2.1 Introduction
Squamata, the group that encompasses snakes, lizards, and amphisbaenians, is the largest (>10,500 sp.) and most disparate group of modern reptiles. Extant squamates are distributed over all but the coldest parts of the world; range in size (snout–vent length, SVL) from millimetres to metres; and show a diversity of diets, shapes, locomotor patterns, and reproductive strategies. Snakes (Serpentes) account for roughly 35 per cent of all extant squamate species and their origin and relationships, which have long intrigued herpetologists, are the focus of this volume. This chapter aims to provide a foundation for subsequent chapters, by reviewing what is currently known of the early stages of squamate evolution and diversification.
Most researchers recognize eight extant major squamate clades (Fig. 2.1): Dibamidae; Gekkota; Scincoidea (=Scinciformata [Reference Vidal and Hedges1]), encompassing Xantusiidae, Scincidae, and Cordyliformes; Lacertoidea (=Laterata [Reference Vidal and Hedges1]), encompassing Lacertidae and Teiioidea; Amphisbaenia; Iguania; Anguimorpha; and Serpentes. Although Camp [Reference Camp2] considered Gekkota to be primitive squamates (part of his Ascalabota), the first comprehensive cladistic analysis [Reference Estes, De Queiroz, Gauthier, Estes and Pregill3], based on morphological characters, placed Iguania as the sister group of other squamates (Scleroglossa). Within Scleroglossa, Estes et al. [Reference Estes, De Queiroz, Gauthier, Estes and Pregill3] united Scincoidea + Lacertoidea in Scincomorpha, and Scincomorpha + Anguimorpha as Autarchoglossa. The position of three limb-reduced clades, Dibamidae, Amphisbaenia, and Serpentes, was unresolved within Scleroglossa. The topology of Estes et al. [Reference Estes, De Queiroz, Gauthier, Estes and Pregill3] remained the working hypothesis for most herpetologists until 2004 with the publication of phylogenies based on molecular data [Reference Townsend, Larson, Louis and Macey4, Reference Vidal and Hedges5]. The molecular trees placed Gekkota rather than Iguania as the sister group of other squamates (invalidating Scleroglossa), with Scincoidea, and then Lacertoidea (including Amphisbaenia) as successive outgroups to a Toxicofera that encompassed Iguania, Anguimorpha, and Serpentes [Reference Vidal and Hedges1]. Although morphological data sets (e.g., [Reference Gauthier, Kearney, Maisano, Rieppel and Behlke6]) tend to replicate the Estes et al. [Reference Estes, De Queiroz, Gauthier, Estes and Pregill3] tree, subsequent molecular and combined evidence analyses [Reference Wiens, Hutter and Mulcahy7–Reference Burbrink, Grazziotin and Pyron13] agree with those of Townsend et al. [Reference Townsend, Larson, Louis and Macey4] and Vidal and Hedges [Reference Vidal and Hedges5]. The molecular tree is therefore used as the phylogenetic framework herein (Fig. 2.1).
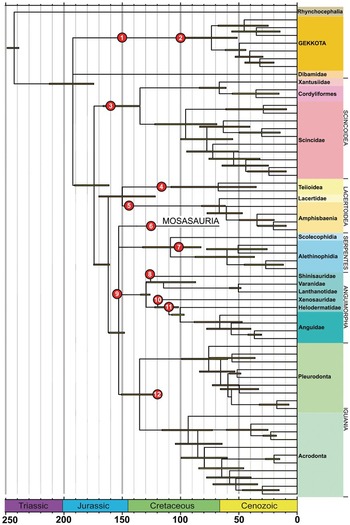
Figure 2.1 Molecular time tree for Squamata, redrawn and simplified from Jones et al. [Reference Jones, Anderson and Hipsley14], showing putative early representatives of major clades discussed in the text. (1) Eichstaettisaurus (Upper Jurassic, Germany); (2) Hoburogekko (Lower Cretaceous, Mongolia); (3) Paramacellodidae and Saurillodon (Upper Jurassic, Portugal); (4) Asagaolacerta, Kuwajimalla , Polyglyphanodontia (Lower Cretaceous, Japan); (5) Purbicella (Lower Cretaceous, UK); (6) Kaganaias (Lower Cretaceous, Japan); (7) ‘Coniophis ’ (Albian-Cenomanian, North America) and terrestrial + aquatic snakes (Albian-Cenomanian, Algeria); (8) Dalinghosaurus (Lower Cretaceous, China); (9) Dorsetisaurus (Upper Jurassic to Lower Cretaceous, Pan-Laurasia); (10) ?Xenostius (Early Cretaceous, Mongolia); (11) Primaderma (Albian–Cenomanian, North America); (12) ?Hoyalacerta (Early Cretaceous, Spain). See Supplementary Figure 2.S1 for terminal taxon labels in full.
