the adjacent near continent due to climatic similarities. That precludes distinguishing between these two areas using oxygen isotope analysis alone (Lightfoot & O'Connell, Reference Lightfoot and O'Connell2016; e.g. Brettell et al., Reference Brettell, Evans, Marzinzik, Lamb and Montgomery2012a: 127; Evans et al., Reference Evans, Chenery and Montgomery2012). Such values could link these individuals either to a climate zone consistent with that of Britain, or to areas of the European mainland with similar rainwater/drinking water compositions. Recent evidence suggests that oxygen isotope ratios in human tooth enamel may also be influenced by culturally mediated behaviour, such as boiling drinking water, brewing, and stewing food (Brettell et al., Reference Brettell, Montgomery and Evans2012b). The values found at Whitwell do, however, contrast with oxygen isotope ratios found amongst human burial populations associated with regions of cooler climate in Europe (e.g. Scandinavia: Montgomery et al., Reference Montgomery, Grimes, Buckberry, Evans, Richards and Barrett2014; Chenery et al., Reference Chenery, Evans, Score, Boyle and Chenery2014; Price & Naumann, Reference Price and Naumann2015; or the Alps: Müller et al., Reference Müller, Fricke, Halliday, McCulloch and Wartho2003), where much lower values can be recorded (δ18Ocarbonate below approximately 24.5‰ or δ18Ophosphate below approximately 15.5‰).
Strontium Isotope Results
The strontium isotope results are presented in Figure 2, which shows the Sr concentrations versus 87Sr/86Sr results for tooth enamel. The data can be divided roughly into two groups. The majority of individuals (0219, 0334, 0451, 0487, 0543) have low Sr concentrations, between 11 and 66 ppm, and high 87Sr/86Sr ratios >0.7164. In contrast, three individuals (0420/1, 0359/1, 0512/2) have relatively low 87Sr/86Sr values, between 0.710 and 0.712, and have Sr concentrations ranging between 54 and 109 ppm. The remains of the latter two individuals (0359/1, 0512/2) were recovered from above the limestone slabs in the linear mortuary enclosure and are attributed to the secondary phase of use of this structure for burial (phase 4/B); whilst the remains of individual 0420/2 were not securely associated with a specific stratigraphic context within the linear mortuary enclosure and could therefore be associated with either the earliest or with a later phase of its use.

Figure 2. 87Sr/86Sr isotope composition plotted against Sr concentration (ppm) for human tooth enamel samples from the Whitwell cairn. Arrows link samples from teeth belonging to the same individual, denoting the direction of change in isotope ratio and concentration between earlier and later forming teeth. Individuals who exhibit large shifts in 87Sr/86Sr values between teeth are highlighted in black and white. A dashed line denotes the bioavailable 87Sr/86Sr range on lithologies in the immediate area around the Whitwell cairn.
The contrasting strontium isotope values exhibited by these two groups could indicate that childhood dietary resources had been derived from two contrasting terrains. Two individuals (0534 and 0957) have values that bridge the gap between the isotope compositions of the two groups, with the latter, 0957, also showing a significant change in Sr concentration between teeth. The dentition from individual 0534 (highlighted in white in Figure 2) was located below the limestone slabs in the linear mortuary structure and is therefore associated with the first phase of burial activity at this structure. Individual 0957 (highlighted in black in Figure 2) is the female burial from the oval cairn next to the linear burial structure within the monument. The shift in values between adjacent teeth exhibited by these individuals, 0534 and 0957, could be interpreted as indicative of movement during childhood between the two terrains (Figure 2).
