1. Introduction
Climate change is causing extreme conditions in coastal communities including (but not limited to) sea level rise, flooding, and an increase in the frequency and severity of storms. Communities that do not have the capacity and are not resilient to these threats are facing the worst crisis. Hence, there is heightened interest in building resilient coastal communities to withstand the threats of growing climate change. Although there is no universal definition of resilience within the field of community planning, resilience planning often includes vulnerability identification and subsequent planning to build capacity to respond to and recover from predicted threats (Desouza & Flanery, Reference Desouza and Flanery2013; Lu & Stead, Reference Lu and Stead2013). There is also no agreed upon method for operationalizing resilience as each system is unique and, therefore, requires a unique approach. For example, resilience is used differently within multiple contexts including community resilience (Berkes & Ross, Reference Berkes and Ross2013; Norris et al., Reference Norris, Stevens, Pfefferbaum, Wyche and Pfefferbaum2008), urban resilience (Meerow et al., Reference Meerow, Newell and Stults2016), infrastructure resilience (Curt & Tacnet, Reference Curt and Tacnet2018), ecological resilience (Adger, Reference Adger2000), and social resilience (Adger, Reference Adger2000; Davidson, Reference Davidson2010).
Food, energy, and water systems are integral pillars of coastal infrastructure and essential for human well-being. These systems each have many individual components that may be compromised due to climate emergencies. For example, the food system involves production, processing, packaging, distribution, retail, and consumption (Tendall et al., Reference Tendall, Joerin, Kopainsky, Edwards, Shreck, Le, Kruetli, Grant and Six2015). Climate change and climate emergencies can impact food security by directly reducing crop production, food supply/delivery, and access (Bizikova et al., Reference Bizikova, Tyler, Moench, Keller and Echeverria2016). Energy systems may be impacted by extreme temperature, storms, and floods which pose damage to transmission lines and substation equipment (Panteli & Mancarella, Reference Panteli and Mancarella2015). Similarly, water and sanitation systems are at risk from climate-related hazards, including drought, flooding, wind, and saline intrusion (Luh et al., Reference Luh, Royster, Sebastian, Ojomo and Bartram2017). Coastal communities are at increased risk to groundwater infiltration and salinization which may result in corrosion of pipes, loss of hydraulics, and pumping pressure (Allen et al., Reference Allen, Crawford, Montz, Whitehead, Lovelace, Hanks, Christensen and Kearney2019).
The nexus of food, energy, and water systems (FEW nexus or ‘nexus’) is increasingly advocated as its own unit of analysis as the three systems are heavily interconnected (Cairns & Krzywoszynska, Reference Cairns and Krzywoszynska2016; Endo et al., Reference Endo, Tsurita, Burnett and Orencio2015; Gallagher et al., Reference Gallagher, Dalton, Bréthaut, Allan, Bellfield, Crilly, Cross, Gyawali, Klein, Laine, LeFlaive, Li, Lipponen, Matthews, Orr, Pittock, Ringler, Smith, Tickner and Vuille2016; Howarth & Monasterolo, Reference Howarth and Monasterolo2016). Specifically, there is a growing body of research dedicated to identifying and understanding the implications of the interdependencies of critical infrastructure (Choi et al., Reference Choi, Dooley and Rungtusanatham2001; Chtioui et al., Reference Chtioui, Baina and Bellafkih2016; Nan & Sansavini, Reference Nan and Sansavini2017). Critical infrastructure refers to the systems relied on to support day-to-day life, such as, the energy grid, food distribution, or water treatment plants. As technology and society have advanced, the resilience of the critical systems depends upon understanding the integrated and interconnected nature of these systems (Nan & Sansavini, Reference Nan and Sansavini2017). System interdependence means that a disruptive event in one critical infrastructure system can induce devastating consequences, or subsequent failure, within each system dependent on it.
The resilience of food (Tendall et al., Reference Tendall, Joerin, Kopainsky, Edwards, Shreck, Le, Kruetli, Grant and Six2015), energy (Burkhard & Gee, Reference Burkhard and Gee2012; Panteli & Mancarella, Reference Panteli and Mancarella2015; Roege et al., Reference Roege, Collier, Mancillas, McDonagh and Linkov2014), and water systems (Diao et al., Reference Diao, Sweetapple, Farmani, Fu, Ward and Butler2016; Gonzales & Ajami, Reference Gonzales and Ajami2017; Juan-García et al., Reference Juan-García, Butler, Comas, Darch, Sweetapple, Thornton and Corominas2017; Luh et al., Reference Luh, Royster, Sebastian, Ojomo and Bartram2017) have individually been studied. However, resilience of the FEW nexus within the coastal context has been less examined (de Grenade et al., Reference de Grenade, House-Peters, Scott, Thapa, Mills-Novoa, Gerlak and Verbist2016). Therefore, this study implemented a systematic literature review to better understand how food, energy, and water systems are integrated into coastal resilience research. The key research question was: How has the food, energy, and water nexus been integrated into coastal resilience?
2. Methods
A systematic literature review to identify papers focused on coastal resilience that incorporated the nexus between food, energy, and water systems was performed initially in May 2020 and repeated in March 2021. The search terms were chosen to reflect the broad variety of terminology (Table 1) that is used to describe food, energy, and water systems, their nexus, coastal communities or systems, and resilience. Food, energy, and water systems are sometimes referred to as ‘critical infrastructure’ (e.g., systems that are essential for a community to function) and the ‘food–energy–water nexus’ is sometimes referred to as ‘critical infrastructure interdependencies’. The terms resilience, adaptation, sustainability, management, planning, mitigation, policy, governance, regulation, and disaster risk reduction were used to search for studies in the context of coastal resilience. Terms marked in Table 1 with an asterisk mean that any multiples of that root word were included in the search (e.g., adapt* would include adapt, adapted, and adaptation in the search).
Table 1. Key search terms

The search terms detailed in Table 1 were used to create a Boolean search in the Advanced Search portal from the Web of Science. No limit was placed on when the studies were published, but they were restricted to peer-reviewed journal articles written in English.
The search yielded 205 papers from Web of Science that were published between 1988 and 2021. The search results were then reviewed in two stages. First, the titles and abstracts were reviewed to select preliminary matches of the criteria listed below, and then a full-text search was performed. This resulted in narrowing the pool of papers ultimately analyzed to address the research question. This two-stage search process will be referred to as the title and abstract search and the full-text search.
2.1 Title and abstract search
To determine how the food–energy–water nexus has been incorporated into coastal resilience research, the search terms and inclusion criteria were intentionally designed to cast a wide net. Contents of the abstract or title were required to meet the criteria defined in Table 2 for the paper to be selected for analysis. Specifically, criteria from both the ‘Food, Energy, Water’ and ‘Resilience’ categories (Table 2) had to be met in the title and/or abstract for the paper to be analyzed further. If specific systems were mentioned, then food, energy, and water must have been included, otherwise the paper was excluded (e.g., if only energy and water were mentioned, the study was excluded as it did not discuss food). The paper was included if the study only mentioned ‘critical infrastructure’ so that the full-text could be searched.