2.2 The Early Squamate Fossil Record
Within Lepidosauria, Squamata is the sister group of Rhynchocephalia. Rhynchocephalia is represented today only by the genus Sphenodon (New Zealand), but the group has a relatively good fossil record through much of the Mesozoic. The occurrence of a primitive rhynchocephalian in the Middle Triassic of Germany [Reference Jones, Anderson and Hipsley14] provides a latest possible age for the division of the lepidosaurian stem, although that division, and thus the origin of Squamata, probably occurred in the Early Triassic [Reference Pyron12, Reference Jones, Anderson and Hipsley14]. However, although there are many records of Triassic rhynchocephalians, there are currently no unequivocal records of Triassic squamates. Those described last century have all been re-assigned to other reptile groups or to the lepidosaurian stem [Reference Evans15, Reference Evans, Jones and Bandyopadhyay16].
Tikiguana estesi, represented by a single jaw with an acrodont dentition collected from Upper Triassic deposits in India [Reference Datta and Ray17], was shown to be a modern intrusion [Reference Hutchinson, Skinner and Lee18]. More recently, Simões et al. [Reference Simões, Caldwell and Tałanda19] placed three monotypic genera, Sophineta cracoviensis (Lower Triassic, Poland, [Reference Evans and Borsuk-Białynicka20]), Megachirella wachtleri (Upper Triassic, Italy [Reference Renesto and Posenato21]), and Marmoretta oxoniensis (Middle Jurassic, UK [Reference Evans22]) into Squamata. However, re-analyses, based on new data for Marmoretta [Reference Griffiths, Ford, Benson and Evans23], do not support a squamate attribution for either Marmoretta or Sophineta , and leave the position of Megachirella as equivocal.
Paikasisaurus indicus is based on two jaw fragments from the Lower–Middle Jurassic Kota Formation of India [Reference Yadagiri24]. The designated holotype bears two teeth, neither of which is obviously pleurodont, whereas the referred specimen bears a single tooth with little resemblance to that of the holotype. Bharatagama rebbanensis, also from the Kota Formation, is represented by multiple jaws bearing both pleurodont and acrodont teeth [Reference Evans, Prasad and Manhas25]. It was described as a possible acrodont iguanian but could also be an aberrant rhynchocephalian [Reference Hutchinson, Skinner and Lee18, Reference Conrad26].
The earliest unequivocal squamates are from the Middle Jurassic (Bathonian) of the United Kingdom, Russia, Kyrgyzstan, and Morocco. One of the most productive Bathonian localities has been Kirtlington Quarry (UK, [Reference Evans27, Reference Evans28]). In addition to the lepidosauromorph Marmoretta , Kirtlington yielded five named squamate genera: Balnealacerta, Bellairsia, cf. Saurillodon, Oxiella, and Parviraptor . Squamate bones were also reported from roughly contemporaneous deposits on the Isle of Skye, Scotland [Reference Griffiths, Ford, Benson and Evans23], and new fieldwork on Skye has yielded both isolated elements and associated material, including specimens referable to the Kirtlington genera Balnealacerta, Bellairsia, and Parviraptor [Reference Pancirioli, Benson and Walsh29]. These specimens both validate the original associations [Reference Evans27, Reference Evans28], contra Caldwell et al. [Reference Caldwell, Nydam, Palci and Apesteguia30], and add new morphological data for phylogenetic analysis. Work on this material is in progress, but it highlights the clear morphological gap between lepidosaurian stem taxa, like Marmoretta, and squamates from the Middle Jurassic onward that show derived features including biradiate squamosals, loss of quadrate–pterygoid fixation, loss of quadratojugal, a synovial epipterygoid–pterygoid joint, and subdivision of the metotic fissure in the braincase.