Some constraints can be placed on these two childhood domains. The high strontium isotope ratios and low Sr concentrations that the majority of the individuals exhibit are typical of areas of ancient rock or very radiogenic rock such as granite. Thus far, such values have been recorded in human teeth from areas where such rocks crop out, in regions such as Sweden (Sjogren et al., Reference Sjogren, Price and Ahlstrom2009), India (Valentine et al., Reference Valentine, Kamenov, Kenoyer, Shinde, Mushrif-Tripathy, Otarola-Castillo and Krigbaum2015), South Africa (Copeland et al., Reference Copeland, Sponheimer, le Roux, Grimes, Lee-Thorp, de Ruiter and Richards2008), and Portugal (Waterman et al., Reference Waterman, Peate, Silva and Thomas2014). Plant samples collected in the Black Forest of south-western Germany (Bentley & Knipper, Reference Bentley and Knipper2005; Bentley, Reference Bentley2006: 145; Oelze et al., Reference Oelze, Nehlich and Richards2012) and on the Massif Central and Armorican Massif in France have also given these values (Négrel & Pauwels, Reference Négrel and Pauwels2004; Willmes et al., Reference Willmes, McMorrow, Kinsley, Armstrong, Aubert and Eggins2013, Reference Willmes, Bataille, James, Moffat, McMorrow and Kinsley2018). British bioavailable values higher than 0.717 have been recorded in plant samples taken on granites in Dartmoor in the south-west of England (Gundula Müldner, pers. comm.) and in Scotland, for example in regions of the Scottish Highlands such as the Cairngorm Mountains (Evans et al., Reference Evans, Montgomery, Wildman and Boulton2010, Reference Evans, Chenery, Mee, Cartwright, Lee, Marchant and Hannaford2018).
The lower range of values from 0.710 to 0.7127 are well within the range of Sr biosphere values found in Britain. Values around 0.710 are fully consistent with the area of the Whitwell cairn, although values above 0.7116 are harder to reconcile with the bioavailable range within the immediate vicinity of the site. Individuals with values between 0.710 to 0.7127 could therefore have sourced their childhood diet locally within Britain; however, this range of strontium values is also very common on the European mainland (Willmes et al., Reference Willmes, McMorrow, Kinsley, Armstrong, Aubert and Eggins2013; Bataille et al., Reference Bataille, von Holstein, Laffoon, Willmes, Liu and Davies2018).
Discussion
The 87Sr/86Sr vs δ18OcarbonateVSMOW for the Whitwell individuals is presented along with published data from other British earlier Neolithic populations, from Hazleton North (Gloucestershire, England), Hambledon Hill (Dorset, England), and Ty Isaf and Penywyrlod (Powys, Wales) (Figure 3). The majority of individuals at these sites have strontium isotope ratios that fall within the appropriate biosphere range for their region. This suggests that most of these individuals could have obtained their childhood diet locally within Britain (see Neil et al., Reference Neil, Evans, Montgomery and Scarre2016, Reference Neil, Montgomery, Evans, Cook and Scarre2017). In contrast, the majority of individuals sampled from the Whitwell cairn, and one individual from the Neolithic Penywyrlod long cairn (Neil et al., Reference Neil, Montgomery, Evans, Cook and Scarre2017) have 87Sr/86Sr values that are greater than 0.7170, which, according to present understanding of the methodology employed, suggests they obtained their dietary resources a significant distance from the regions in which they were buried.

Figure 3. 87Sr/86Sr vs δ18OcarbonateVSMOW comparison of dentition from earlier Neolithic sites at Hazleton North (Gloucestershire, England, Neil et al., Reference Neil, Evans, Montgomery and Scarre2016), Hambledon Hill (Dorset, England, Neil et al., Reference Neil, Evans, Montgomery and Scarre2018), Ty Isaf and Penywyrlod (Powys, Wales, Neil et al., Reference Neil, Montgomery, Evans, Cook and Scarre2017), and Whitwell (Derbyshire, England).