Table 2. Categories of inclusion criteria for the title and abstract search
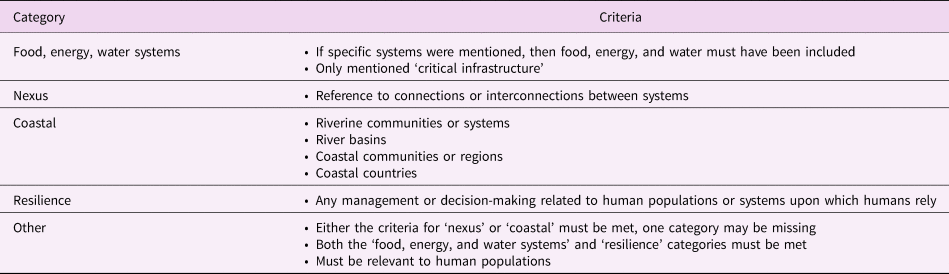
Although not specified in the search terms, the criteria for ‘coastal’ was loosened to include studies of riverine systems, river basins, as well as coastal regions and countries. A riverine focus was allowed at this stage of analysis because these regions and communities are also near bodies of water and can face similar challenges as coastal communities along the ocean or seas. The term resilience has many different definitions and is applied in a variety of ways, so the criterion describing ‘resilience’ was broadened to include any sort of management or decision-making related to human populations or systems upon which humans rely. One keyword from either the ‘nexus’ category or ‘coastal’ category (Table 1) had to be present, but one may have been excluded at this level of analysis. If no keywords from the ‘coastal’ or ‘nexus’ category were included in the title or abstract, the paper was excluded from further analysis.
Finally, the article had to be relevant to human populations (i.e., not a study of an animal or other species). Papers with a sole focus on engineering resilience were not included as the term tends to be used differently, unless it was in the context of management.
Fifty-four papers met all the criteria of the title and abstract and their PDFs were collected for use in the full-text search.
2.2 Full-text search
Each of the 54 articles selected during the title and abstract search were then manually analyzed during the full-text search. Papers were included or excluded based on the criteria for including ‘food, energy, and water systems’, ‘nexus’, ‘coastal’, and ‘resilience’.
2.2.1 Food, energy, and water systems
Papers had to incorporate food, energy, and water systems into their methods, results, and discussions or be included as more than a buzzword. Inclusion of a term as a buzzword meant that the food–energy–water nexus was only used once or twice, often in the introduction and one line in the discussion or conclusion section. A ‘mention’ was defined as the inclusion of a specific term or system one to three times throughout an entire paper without meaningful discussion. Papers were excluded if the critical infrastructure referred to in the abstract of the study only referred to coastal protection measures (e.g., seawalls).
2.2.2 Nexus
The study was considered for further analysis if it incorporated a discussion or consideration of the interconnections between the food, energy, and water systems, either directly or indirectly. Directly meant that the nexus and interconnections among its components were analyzed as a central part of the study. Indirectly meant that the study did not have a nexus focus but happened to include a discussion of how two or more systems were connected, or may have impacted one another.
2.2.3 Coastal
Papers were included if they focused on a coastal system, population, or community, or if the study was of a coastal area or coastal hazard (e.g., flood event). Studies were initially included if they focused on a riverine system or river basin, but were later excluded during the full-text analysis if they did not also consider an acute hazard (e.g., a flood event). Studies were included if they focused on an entire country (e.g., Australia), but were later excluded during the full-text analysis if the focus was not on the coast or a coastal hazard.
2.2.4 Resilience
Papers were included if they incorporated a resilience perspective, which, in this case, means they considered how to make better decisions or manage a particular system for the benefit of human populations. This perspective was considered if the paper was from a resilience, adaptation, planning, mitigation, or policy perspective (to name a few). This means that the studies did not need to be only in the context of a hazard (chronic or acute), they just needed to seek to increase self-reliance or persistence in the face of any challenge relevant to a coastal area. This is supported by studies of resilience which proposes several classifications of resilience; resilience as resistance, incremental adjustment, and transformation (Matyas & Pelling, Reference Matyas and Pelling2015), which can include adaptation, sustainability, and other related concepts within a broader definition of resilience.
Fifty-four studies were included in the full-text search. After this full-text search, 34 studies were eliminated and twenty studies were found to meet all of the search criteria (see Appendix A for a list of the 20 studies). Of the 34 studies that were eliminated, 11 had only passing mentions of, or did not include, one or more of the food, energy, or water systems in the methodology or analysis (Abdalla et al., Reference Abdalla, Tao, Cheng and Li2007; Anderson et al., Reference Anderson, Hotchkiss, Myers, Stout, Grue, Gilroy, Aldred and Rits2020; Fekete, Reference Fekete2019; Gurdak et al., Reference Gurdak, Geyer, Nanus, Taniguchi and Corona2017; Little et al., Reference Little, Loggins and Wallace2015; Montgomery et al., Reference Montgomery, Broyd, Cornell, Pearce, Pocock and Young2012; Saladini et al., Reference Saladini, Betti, Ferragina, Bouraoui, Cupertino, Canitano, Gigliotti, Autino, Pulselli, Riccaboni, Bidoglio and Bastianoni2018; Samimi et al., Reference Samimi, Ebrahimnejad and Mojtahedi2020; Veijalainen et al., Reference Veijalainen, Ahopelto, Marttunen, Jääskeläinen, Britschgi, Orvomaa, Belinskij and Keskinen2019; Wang, Reference Wang2013; Zimmerman et al., Reference Zimmerman, Foster, González, Jacob, Kunreuther, Petkova and Tollerson2019). Thirteen were eliminated because they were of riverine locations which did not consider any acute hazards (Bekchanov & Lamers, Reference Bekchanov and Lamers2016; Dombrowsky & Hensengerth, Reference Dombrowsky and Hensengerth2018; Intralawan et al., Reference Intralawan, Wood, Frankel, Costanza and Kubiszewski2018; Jalilov et al., Reference Jalilov, Amer and Ward2018; Keskinen et al., Reference Keskinen, Someth, Salmivaara and Kummu2015; Kleinschroth et al., Reference Kleinschroth, Winton, Calamita, Niggemann, Botter, Wehrli and Ghazoul2021; Mamatova et al., Reference Mamatova, Ibrokhimov and Dewulf2016; Martin-Gorriz et al., Reference Martin-Gorriz, Soto-García and Martínez-Alvarez2014; Melloni et al., Reference Melloni, Turetta, Bonatti and Sieber2020; Payet-Burin et al., Reference Payet-Burin, Kromann, Pereira-Cardenal, Strzepek and Bauer-Gottwein2019; Rasul et al., Reference Rasul, Neupane, Hussain and Pasakhala2019; Shi et al., Reference Shi, Luo, Zheng, Chen, Bai, Liu, Ochege and De Maeyer2020; Zeng et al., Reference Zeng, Zhang, Yu, Zhu, Li and Tang2019). Five studies were eliminated because they focused on entire countries or regions and were not of a fine enough scale to provide useful insight into coastal resilience issues, management, or planning (DeLonge & Basche, Reference DeLonge and Basche2017; Melo et al., Reference Melo, Parry, Brancalion, Pinto, Freitas, Manhães, Meli, Ganade and Chazdon2021; Saidmamatov et al., Reference Saidmamatov, Rudenko, Pfister and Koziel2020; Taniguchi et al., Reference Taniguchi, Masuhara and Burnett2017; Zeng et al., Reference Zeng, Cai, Ringler and Zhu2017). One study was eliminated because it was of an inland floodplain but did not incorporate any riverine or flooding considerations (Fan et al., Reference Fan, Zhang, Chen and Chen2020). One study only considered food, energy, and water systems from a health perspective (i.e., food-borne illness, water contamination, and energy-related heat illness/death) and did not consider food–energy–water systems interconnections (Bambrick et al., Reference Bambrick, Capon, Barnett, Beaty and Burton2011). One study presented a hypothetical model and then mentioned food–energy–water systems in the case study application, but chose to focus on transportation and education infrastructure (Cheng, Reference Cheng2017). One study included short sections each on coastal, food, energy, and water but they were not the focus of the paper and their interconnections were not emphasized (Uchiyama et al., Reference Uchiyama, Stevenson and Tandoko2020). The last study to be eliminated had one mention each of hydropower and farming/fishing for food security, but did not discuss them specifically in the context of the power and governance perspective of their study (Bréthaut et al., Reference Bréthaut, Gallagher, Dalton and Allouche2019).