Among other Middle Jurassic records, Changetisaurus estesi (Bathonian, Kyrgyzstan [Reference Fedorov and Nessov31]) is a partial skeleton attributed to Squamata, but it requires re-study. Squamate remains are also recorded from the Bathonian Berezovsk Mine, western Siberia (a possible paramacellodid, two ‘scincomorphs’: [Reference Averianov, Martin and Skutschas32]) and from similar aged deposits in Morocco (‘scincomorphs’, ‘Parviraptor-like’ taxon [Reference Haddoumi, Allain and Meslouh33]). An osteoderm-covered lizard was briefly described from Callovian–Oxfordian age deposits at Shishougou (Junggar Valley, China [Reference Conrad, Wang, Xu, Pyron and Clark34]), but a detailed description and analysis has yet to be published. Roughly contemporaneous deposits at Daohugou, Chinese Inner Mongolia, have also yielded associated lizard skeletons, one of general proportions and the other with elongate limbs [Reference Evans and Wang35, Reference Evans and Wang36], but both are immature and the preservation is poor. More recently, however, a complete lizard skeleton was recovered from Daohugou equivalent deposits at Guancaishan, Liaoning Province (Hongshanxi xiei, [Reference Dong, Wang, Mou, Zhang and Evans37]).
Moving into the Upper Jurassic, squamate material is known from several additional Laurasian localities, notably: Portugal (Oxfordian, Guimarota [Reference Seiffert38]), Kazakhstan (Oxfordian–Kimmeridgian, Karabastau Formation [Reference Hecht and Hecht39]), North America (Kimmeridgian, Morrison Formation [Reference Prothero and Estes40–Reference Evans and Chure42]), Germany (Tithonian, Solnhofen and neighbouring localities [Reference Conrad26, Reference Cocude-Michel43–Reference Talanda48] and France (Kimmeridgian, Cerin [Reference Evans49]). Unfortunately, the only contemporaneous Gondwanan record is a ‘paramacellodid’ osteoderm from the Tendaguru Formation of Tanzania [Reference Zils, Werner, Moritz and Saanane50], but this identification is unconfirmed.
The squamate fossil record improves substantially in the Early to Middle Cretaceous (i.e., Berriasian–Cenomanian), with specimens from Europe, Africa, the Middle East, Asia, and the Americas. Concurrent with this apparent global expansion, Early Cretaceous (Barremian) specimens include some of the first known occurrences of squamate gliding [Reference Li, Gao, Hou and Xu51], body elongation with limb reduction [Reference Evans, Manabe, Noro, Isaji and Yamaguchi52], viviparity [Reference Wang and Evans53], herbivory [Reference Evans and Manabe54], and specialist climbing [Reference Evans and Barbadillo55, Reference Arnold and Poinar56].
2.3 The First Records of Crown-Group Squamates
Most recent molecular divergence estimates date the first radiation of crown-group squamate lineages to the Early–Middle Jurassic [Reference Pyron12–Reference Jones, Anderson and Hipsley14], with further splitting of major lineages in the Late Jurassic. As yet, however, none of the known Middle Jurassic taxa, and relatively few of those from the Late Jurassic, can be placed unequivocally into crown lineages (Fig. 2.1). There is some improvement in the Early Cretaceous, but many lizards from this period are phylogenetically problematic and behave as wild cards in analyses.