In considering where values higher than 0.7170 may originate, we are restricted by the amount and extent of current biosphere reference data, and so any assessment must be considered within the context of this constraint. As noted above, Dartmoor in south-west England and Scotland are among the possible source areas, but both are more than 300 km from Whitwell. Furthermore, in neither region does the appearance of Neolithic features significantly pre-date the Whitwell burials: current modelling of radiocarbon dates suggests that Neolithic material culture and practices began to appear in south-western England and Scotland in the decades around 3800 cal bc (Bayliss et al., Reference Bayliss, Healy, Whittle, Cooney, Whittle, Healy and Bayliss2011: 736, 822–24, 835–40). Bayesian modelling indicates that Neolithic practices appeared within the English Midlands, the region surrounding Whitwell, from the thirty-ninth century cal bc to the thirty-seventh century cal bc (Bayliss et al., Reference Bayliss, Healy, Whittle, Cooney, Whittle, Healy and Bayliss2011: 833; Griffiths, Reference Griffiths2011: 299–300). This suggests the Neolithic way of life may have only recently become established in central England at the time the Whitwell cairn was first used for burial (beginning at 3780–3700 cal bc 95 per cent probability; Griffiths, Reference Griffiths2011: 85, see above). Ireland does not presently seem to be a possible place of origin from the currently available biosphere data (Snoeck et al., Reference Snoeck, Pouncett, Ramsey, Meighan, Mattielli and Goderis2016, Reference Snoeck, Ryan, Pouncett, Pellegrini, Claeys and Wainwright2020). Values above 0.7170 have been recorded in Norway and Sweden (e.g. Price et al., Reference Price, Frei and Naumann2015: 112), the Alps (Müller et al., Reference Müller, Fricke, Halliday, McCulloch and Wartho2003), Portugal (Waterman et al., Reference Waterman, Peate, Silva and Thomas2014), the Massif Central in France (Willmes et al., Reference Willmes, McMorrow, Kinsley, Armstrong, Aubert and Eggins2013, Reference Willmes, Bataille, James, Moffat, McMorrow and Kinsley2018), and the Black Forest of south-western Germany (Bentley & Knipper, Reference Bentley and Knipper2005; Bentley, Reference Bentley2006: 145; Oelze et al., Reference Oelze, Nehlich and Richards2012). Most of Scandinavia, however, and possibly Portugal, may be excluded on the basis of the oxygen isotope composition and, furthermore, none of these regions have Neolithic material culture similar to that found in Britain during the early fourth millennium bc.
Biosphere 87Sr/86Sr values within the range exhibited by those buried at Whitwell have also been recorded in plants growing on the Armorican Massif within Lower Normandy and Brittany, in north-western France (Négrel & Pauwels, Reference Négrel and Pauwels2004; Willmes et al., Reference Willmes, McMorrow, Kinsley, Armstrong, Aubert and Eggins2013, Reference Willmes, Bataille, James, Moffat, McMorrow and Kinsley2018). Neolithic material culture comparable to that found in Britain is present in this region (Pioffet, Reference Pioffet2015). Similarities in material culture indicate that this was one of the areas of the European mainland from which Neolithic material culture and practices were introduced to Britain (e.g. Sheridan, Reference Sheridan, Finlayson and Warren2010a, Reference Sheridan, Vanmontfort, Louwe Kooijmans, Amkreutz and Verhart2010b; Rowley-Conwy, Reference Rowley-Conwy2011; Anderson-Wymark & Garrow, Reference Anderson-Whymark, Garrow, Anderson-Whymark, Garrow and Sturt2015: 74; Pioffet, Reference Pioffet2015).
Radiocarbon dating evidence suggests that development of farming in Britain was an asynchronous process, beginning during the first few centuries of the fourth millennium bc, with variation in both the regional timing and tempo of establishment of the Neolithic across Britain (Bayliss et al., Reference Bayliss, Healy, Whittle, Cooney, Whittle, Healy and Bayliss2011; Whittle et al., Reference Whittle, Healy and Bayliss2011; Griffiths, Reference Griffiths2011). Both the asynchronous nature of the establishment of new cultural traditions and recent study of Early Neolithic material culture found within Britain indicate that mobility patterns during the first few centuries of the fourth millennium bc were likely to have been complex. The analysis of material culture shows that Britain was linked to at least two different regions of the European mainland during the first few centuries of the fourth millennium bc, with mobility from both the Picardie/Pas de Calais area of northern France to the eastern seaboard of Britain at approximately 4000 bc, as well as from Brittany and Lower Normandy to south-western Britain from approximately 3800 bc (Pioffet, Reference Pioffet2015; see also Sheridan, Reference Sheridan, Finlayson and Warren2010a, Reference Sheridan, Vanmontfort, Louwe Kooijmans, Amkreutz and Verhart2010b). As biosphere 87Sr/86Sr values comparable to those found in enamel at Whitwell have been recorded in Lower Normandy and Brittany (Négrel & Pauwels, Reference Négrel and Pauwels2004; Willmes et al., Reference Willmes, McMorrow, Kinsley, Armstrong, Aubert and Eggins2013, Reference Willmes, Bataille, James, Moffat, McMorrow and Kinsley2018), the results could perhaps be interpreted to support archaeological arguments for mobility between these areas of the European mainland and Britain during the early fourth millennium bc.