2.3 Data analysis
Once the sample of papers to be analyzed was determined following the full-text search, the following information was extracted from each study: article date; journal; keywords; geographic location of the study; any hazards considered; the research question of the study (if specified); the goal or objective of the study (if specified); how each of the food, energy, and water systems were included; other systems or perspectives that were included; how the nexus was defined or included; what the resilience perspective was; and if there were any recommendations or broad conclusions from the study.
3. Results
The 20 papers that met the search criteria offer insights into the location and hazards considered, how the food–energy–water nexus was conceptualized, the benefits of nexus approaches and other methodologies, perspectives on community resilience from extreme events in urban areas, and other systems that were included in addition to the food–energy–water nexus (e.g., transportation).
3.1 Summary of locations and hazards
The 20 studies that met the search criteria were all published recently, considered predominantly acute hazards, and focused on locations from around the world. The studies examined were conducted between 2016 and 2021. The oldest study that met the search criteria was from 2016, indicating that the incorporation of food–energy–water systems within the study of coastal resilience is relatively new. Most of the studies (14 of 20) focused on acute hazards from extreme climate events (i.e., short-term, extreme events), three considered other challenges (e.g., coastal resource use and heavy metal pollution), and three studies considered chronic hazards (i.e., long-term hazards) (Table 3). Most of the studies focused on urban locations (13 of 20), four considered both rural and urban areas, and three considered purely rural locations (i.e., Cordova, Alaska, the Chamela-Cuixmala Biosphere Reserve, Mexico, and Lao PDR). Eight of the studies were situated in the USA, two were of hypothetical communities, two were island communities (i.e., Puerto Rico and Zanzibar), the rest were situated in the Taipei metropolitan area, a biosphere reserve in Mexico, the Persian Gulf, Khartoum, Sudan, and Lao People's Democratic Republic (PDR). Some studies looked into multiple cities, such as one jointly of Manila, The Philippines and Rotterdam, The Netherlands. Another study examined 16 coastal cities in China and another used many examples from around the globe.
Table 3. Summary of the studies categorized by kind of location and the hazard considered by each
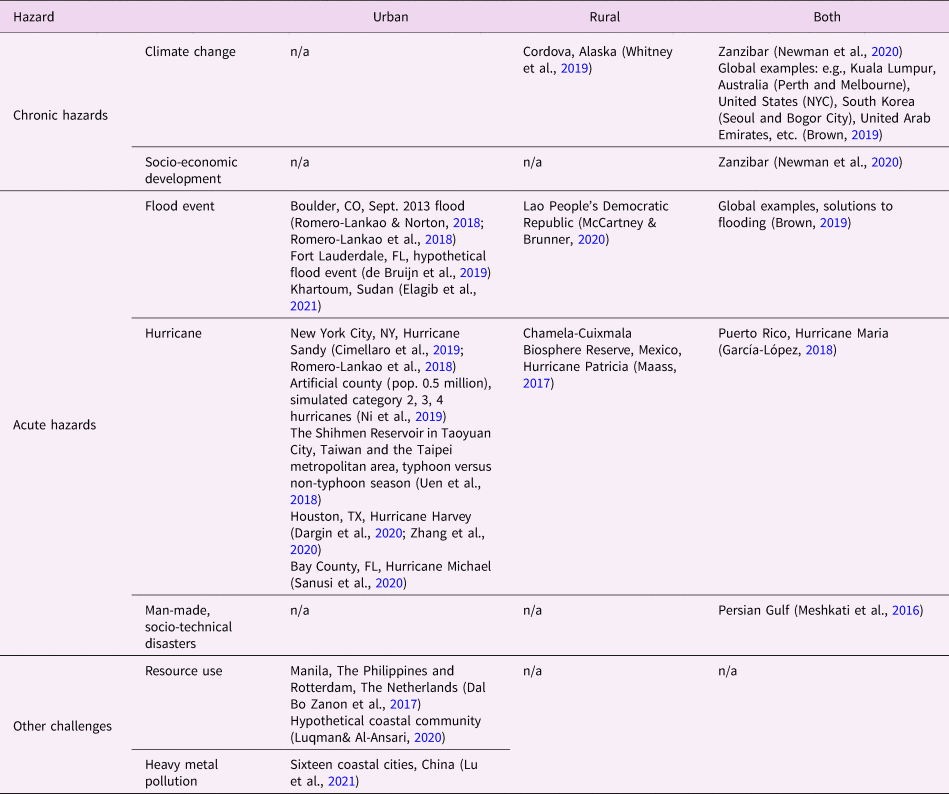
Multiple hazards were considered in several studies.
The authors of the studies were primarily affiliated with universities and organizations from the United States and Europe (62 of 79 authors, 78%) and the dominant field was engineering (36 of 79 authors, 46%). The remaining authors were affiliated with Africa, Qatar, South Korea, Taiwan, China, and Mexico and the second most common field affiliation was environment/natural science (25 of 79 authors, 32%).
3.2 Food–energy–water nexus considerations
In urban areas and in regions that contained urban areas (17 of 20), the food–energy–water systems were mostly (10 of 17 studies) considered in terms of water and wastewater treatment facilities, the electric grid (utilities) and generators, and supermarkets and food retailers (critical commercial services). These studies identified the centrality of energy systems, the need for electricity to pump and treat water in water treatment facilities, the need for energy for the refrigeration systems for food retailers and the energy and water inputs required to operate facilities (Cimellaro et al., Reference Cimellaro, Crupi, Kim and Agrawal2019; de Bruijn et al., Reference de Bruijn, Maran, Zygnerski, Jurado, Burzel, Jeuken and Obeysekera2019; Ni et al., Reference Ni, Little, Sharkey and Wallace2019; Romero-Lankao & Norton, Reference Romero-Lankao and Norton2018; Romero-Lankao et al., Reference Romero-Lankao, Bruns and Wiegleb2018). One study in Cordova, Alaska, a small coastal town with population less than 2500, considered the FEW nexus similar to the urban studies with a fish-processing plant and a hydroelectric facility along with power utility, food security, water utility, and a wastewater treatment plant (Whitney et al., Reference Whitney, Schnabel, Aggarwal, Huang, Wies, Karenzi, Huntington, Schmidt and Dotson2019).