2.3.1 Gekkota
Given that gekkotans are now considered to be one of the first of the major branches to diverge from the ancestral crown squamate, they should be well represented in early squamate assemblages. Their apparent absence could be due to preservational or ecological constraints, or a failure of identification due to a lack of distinctive gekkotan characters in their early history. Several Late Jurassic and Early Cretaceous genera (notably Ardeosaurus, Bavarisaurus, Eichstaettisaurus, Palaeolacerta, Yabeinosaurus ) were originally attributed to Gekkota (e.g., [Reference Hoffstetter44]), but a majority of these have been re-assigned. One exception is Eichstaettisaurus (Upper Jurassic, Germany [Reference Cocude-Michel43]; Lower Cretaceous, Spain, Italy [Reference Evans, Lacasa-Ruiz and Erill Rey57, Reference Evans, Raia and Barbera58]) which is frequently placed on the gekkotan stem (e.g., [Reference Gauthier, Kearney, Maisano, Rieppel and Behlke6, Reference Pyron12, Reference Conrad59]), albeit with limited evidence. The first unequivocal stem gekkotan is Hoburogekko suchanovi (Aptian–Albian, Höövor, Mongolia [Reference Alifanov60, Reference Daza, Bauer and Snively61]) although Norellius nyctisaurops (Aptian–Albian, Mongolia [Reference Conrad and Norell62, Reference Conrad and Daza63]) has also been assigned to Gekkota (e.g., [Reference Pyron12], but see [Reference Reeder, Townsend and Mulcahy9]), based primarily on the combination of notochordal vertebrae and paired parietals. In recent years, Cretaceous amber has offered an important window into gekkotan history because it is able to preserve small, delicate skeletons, often with exquisite soft tissues (e.g., Cretaceogekko burmae [Reference Arnold and Poinar56]). The Albian–Cenomanian amber deposits of north-western Myanmar are particularly rich in gekkotans and stem gekkotans from a rarely sampled tropical forest ecosystem [Reference Daza, Stanley, Wagner, Bauer and Grimaldi64, Reference Fontanarrosa, Daza and Abdala65].
2.3.2 Dibamidae
The phylogenetic position of this small, specialized, and biogeographically disparate clade (Dibamus , South East Asia, New Guinea, Philippines; Anelytropsis , Mexico) has long been problematic (e.g., [Reference Estes, De Queiroz, Gauthier, Estes and Pregill3, Reference Greer66]). Molecular analyses have provided greater clarity but there remains uncertainty as to whether dibamids are sister to all other squamates including gekkotans [Reference Zheng and Wiens10, Reference Streicher and Wiens11], to gekkotans alone [Reference Burbrink, Grazziotin and Pyron13], or to all squamates except gekkotans [Reference Pyron12]. Unfortunately, the group has no Mesozoic record. The only putative fossil dibamid is Hoeckosaurus mongoliensis from the Oligocene of Mongolia [Reference Cernansky67].
2.3.3 Scincoidea
Estes et al. [Reference Estes, De Queiroz, Gauthier, Estes and Pregill3] grouped scincoids and lacertoids within Scincomorpha. Consequently, many fossil lizard remains are classified simply as ‘scincomorphs’, making them difficult to attribute to any clade. Scincoidea is probably represented by the Jurassic–Cretaceous Paramacellodidae [Reference Estes and Sauria68] with their body covering of rectangular osteoderms, and possibly by short-jawed Jurassic–Cretaceous taxa like Saurillodon (Fig.2.1). Saurillodon (S. proraformis, S. henkeli ) was first described from Guimarota (Oxfordian, Portugal) based on short robust dentaries with a few large conical teeth [Reference Seiffert38, Reference Kosma69], resembling the jaws of limb-reduced modern scincids and amphisbaenians. Similar jaws were reported from Anoual (Lower Cretaceous, Morocco, Tarratosaurus anoualensis [Reference Broschinski and Sigogneau‑Russell70]) and from Kirtlington (Middle Jurassic, UK [Reference Evans28]). The Kirtlington specimens were referred to Saurillodon, and tentatively associated with unusual elongated vertebrae. If the association is correct, then this Middle Jurassic taxon would be of considerable interest as an early long-bodied, and presumably limb-reduced, squamate. Balnealacerta , also from Kirtlington [Reference Evans28] was attributed to Paramacellodidae based on similarities in jaw and tooth structure, as were dentaries from contemporaneous localities on Skye [Reference Griffiths, Ford, Benson and Evans23]. However, no osteoderms were recovered from among the many kilos of residue from Kirtlington sorted by the author, nor are they known from Skye (e.g., in association with the new material of Balnealacerta [Reference Pancirioli, Benson and Walsh29]), and therefore attribution of these Middle Jurassic remains to Paramacellodidae remains tentative. Nonetheless, paramacellodids (including osteoderms) are recorded from Upper Jurassic deposits including those from Guimarota (Oxfordian [Reference Seiffert38, Reference Broschinski, Martin and Krebs71]), Kazakhstan (Kimmeridgian, Sharovisaurus [Reference Hecht and Hecht39]), and the Morrison Formation, USA (Kimmeridgian [Reference Prothero and Estes40, Reference Evans and Chure41]), as well as from the Lower Cretaceous of the United Kingdom [Reference Hoffstetter72], China [Reference Li73], Russia [Reference Averianov and Skutchas74], Spain [Reference Richter75], Morocco [Reference Richter75], and North America [Reference Nydam and Cifelli76]. Most recently, a partial lizard skeleton, Neokotus sanfranciscanus (Valanginian, Brazil), was described as a possible Gondwanan paramacellodid [Reference Bittencourt, Simões, Caldwell and Langer77], but no osteoderms are associated with the skeleton and the attribution is based mainly on dental morphology.