The precise nature of that mobility remains to be established. Reiter and Frei (Reference Reiter and Frei2019: 457) have recently proposed a four-part model for human mobility patterns in prehistory: 1) non-migratory; 2) point-to-point migratory; 3) back-and-forth; and 4) repeated mobility, subdivided into 4a (cyclical mobility) and 4b (non-cyclical mobility). There is also evidence supporting larger-scale movements of people into new areas. Recent aDNA analysis, drawn from a sample of six Mesolithic and sixty-seven Neolithic individuals from England, Wales and Scotland, proposed that the Neolithic transition in Britain could be attributed predominantly to the immigration of farmers from mainland Europe, with relatively little contribution from pre-existing Mesolithic populations (Brace et al., Reference Brace, Diekmann, Booth, van Dorp, Faltyskova and Rohland2019). Domestic plants and animals too must have been brought from the European mainland, and material culture (ceramics, polished stone, funerary monumentality) also shows strong European connections (e.g. Pioffet, Reference Pioffet2015; Scarre, Reference Scarre, Anderson-Whymark, Garrow and Sturt2015; Walker, Reference Walker2018). Taken together with the aDNA evidence, this suggests that entire communities may have moved from mainland Europe during this period. That could fall within the category of point-to-point mobility, although the process was at a larger than individual scale. There is evidence of specific artefacts such as axeheads of Alpine jadeitite and Plussulien (Brittany) dolerite entering Britain at the same period; although the latter are relatively few in number and restricted to southern England, whereas the former spread northwards into Scotland (Sheridan et al., Reference Sheridan, Pailler, Pétrequin, Errera, Davis and Edmonds2011, Reference Sheridan, Pailler, Pétrequin, Cassen, Errera, Klassen, Sheridan and Pétrequin2012; Walker, Reference Walker2018). There is also a chronological distinction, the jadeitite axeheads being brought, perhaps as heirlooms, by Early Neolithic farmers (assuming they were not already circulating among the Late Mesolithic communities of Britain: Thomas, Reference Thomas2013: 280–83), whereas the Plussulien quarries may have been in active use during the earlier and middle centuries of the fourth millennium, and may indicate ongoing movement from France to Britain after the initial farming migration (Bayliss et al., Reference Bayliss, Healy, Whittle, Cooney, Whittle, Healy and Bayliss2011: 797–800). By contrast, there is very little evidence for movement in the contrary direction, from Britain to northern France, although some degree of back-and-forth mobility cannot be excluded.
Recent application of stable isotope analysis has provided evidence for movement at an individual level and has begun to elucidate patterns of mobility during the early centuries of the fourth millennium bc (Neil et al., Reference Neil, Evans, Montgomery and Scarre2016, Reference Neil, Montgomery, Evans, Cook and Scarre2017, Reference Neil, Evans, Montgomery and Scarre2018). Thus far, it is individuals buried in monuments that were in use in Britain from the thirty-eighth century bc who have been found to have the highest 87Sr/86Sr values. Individuals from the sites of Whitwell and Penywyrlod in Wales (which radiocarbon models suggest may have both been in use from the thirty-eighth century bc; Neil et al., Reference Neil, Montgomery, Evans, Cook and Scarre2017; Whittle, pers. comm.) have values consistent with mobility over long distances (greater than 300 km) during their lifetimes.
The young woman buried at Whitwell, individual 0957, who died between 3770 and 3670 cal bc (95 per cent probability, Griffiths, Reference Griffiths2011: 85), perhaps provides a good example of point-to-point migratory mobility (after the model proposed by Reiter & Frei, Reference Reiter and Frei2019). This individual was buried in the oval cairn adjacent to the linear mortuary structure (Figure 1) under the long mound at Whitwell. She exhibits a very large shift in 87Sr/86Sr values between her second and third molar teeth, from a value of 0.7192 to 0.7105 (highlighted in black in Figure 2). The strontium isotope ratio of enamel from her third molar is comparable to the local 87Sr/86Sr range in the area around Whitwell. The large change in values this individual exhibits between these two teeth could suggest that she moved a significant distance at approximately eight to nine years of age, around the time her third molar tooth began to form. She died shortly after the formation of her third molar was complete, at approximately sixteen to seventeen years of age (Chamberlain and Witkin, Reference Chamberlain, Witkin, Vyner and Wall2011: 7475).