Although a majority of the studies considered the food–energy–water nexus in a similar manner (i.e., in terms of the reliance across these systems that they be maintained to ensure uninterrupted services so they can provide resources needed to sustain human needs), there were also some outlier conceptualizations. For example, the FEW nexus was considered in terms of hydropower in the Syr Darya River Basin where the food–energy–water–ecology nexus was coupled with an optimization model (Uen et al., Reference Uen, Chang, Zhou and Tsai2018). The nexus tradeoffs were centered around water use for either energy generation or water use for irrigated agriculture. This study explored the impact on FEW systems between typhoon and non-typhoon season and water availability and short-term versus long-term reservoir operation. Here, the authors recommended that an optimization model can help develop governance strategies that are feasible and socially acceptable in the food–energy–water nexus (Uen et al., Reference Uen, Chang, Zhou and Tsai2018). Another study in the Persian Gulf conceptualized the food–energy–water nexus in terms of the connections between nuclear power, seawater desalinization, and seafood resources (Meshkati et al., Reference Meshkati, Tabibzadeh, Farshid, Rahimi and Alhanaee2016). Seawater desalination produces brine, which can impact seafood resources that communities rely on. Nuclear power could accidentally release radioactively contaminated water or cause a nuclear accident, which would negatively impact water and seafood resources that rely on the water.
The nexus was also considered in terms of modeling the interdependencies between critical infrastructure systems. In these studies, the food–energy–water nexus was not directly targeted, but the interactions between food, energy, and water systems were found to be significant, regardless. For example, a multi-commodity disruption model and restoration model was used to better examine interdependencies between infrastructure systems (infrastructures: power, water, wastewater, telecommunications, and transportation; emergency services: police, firefighters, and emergency medical services; and critical commercial services: cash, fuel, pharmaceuticals, and basic food) during simulated hurricanes (Ni et al., Reference Ni, Little, Sharkey and Wallace2019). This model suggested that well-coordinated restoration decisions can help to improve community resilience, especially with category 4 and 5 hurricanes (Ni et al., Reference Ni, Little, Sharkey and Wallace2019). An Interoperability Input–Output model designed for New York City found the utilities, liquid fuel, and transportation systems to be the most important systems in the network during hurricanes as they caused the most indirect impact to other systems (i.e., food, liquid fuel, healthcare, telecommunication, transportation, water and wastewater, and waste) (Cimellaro et al., Reference Cimellaro, Crupi, Kim and Agrawal2019). This study recommended prioritizing initiatives that reduced the linkages between energy, liquid fuel, transportation, and other systems. To demonstrate this, they cited examples from the New York City resilience plan, such as providing backup generators to gas stations, protecting tunnels from flooding, and developing a fuel infrastructure hardening program (Cimellaro et al., Reference Cimellaro, Crupi, Kim and Agrawal2019).
3.3 Nexus approaches and other methodologies
The food–energy–water nexus was incorporated within the 20 studies in two ways: a direct nexus approach was used as part of the methodology, or the interconnections between food, energy, and water systems were revealed despite not directly targeting them in the methodology. A food–energy–water nexus approach (or similar types of approaches) was directly used and advocated for as a methodology in half of the studies (11 of 20). Intentional use of a food–energy–water nexus approach meant that the study was designed to purposely investigate food, energy, and water systems and their interconnections within the context of a particular study location or broader research project. Results suggest that a nexus approach can ensure that the key tradeoffs are considered in resilience research and planning. For example, the food–energy–water nexus approach study in Cordova, Alaska highlighted key tradeoffs between water for hydroelectric energy generation, water for use in a fish-processing plant, and water for the municipal water system, which may have otherwise been overlooked (Whitney et al., Reference Whitney, Schnabel, Aggarwal, Huang, Wies, Karenzi, Huntington, Schmidt and Dotson2019). This study found that ‘incorporating FEW interdependencies with this approach can lead to decisions that are more consistent with FEW security and community well-being’ (Whitney et al., Reference Whitney, Schnabel, Aggarwal, Huang, Wies, Karenzi, Huntington, Schmidt and Dotson2019, p. 849).
Many groups are consulted during a resilience planning process, so it is important to consider diverse perspectives and systems. When planning for resilience, a food–energy–water nexus approach can ensure a more holistic perspective of the community by ensuring that multiple groups, systems, and their interconnections are included. Dynamic Environmental Livelihood Sustainability (DESL) is a conceptual framework, which works to incorporate the community perspective into food–energy–water nexus thinking (Newman et al., Reference Newman, Capitani, Courtney-Mustaphi, Thorn, Kariuki, Enns and Marchant2020). This study reports that nexus thinking is needed to better understand the many interconnected systems and how they impact local communities. There are many other factors outside of food–energy–water systems that can impact security in the face of hazards. As such, two studies advocate for using a framework that integrates the connections between the socio-demographic, economic, technological, environmental, and governance (SETEG) domains (Romero-Lankao & Norton, Reference Romero-Lankao and Norton2018; Romero-Lankao et al., Reference Romero-Lankao, Bruns and Wiegleb2018). Considering SETEG domains and their interdependencies allows cities to ‘begin to anticipate and avoid cascading effects on WEF [water–energy–food] systems and resources’ (Romero-Lankao et al., Reference Romero-Lankao, Bruns and Wiegleb2018, p. 221).
A nexus approach can act as a starting framework that allows the researcher or planner to consider additional linkages with other systems, such as considering the connections between the systems that support human life and the natural world they rely on. The socio-ecosystem (SES) approach was advocated for to guide food–energy–water research as it links ecosystem supporting services (e.g., energy, nutrient, and water processes) with provisioning services (e.g., food, charcoal, and clean water) (Maass, Reference Maass2017). The ‘infrastructural ecology’ planning paradigm embraces the principles of natural ecosystems (Brown, Reference Brown2019). Infrastructural ecology is when human systems are designed to work together just as ecological systems do, by sharing structures or space to produce efficiencies.
Strategies for how a food–energy–water nexus approach can be implemented to help increase coastal resilience were incorporated in four studies (Table 4). Numerous examples were provided from all over the world to demonstrate an infrastructural ecology approach to show how power, water, sanitation, transport, and food systems can be combined or linked (Brown, Reference Brown2019). Combining systems offers advantages such as, passing along waste, water, or nutrients for use in another system, combining resources and reducing demand for additional inputs thereby creating efficiencies. Redundant systems are important due to the cascading impacts that damage to one FEW system can have on the other (so that if one was damaged there was a redundant system available to keep operations running), such as was highlighted during the 2013 flood in Boulder, CO (Romero-Lankao & Norton, Reference Romero-Lankao and Norton2018). They highlight that since the city had backup generators (energy) they were able to keep the water treatment plant functional even though the broader energy system had been damaged by the flood (Romero-Lankao & Norton, Reference Romero-Lankao and Norton2018).