Other than paramacellodids, the well-preserved Tepexisaurus tepexii (Albian, Mexico [Reference Reynoso and Callison78]) may also be a scincoid [Reference Gauthier, Kearney, Maisano, Rieppel and Behlke6], but other attributions have less support. Talanda [Reference Talanda48] argued that Ardeosaurus (Upper Jurassic, Germany) was a scincid, but this was based on an interpretation of cranial ornamentation as representing compound osteoderms. By contrast, Simões et al. [Reference Simões, Caldwell, Nydam and Jiminez-Huidabro47] recovered Ardeosaurus as a gekkotan, whereas other researchers (e.g., [Reference Pyron12]) found Ardeosaurus to be a wild-card taxon in analyses. Finally, Calanguban alamoi is known from a poorly preserved skeleton from Brazil (Aptian–Albian, Crato Formation [Reference Simões, Wilner, Caldwell, Weinschutz and Kellner79]). It was recovered within Cordyliformes in an analysis using the Conrad [Reference Conrad59] matrix, but unresolved using Gauthier et al.’s [Reference Gauthier, Kearney, Maisano, Rieppel and Behlke6] matrix. Neither analysis attempted to use a molecular constraint.
2.3.4 Lacertoidea
Lacertoidea encompasses lacertids, amphisbaenians, teiids and gymnophthalmids, and may be represented by Purbicella ragei from the Lower Cretaceous of England (Berriasian, Purbeck Limestone Group [Reference Evans, Jones and Matsumoto80]). Meyasaurus spp. (Barremian, Spain) was proposed to lie on the teiioid stem by Evans and Barbadillo [Reference Evans and Barbadillo81], but within anguimorphs by Richter [Reference Richter82] and Conrad [Reference Conrad59]. Tijubina pontei (Aptian–Albian, Crato Formation, Brazil) was originally attributed to Teiidae [Reference Bonfim-Junior and Marques83], but subsequent researchers either failed to place it securely within the squamate crown [Reference Bonfim-Junior and Avila84, Reference Simões85] or suggested that it and a second Brazilian species (Olindalacerta brasiliensis [Reference Evans and Yabumoto86]) might be polyglyphanodonts [Reference Simões, Wilner, Caldwell, Weinschutz and Kellner79]. Until recently, Polyglyphanodontia were classified as teiioids (e.g., [Reference Estes and Sauria68, Reference Alifanov87, Reference Nydam, Eaton and Sankey88]), a position also recovered by Pyron [Reference Pyron12] using a combined morphological [Reference Conrad59] and molecular matrix. However, Gauthier et al. [Reference Gauthier, Kearney, Maisano, Rieppel and Behlke6] recovered polyglyphanodonts on the stem of ‘Scleroglossa’, whereas Pyron [Reference Pyron12], combining the Gauthier et al. [Reference Gauthier, Kearney, Maisano, Rieppel and Behlke6] matrix with a molecular dataset, found polyglyphanodonts to be the sister group of Iguania. The position of this large and important fossil group therefore remains problematic, but it is attributed to Teiioidea in Figure 2.1. The earliest attributed polyglyphanodonts are Kuwajimalla kagaensis [Reference Evans and Manabe54] and Asagaolacerta tricuspidens [Reference Evans and Matsumoto89] from the Tetori Formation (Barremian–Aptian, Japan). An unnamed lizard specimen preserved in amber (Albian–Cenomanian, Myanmar) was also described as a crown lacertoid [Reference Daza, Stanley, Wagner, Bauer and Grimaldi64], but this attribution was based primarily on comparison with polyglyphanodonts.