In contrast to Whitwell and Penywyrlod, all individuals sampled from sites that radiocarbon models suggest were in use from the thirty-seventh century onward, such as Hazleton North (Gloucestershire, England), Hambledon Hill (Dorset, England), and Ty Isaf (Powys, Wales), have lower 87Sr/86Sr values, consistent with those that are more locally bioavailable. The site of Hazleton North, which modelling suggests was used for burial from the thirty-seventh century, may provide an example of repeated cyclical mobility (as defined by Reiter & Frei, Reference Reiter and Frei2019; see Meadows et al., Reference Meadows, Barclay and Bayliss2007; Neil et al., Reference Neil, Evans, Montgomery and Scarre2016). Here, 87Sr/86Sr values could be interpreted as being indicative of a routine of mobility between two different locations followed by short stays, perhaps suggesting transhumance (see Neil et al., Reference Neil, Evans, Montgomery and Scarre2016).
Recent data has, therefore, begun to provide an insight into differing patterns of mobility during the fourth millennium bc. However, in modelling mobility patterns during this period, it should be remembered that in Britain the number of sites so far sampled for 87Sr/86Sr analysis still remains small. More detailed modelling of population mobility patterns, including variation in mobility patterns over time through the fourth millennium bc, may become possible with the production of further data.
Conclusion
The majority of the individuals buried in the Whitwell cairn have strontium isotope ratios higher than 0.7170. High values are rare in human enamel in burials found in Britain (Evans et al., Reference Evans, Chenery and Montgomery2012), and all current evidence suggests that such values do not originate from the biosphere of central England (Evans et al., Reference Evans, Chenery, Mee, Cartwright, Lee, Marchant and Hannaford2018). Based on present understanding of the methodology employed and the way in which strontium isotope ratios within human enamel are derived, the results suggest that these individuals obtained the majority of their dietary resources from a geographical area in which high 87Sr/86Sr values are routinely bioavailable: for example, on granite or ancient basement gneisses. British bioavailable values higher than 0.7170 have been recorded in plants growing in Scotland and on granites in Dartmoor in south-west England. In these regions, however, the appearance of Neolithic features does not significantly pre-date the Whitwell burials: current modelling of radiocarbon dates suggests that Neolithic material culture and practices only began to appear in south-western England and Scotland in the decades around 3800 cal bc (Bayliss et al., Reference Bayliss, Healy, Whittle, Cooney, Whittle, Healy and Bayliss2011: 736, 822–24, 835–40). Strontium isotope ratios higher than 0.7170, comparable to those exhibited by the group at Whitwell, have been recorded in the biosphere on the Armorican Massif in north-western France (Négrel & Pauwels, Reference Négrel and Pauwels2004; Willmes et al., Reference Willmes, McMorrow, Kinsley, Armstrong, Aubert and Eggins2013, Reference Willmes, Bataille, James, Moffat, McMorrow and Kinsley2018). Recent analysis of Early Neolithic material culture found within Britain has been used to argue that there may have been population mobility between this area and Britain from approximately 3800 cal bc (Pioffet, Reference Pioffet2015; see also Sheridan, Reference Sheridan, Finlayson and Warren2010a, Reference Sheridan, Vanmontfort, Louwe Kooijmans, Amkreutz and Verhart2010b). Strontium isotope analysis cannot, on its own, determine the geographical source of the 87Sr/86Sr values found within human enamel at Whitwell: competing arguments for links to different source areas must, therefore, be evaluated on the basis of present archaeological and radiocarbon dating evidence. Based on current understanding of British biosphere 87Sr/86Sr data, the results do, however, provide evidence for individual human mobility over long distances during the thirty-eighth century bc, a period associated with the rapid spread of new cultural practices across Britain (Bayliss et al., Reference Bayliss, Healy, Whittle, Cooney, Whittle, Healy and Bayliss2011: 801).
Acknowledgements and Authorship
Samantha Neil and Jane Evans undertook the analysis; Samantha Neil wrote the article with contributions from Chris Scarre; all authors discussed drafts of the manuscript. Samantha Neil was funded through a Durham University Doctoral Studentship award; the strontium and oxygen isotope analysis was funded through NIGFSC grant IP-1290-0512 awarded to Janet Montgomery. Samantha Neil designed the research. The results of analysis are available in Table 1 here. We would like to thank Gundula Müldner for discussion of British 87Sr/86Sr biosphere data. We would also like to thank Hilary Sloane (National Environmental Isotope Facility) for analytical support, Andrew Chamberlain, Blaise Vyner, Ian Wall, Maria Smith, and Creswell Crags Museum for access to collections and for permission to undertake sampling.