Table 4. Strategies that leverage nexus principles in coastal areas as highlighted by four studies
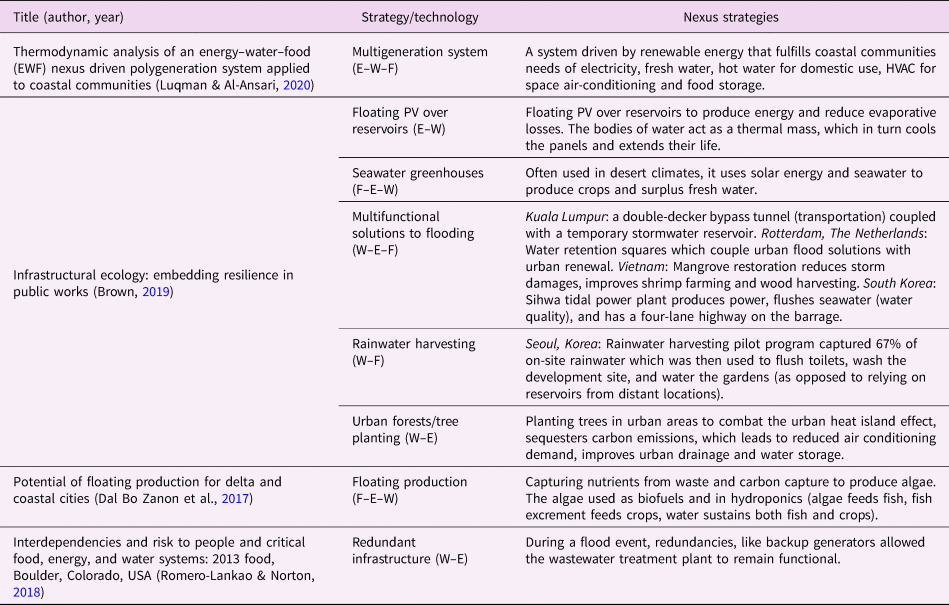
As urban areas have high-population densities, they rely on surrounding areas, even global supply chains (Heard et al., Reference Heard, Miller, Liang and Xu2017), for the provision of food, energy, and water resources. A multigeneration system (Luqman & Al-Ansari, Reference Luqman and Al-Ansari2020) and floating production (Dal Bo Zanon et al., Reference Dal Bo Zanon, Roeffen, Czapiewska, de Graaf-Van Dinther and Mooij2017) were proposed to decrease the dependence of coastal cities on surrounding areas by capitalizing on the linked nature of food–energy–water systems. The multigeneration system presented by Luqman and Al-Ansari (Reference Luqman and Al-Ansari2020) capitalized on the interconnections between systems to create one compact system that generates energy, fresh cold water and hot water for domestic use, heating, ventilation, and air conditioning (HVAC) for space air conditioning, and food storage for coastal communities. Floating production is a technique to reduce coastal cities' dependence on surrounding areas by capturing waste and using it as an input for hydroponic systems. These hydroponic systems use waste as nutrient input to grow algae, the algae feed fish and can be used to produce biofuel, fish excrement provides nutrients to feed crops and both the crops and fish are used as a food source for coastal populations (Dal Bo Zanon et al., Reference Dal Bo Zanon, Roeffen, Czapiewska, de Graaf-Van Dinther and Mooij2017).
3.4 Acute events in urban areas
Flood events and hurricanes (acute hazards) were the primary connecting points across the food–energy–water nexus in the majority (13 of 17) of urban areas (and regions that contained urban areas) studied. In these studies, the importance of the energy and transportation systems was particularly evident. For instance, losing power during a hurricane and flood event impacted all the other critical infrastructure systems in studies focused on New York City and Fort Lauderdale, FL (Cimellaro et al., Reference Cimellaro, Crupi, Kim and Agrawal2019; de Bruijn et al., Reference de Bruijn, Maran, Zygnerski, Jurado, Burzel, Jeuken and Obeysekera2019). Furthermore, the lack of power created health issues via its impact on food and water systems. Specifically, loss of power for refrigeration and water sanitation increased the risk of waterborne and foodborne illness in a study of the 2013 flood in Boulder, CO (Romero-Lankao & Norton, Reference Romero-Lankao, Bruns and Wiegleb2018). Figure 1 shows that the energy system impacted every other system it was connected to.
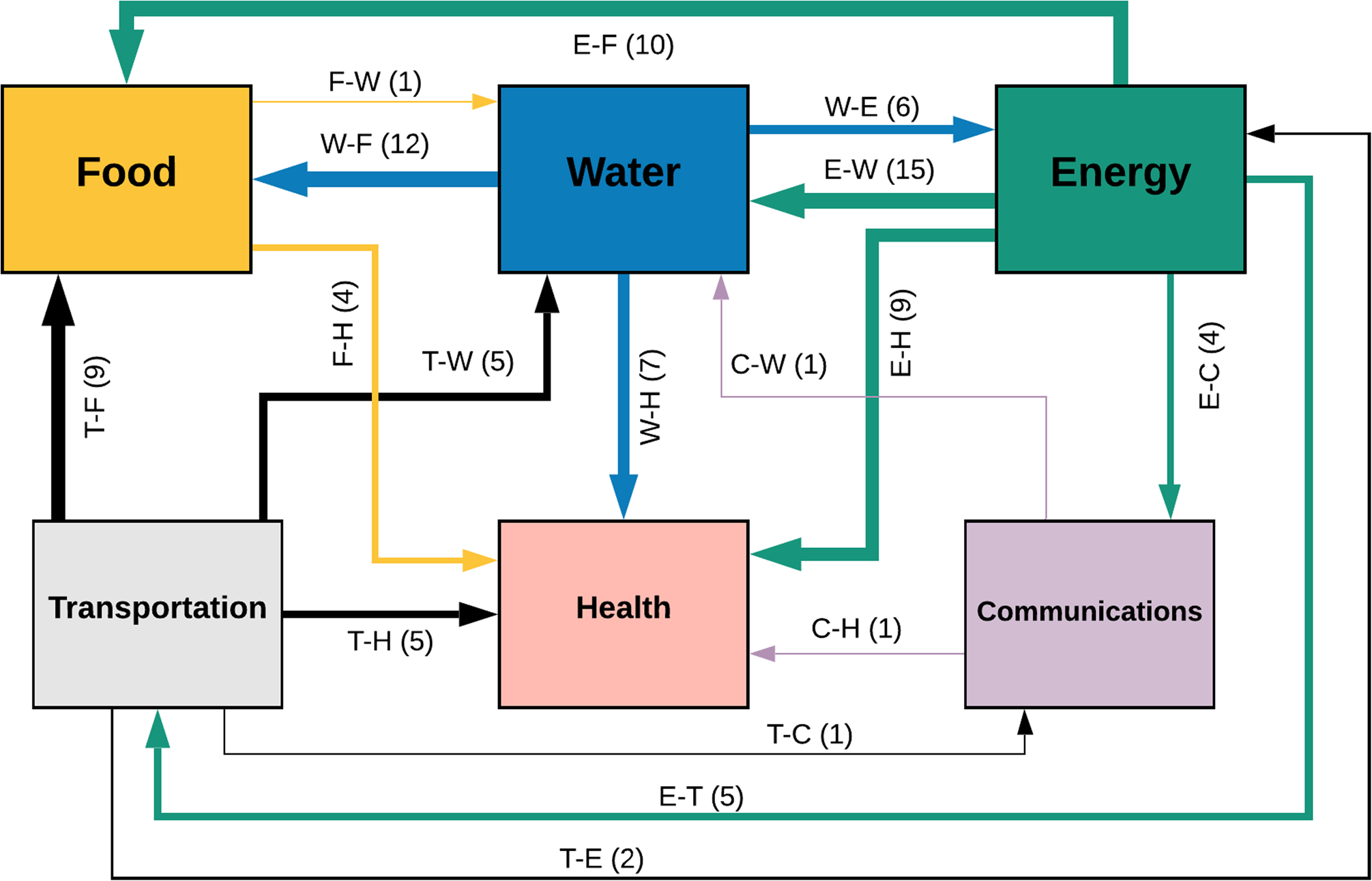
Fig. 1. Connections between systems highlighted in each of the 20 studies. Each system is represented by a box and the directional lines between systems show connections. For example, a line from energy to water means that water was described as being dependent on the energy system. Line weight and the numbers in parentheses indicate the number of studies that described a connection between systems.
Results revealed that damage to the transportation system negatively impacted many other systems, primarily the food system (Figure 1). Disruptions to the transportation system during the 2013 flood in Boulder, CO, amplified the flood impacts (Romero-Lankao & Norton, Reference Romero-Lankao, Bruns and Wiegleb2018). Critical commercial services, such as food, are heavily linked to the transportation system (Ni et al., Reference Ni, Little, Sharkey and Wallace2019). Urban areas are heavily reliant on global food supply chains, for example, New York City's food is transported by truck and depends on transportation infrastructure (Romero-Lankao et al., Reference Romero-Lankao, Bruns and Wiegleb2018). There was a food shortage crisis that resulted from Hurricane Maria in Puerto Rico (García-López, Reference García-López2018). Many could not access supermarkets due to blocked roads and, since the island relies on imported food, they suffered from a delayed delivery of food and other aid. Commercial food facilities were found to keep small inventories, which exacerbated the challenges when new supplies were limited due to disruptions to the transportation system (Romero-Lankao et al., Reference Romero-Lankao, Bruns and Wiegleb2018). It is recommended that retailers increase their inventory when a category 3 hurricane is predicted (Ni et al., Reference Ni, Little, Sharkey and Wallace2019).
Many studies note the cascading impacts that damage to both the energy and transportation systems could have on other systems (Figure 1). A study conducted in Fort Lauderdale, FL, classified the food system as a commercial facility (e.g., supermarkets), and the study found that during a flood event disruptions to power, transportation, and financial services resulted in a lack of food supplies (de Bruijn et al., Reference de Bruijn, Maran, Zygnerski, Jurado, Burzel, Jeuken and Obeysekera2019). During Hurricane Sandy, direct damage to the food system included physical damages to the facilities, indirect damages to operations due to electrical outages, lack of access to water, and transportation in New York City (Cimellaro et al., Reference Cimellaro, Crupi, Kim and Agrawal2019). Food suppliers (i.e., supermarkets, warehouses, and restaurants) in NYC were impacted by the shutdown of the transportation system and power outages after Hurricane Sandy (Romero-Lankao et al., Reference Romero-Lankao, Bruns and Wiegleb2018). A study of the 2013 flood in Boulder, CO highlighted the links between food, transportation, and power systems (Romero-Lankao et al., Reference Romero-Lankao, Bruns and Wiegleb2018). This study found that lack of power to refrigerators and freezers caused food to spoil and the damaged transportation system prevented food deliveries from reaching stores (Romero-Lankao et al., Reference Romero-Lankao, Bruns and Wiegleb2018).
3.5 Other systems
Although food, energy, and water systems were the focus of this study, the results show that three other systems were commonly included in the relevant studies (Figure 1). Transportation was the most common system to be included along with food–energy–water systems (12 of 20). Health was the next most common system (10 of 20) followed by communication/telecommunication (6 of 20).
As previously detailed, transportation was often incorporated into the food–energy–water nexus as it relates to food retailers in urban areas. Transportation was also included in several studies and its indirect impact on multiple other systems after an acute event. If roads are closed or blocked, fuel and supplies cannot be obtained to operate backup generators (Cimellaro et al., Reference Cimellaro, Crupi, Kim and Agrawal2019; de Bruijn et al., Reference de Bruijn, Maran, Zygnerski, Jurado, Burzel, Jeuken and Obeysekera2019; Romero-Lankao & Norton, Reference Romero-Lankao and Norton2018; Romero-Lankao et al., Reference Romero-Lankao, Bruns and Wiegleb2018). A closed or blocked transportation system can also mean personnel cannot reach other systems to repair them from acute hazard damage, such as downed power lines (de Bruijn et al., Reference de Bruijn, Maran, Zygnerski, Jurado, Burzel, Jeuken and Obeysekera2019) or bridges in need of inspection (Cimellaro et al., Reference Cimellaro, Crupi, Kim and Agrawal2019).
Health was included in several studies as it was connected to the food, energy, and water systems, with particular emphasis on the influence of energy systems. As shown in Figure 1, health was impacted by disruptions to food, energy, water, and transportation systems. Namely, water, food, and airborne illnesses were associated with lack of power after an extreme event. After the 2013 flood in Boulder, CO, foodborne illness arose when there was no power for refrigeration units and food spoiled (Romero-Lankao & Norton, Reference Romero-Lankao and Norton2018). Waterborne illness can arise when there is no power for water treatment plants or when water sources become contaminated from overflowing sanitation systems after an acute event such as floods (Romero-Lankao & Norton, Reference Romero-Lankao and Norton2018). The use of backup electric generators led to air (respiratory illness) and noise pollution (García-López, Reference García-López2018).
Studies also integrated the food–energy–water nexus with healthcare and environmental justice issues that may impact health (Cimellaro et al., Reference Cimellaro, Crupi, Kim and Agrawal2019; de Bruijn et al., Reference de Bruijn, Maran, Zygnerski, Jurado, Burzel, Jeuken and Obeysekera2019; García-López, Reference García-López2018). For example, after Hurricane Sandy, healthcare facilities had to stop operations due to an electrical outage, lack of access to water, and transportation (Cimellaro et al., Reference Cimellaro, Crupi, Kim and Agrawal2019). Health was also included in terms of heat-related illness, especially with the increasing temperatures associated with climate change and the urban heat island effect (Brown, Reference Brown2019). In terms of environmental justice in Puerto Rico, the lower-income communities located next to toxic storage facilities (like the mountain of coal ash from the coal power plant) were at greater risk from flood events due to potential spills (García-López, Reference García-López2018).
Communication/telecommunication was included as critical infrastructure and primarily in terms of its connection to the energy system. In New York City, Hurricane Sandy caused direct damage to telecommunication facilities and indirect damage to them from an electrical outage which stopped telecommunications operations (Cimellaro et al., Reference Cimellaro, Crupi, Kim and Agrawal2019). The communication lines suffered no direct impacts from a hypothetical flood event in Fort Lauderdale, but, equally important for functionality, the system shut down due to lack of power (de Bruijn et al., Reference de Bruijn, Maran, Zygnerski, Jurado, Burzel, Jeuken and Obeysekera2019). This study also noted that the communication system was dependent on the transportation system because if the roads were closed then no backup equipment could be supplied. This highlighted the importance of having multiple methods to provide FEW system services, thus minimizing the impact of any one method on the system. To exemplify this, after the 2013 flood in Boulder, CO, landlines and radios were used as backups to mitigate the impact of losing cell towers due to power outages (Romero-Lankao & Norton, Reference Romero-Lankao and Norton2018).
4. Discussion
The results support that, due to the unique nature of any given extreme event on a coastal system, there is no ‘one size fits all’ way to incorporate the food–energy–water (FEW) nexus into coastal resilience. Nonetheless, five broad conclusions can be drawn: (1) the FEW nexus approach was most commonly applied to coastal resilience in the study of US locations and in the context of acute hazards, (2) a direct FEW or other nexus approach was directly employed by only half of the studies, however, all of the studies highlighted the relevance of systems interconnections in the context of coastal resilience, (3) the energy system was shown to impact every system to which it was connected, (4) the transportation system was also shown to impact every system to which it was connected, which suggests that the FEW nexus should be used as a starting framework and expanded to include transportation systems, and (5) although the studies that resulted from this systematic literature review were in the context of research and did not directly address planning, there are several lessons (or areas of future research) that can inform resilience planning.
(1) The FEW nexus approach was most commonly applied to coastal resilience in the study of US locations and in the context of acute hazards.
The majority of the 20 studies that met the search criteria were of US locations and the majority of the authors were from the US or European countries. The US focus of the studies could be attributed to its long history of hurricanes. For example, between 1980 and 2008 US hurricanes alone resulted in 57% of global economic losses from extreme weather events (Mohleji & Pielke, Reference Mohleji and Pielke2014). It is also possible that the US focus is due to funding availability (i.e., the United States is a large contributor of research funding) or that one of the systematic literature review search criteria was that papers be available in English. Either way, this finding serves as a call to investigate the food–energy–water nexus in the context of coastal resilience in non-US countries to expand the results of this study.
Additionally, most of the studies that met the search criteria focused on acute hazards, predominantly hurricanes and flood events. This is likely due to the fact that these disruptive events highlight the interdependencies between food, energy, and water systems; meaning, how disruption to one system can cause negative impacts on other systems. However, the alternate nexus perspective, as considered in a few of the studies (e.g., Brown, Reference Brown2019; Dal Bo Zanon et al., Reference Dal Bo Zanon, Roeffen, Czapiewska, de Graaf-Van Dinther and Mooij2017; Luqman & Al-Ansari, Reference Luqman and Al-Ansari2020), is to consider systems interconnections in terms of positive, co-benefits. For example, the study of ‘floating production’ leveraged food–energy–water systems interconnections as co-benefits, where human waste provides a nutrient source for a hydroponics system that can generate biofuel from algae and fish and produce (Dal Bo Zanon et al., Reference Dal Bo Zanon, Roeffen, Czapiewska, de Graaf-Van Dinther and Mooij2017). The findings suggest that acute events are an important context to apply the FEW nexus and they highlight a need for future research into the co-benefit perspective.
(2) A direct FEW or other nexus approach was employed by only half of the 20 studies; however, all of the studies highlighted the relevance of systems interconnections in the context of coastal resilience. Applying a nexus approach within the context of coastal resilience is a relatively new phenomena, hence, there is room for growth to enhance research and to further explore the intersection of nexus approaches and coastal resilience.
Considering the food–energy–water nexus during coastal resilience research and planning provides a method to consider systems interconnections that can reduce illness, loss of life, and damage to infrastructure that may result from an extreme event. Although the results found that some studies did not directly employ a food–energy–water nexus approach, they all recognized the potential impacts one food–energy–water system might have on another. The studies suggested that considering the linkages between multiple critical systems was beneficial to community resilience as tradeoffs were able to be identified, and subsequently, the suite of systems and perspectives incorporated into resilience could be expanded.
Incorporating the food–energy–water nexus approach into coastal resilience research and planning provides a framework for how to consider the interconnections and inherent tradeoffs between multiple systems (food, energy, water, and more). Multiple benefits and efficiencies can arise when systems are considered together. For example, in a study of infrastructural ecology, Brown (Reference Brown2019) demonstrated that intentionally linking power, water, sanitation, transport, and food systems ‘may produce efficiencies simply by sharing structure or space. Once conjoined, these otherwise unrelated sector systems may additionally achieve lower first- and/or operating costs’ (p. 22).
Applying the food–energy–water nexus approach in the context of coastal resilience can intentionally bring a diversity of perspectives to the resilience planning process. Resilience planning involves internal coordination between those in a planning body and with external actors (Sellberg et al., Reference Sellberg, Ryan, Borgström, Norström and Peterson2018). This is evidenced by the study that applied the DESL framework on the island of Zanzibar, which found that the combined participatory and nexus framework approach ‘supported learning between both communities and professionals as well as among institutions, which ordinarily work in isolation’ (Newman et al., Reference Newman, Capitani, Courtney-Mustaphi, Thorn, Kariuki, Enns and Marchant2020, p. 13). A study of knowledge co-production across the energy–water–food nexus intentionally sought to learn from the expertise of stakeholders that work across the nexus to overcome the limitation of siloed approaches (Howarth & Monasterolo, Reference Howarth and Monasterolo2017).
Applying the food–energy–water nexus framework to coastal resilience is supported by Stringer et al. (Reference Stringer, Quinn, Le, Msuya, Pezzuti, Dallimer, Afionis, Berman, Orchard and Rijal2018) who developed a framework for how to integrate the nexus approach with resilience thinking, which they call the WEF-PIK resilience framework (water–energy–food nexus and connections between policies, institutions, and knowledges). This study reports that the WEF-PIK framework can go beyond what either a nexus approach or resilience thinking could do on its own, particularly in terms of equitable outcomes.
All the studies in this analysis were published within the last 5 years (since 2016), which means that this is a relatively new area of research. The multiple benefits of the food–energy–water nexus approach, which include considering tradeoffs, linkages, and bringing diverse perspectives into consideration, are a natural complement to resilience and merits further research.
(3) Across the 20 studies, the energy system was shown to impact every system to which it was connected. This included food, water, transportation, communications, and health systems.
The centrality of the energy system across the studies suggests that coastal resilience should consider an energy-centric perspective of the food–energy–water nexus in the context of future research or planning. This is true, especially in more urban areas where the food–energy–water nexus interconnections are more pronounced. Several of the studies found that the damage to the energy system was a central link and had the most cascading, indirect impacts on other systems, such as water, health, and food (Cimellaro et al., Reference Cimellaro, Crupi, Kim and Agrawal2019; de Bruijn et al., Reference de Bruijn, Maran, Zygnerski, Jurado, Burzel, Jeuken and Obeysekera2019; Romero-Lankao & Norton, Reference Romero-Lankao and Norton2018; Romero-Lankao et al., Reference Romero-Lankao, Bruns and Wiegleb2018). This observation is supported by other studies, such as how the energy system was found to be the ‘key sector to propagate risks to other sectors’ in New York City after Hurricane Sandy (Haraguchi & Kim, Reference Haraguchi and Kim2016, p. 133) and a study conducted in Puerto Rico after Hurricane Irma and Maria found that ‘understanding the relationships of infrastructure recovery efforts between power infrastructure systems and the other [critical infrastructures] has the potential to be a key in developing an effective recovery plan leading to resilient infrastructure systems, and thereby a more resilient community’ (Sarker & Lester, Reference Sarker and Lester2019, p. 1).
Applying an energy-centric approach to the food–energy–water nexus within coastal resilience would tie to the prior discussion of which groups and systems are brought to the table during research and planning. Hypothetically, this could manifest as the energy system and its critical ties to other systems being highlighted by inviting members of the local utility to meetings, through the systems inclusion in questionnaires and interview guides, or including the energy system centrally in modeling endeavors. This is supported by a study of system restoration interdependencies, which found that ‘information from and communications with the restoration efforts of the energy sector could be quite valuable in restoration efforts across sectors’ (Sharkey et al., Reference Sharkey, Nurre, Nguyen, Chow, Mitchell and Wallace2016, p. 8).
As the cascading impacts of damage to the energy system were highlighted in several of the studies, it was curious to see a lack of consideration or discussion of SmartGrid technology in the studies that met the selection criteria. SmartGrid solutions, such as energy storage, distributed generation (via microgrids), and demand side management, could help increase the resilience of the energy system in the face of disasters (Panteli & Mancarella, Reference Panteli and Mancarella2015). Future research would benefit from including a consideration of SmartGrid technologies into the study of coastal resilience using a FEW nexus approach.
(4) The transportation system was also shown to impact every system it was connected to (i.e., food, energy, water, communications, and health), which suggests that the FEW nexus should be used as a starting framework and expanded to include the transportation system.
The results indicate that just a food–energy–water nexus approach may be too narrow within the context of coastal resilience. Transportation was shown to have direct and indirect impacts on many other systems, most notably, the food system in urban areas. The urban food system is dependent on food retailers (critical commercial services), which, in turn, rely on the transportation system for the delivery of food from surrounding areas and the global market. This is supported by Paci-Green and Berardi (Reference Paci-Green and Berardi2015) who note that the food system primarily relies on the transportation and energy systems and regional farm production can increase resilience from natural hazards when combined with a regional food resilience strategy. The FEW approach should include the transportation system in the nexus (the FEWT nexus, if you will).
Given the reliance of the food system on transportation and surrounding regions/networks, the locavore movement seems a natural connection that was curiously missing from all of the studies. Aside from the two innovations, the multigeneration machine and floating production, none of the studies considered resource production more locally as a component of resilience. Local food production as a component of resilience is supported by Smith et al. (Reference Smith, Lawrence, MacMahon, Muller and Brady2016) who studied long and short food chains after a flood event in Queensland, Australia. The flood event cut off roads, which resulted in food shortages. Short (more local) food chains were found to be more resilience than long (supermarket-based) food chains (Smith et al., Reference Smith, Lawrence, MacMahon, Muller and Brady2016).
Results suggest that considering how the FEW nexus connects to other systems should be encouraged in coastal resilience research and planning. For example, although health was not shown to have an impact on other systems in the sample of studies used in this analysis, food, energy, water, and transportation systems were each shown to impact health.
(5) Although the studies that resulted from this systematic literature review were in the context of research and did not directly address planning, there are several lessons (or areas of future research) that can inform resilience planning.
Although the studies in this systematic literature review were largely implemented in response to acute hazards, they offer valuable lessons in coastal resilience planning. Food–energy–water nexus approaches are rarely considered within planning (e.g., a food–energy–water nexus approach was not found to be employed in US coastal resilience planning; Raub et al., Reference Raub, Stepenuck, Panikkar and Stephens2021) and can be an important tool in building resilience and in disaster preparation and management (e.g., see Burby et al., Reference Burby, Beatley, Berke, Deyle, French, Godschalk, Kaiser, Kartez, May, Olshansky, Paterson and Platt1999; Cucuzza et al., Reference Cucuzza, Stoll and Leslie2020; Nelson and French, Reference Nelson and French2002). The results from this study are most salient to planners to (1) consider applying a nexus approach within their plans and to the planning process, especially within the context of disasters and (2) although utilizing the nexus approach, planners should also include and explore other systems interconnections, such as transportation. As evidenced by Romero-Lankao and Norton (Reference Romero-Lankao and Norton2018) and Newman et al. (Reference Newman, Capitani, Courtney-Mustaphi, Thorn, Kariuki, Enns and Marchant2020), applying a food–energy–water nexus approach to their study ensured that a diverse group of stakeholders were included in the research process. Utilizing a nexus approach thus allows planners to use an integrated systems approach to planning that considers the interconnections between biological and physical systems, approaches, and interactions between collaborators.
5. Limitations and future research
The most notable limitation to this study was that only Web of Science was used to discover studies that incorporated the food–energy–water nexus within the context of coastal resilience. Web of Science was selected for its advanced search capabilities given the nuanced nature of this study's search criteria and for its broad selection of journals. However, not all journals are indexed within Web of Science and it excludes many books and grey literature sources. Future research should investigate other databases and include books.
Additionally, the scope of this study only considered resilience research from an academic perspective as investigating grey literature would require a different approach and methodology. For example, there is much being done by US cities, towns, and states that has not been published in peer-reviewed journals and was therefore not captured in the scope of this study. Resilience can be found in grey literature, such as city comprehensive plans, the resilience plans produced through collaboration with 100 resilient cities, and hazard mitigation plans. Future research is needed to determine how the nexus of food, energy, water, and transportation systems have been included into grey literature, specifically, city resilience plans.
Many studies were excluded during the title and abstract search because they did not include food, energy, and water systems or they focused on only a subset of the systems. An avenue for future research would be to expand the systematic literature review search criteria to discover, broadly, which interconnected systems are most commonly included in studies of coastal resilience. The transportation, health, and communications systems could be used as a starting point.
Most of the studies considered an acute hazard (14 of 20) and were in urban areas (13 of 20). This emphasis on urban areas and acute hazards may partially be an artifact of the search criteria. For example the definition of ‘coastal’ was expanded to include riverine locations but only if those studies incorporated an acute, water-related hazard. Therefore, additional research is needed to better understand how the food–energy–water nexus could be applied to the study of coastal resilience in rural areas and with chronic hazards. Additionally, future research could compare how the food–energy–water nexus has been incorporated into studies of coastal resilience with those of riverine resilience.
6. Conclusions
Studies focused on food, energy, and water systems revealed strong interdepencies of these systems largely as related to acute hazards in urban areas. Of particular influence, when damaged, the energy system impacted nearly every system to which it was connected. Similarly, the food system, as a critical commercial service, was demonstrated to rely heavily upon the transportation system to replenish its supplies. Both the energy system and transportation systems were essential to improve access to key services and address impacts from acute coastal hazards, resulting from hurricanes and flood events. As such, an energy-centric perspective and intentionally broadening the food–energy–water nexus to include transportation systems may be warranted in the context of coastal resilience planning efforts in urban areas. With the number of acutely hazardous events projected to increase in severity and frequency in coastal areas due to climate change, applying a food–energy–water–transportation nexus approach to the study of coastal resilience could help ensure the stability of critical infrastructure systems in coastal urban communities during crises. The results from this study suggest potential new strategies to investigate to improve coastal resilience.
Supplementary material
The supplementary material for this article can be found at https://doi.org/10.1017/sus.2021.20.
Acknowledgements
The authors would like to acknowledge Dr. Jennie Stephens and Dr. Asim Zia for feedback on an early draft and our anonymous reviewers for their constructive feedback that helped to improve the article.
Author contributions
K.B.R. conducted the systematic literature review, led the analysis, and was the primary writer of the manuscript. K.F.S. and B.P. each were consulted during the analysis, assisted in framing the scope of the study, and provided in-depth edits and feedback.
Financial support
This research received no specific grant from any funding agency, commercial, or not-for-profit sectors.
Conflict of interest
K.B.R, K.F.S., and B.P. declare none.
Research transparency and reproducibility
Data and methods are included in the article and in Appendix A.