Molecular divergence estimates (e.g., [Reference Pyron12]) date the separation of stem amphisbaenians and lacertids to the Late Jurassic–Early Cretaceous, but the earliest unequivocal record of amphisbaenians is from the Palaeocene [Reference Sullivan90]. Hodzhakulia magna (Albian, Uzbekistan [Reference Nessov91]; Mongolia [Reference Alifanov92]) and Sineoamphisbaena hexatabularis (Santonian–Campanian, China [Reference Wu, Brinkman and Russell93]) were originally classified as stem amphisbaenians, but Hodzhakulia is fragmentary (‘scincomorph’ [Reference Alifanov92]) and Sineoamphisbaena may be related to polyglyphanodonts [Reference Pyron12]. The limb-reduced Slavoia darevskii has also been proposed as a candidate stem amphisbaenian [Reference Talanda94]. The type material is from the Campanian of Mongolia, but older ‘slavoiids’ were reported from the Albian Mongolian locality of Höövor [Reference Alifanov95].
2.3.5 Anguimorpha
Parviraptorids (Middle Jurassic–Early Cretaceous, UK [Reference Evans27]) were originally attributed to Anguimorpha, based on jaw and tooth morphology. This attribution will be tested by new, in progress, analyses based on associated material from Skye (Middle Jurassic). The Middle Jurassic Changetisaurus (Bathonian, Kyrgyzstan) was also attributed to Anguimorpha [Reference Fedorov and Nessov31], but this taxon needs re-examination. Currently, the earliest generally accepted anguimorph is Dorsetisaurus purbeckensis. As its name suggests, this taxon was first described from the Lower Cretaceous (Berriasian) Purbeck Limestone Group of England [Reference Hoffstetter72], but dorsetisaurs have subsequently been reported from the Upper Jurassic of Portugal (Guimarota, Oxfordian [Reference Seiffert38, Reference Broschinski, Martin and Krebs71] and the USA (Morrison Formation, Kimmeridgian [Reference Prothero and Estes40], as well as the Early Cretaceous of Mongolia (Aptian–Albian, Paradorsetisaurus postumus [Reference Alifanov96] (Fig. 2.1). Additionally, a small lizard skeleton (Indrasaurus wangi [Reference O’Connor, Zheng and Dong97] found as gut contents within an Early Cretaceous Chinese Microraptor has a dorsetisaur-like dentition and may be related to dorsetisaurs.
Further anguimorph taxa are recorded from the Lower Cretaceous of Mongolia (Aptian–Albian, Xenostius futilis , crown xenosaur [Reference Alifanov95]); Uzbekistan (Albian, xenosaur [Reference Gao and Nessov98]); Thailand (Barremian, eggs with embryos [Reference Fernandez, Buffetaut and Suteethorn99]); China (Barremian, Dalinghosaurus longidigitus , stem shinisaur [Reference Evans and Wang100]); North America (Albian–Cenomanian, Primaderma, stem monstersaur [Reference Cifelli and Nydam101, Reference Nydam102]); England (Wealden, indet. [Reference Sweetman, Evans and Batten103]); and Spain (Barremian–Aptian, Arcanosaurus ibericus , ‘varanoid’ [Reference Houssaye, Rage and Fernandez-Baldor104]), although many of these records are based on isolated elements. The tiny attenuate Barlochersaurus winhtini from the mid-Cretaceous amber of Myanmar was also tentatively attributed to Anguimorpha [Reference Daza, Bauer and Stanley105], but a more mature specimen is needed to verify this because the skull is poorly preserved.
The relationships of mosasaurians, also frequently grouped within Anguimorpha, are discussed separately (§ 2.3.7).
2.3.6 Iguania
This major squamate clade, comprising both pleurodont and acrodont jawed taxa, is first recorded with certainty from the Late Cretaceous (e.g., Mongolia, North America [Reference Estes and Sauria68]. Some analyses (e.g., [Reference Conrad59]) recovered Hoyalacerta sanzi , a short-limbed lizard from the Lower Cretaceous of Spain (Barremian, Las Hoyas [Reference Evans and Barbadillo106]) on the iguanian stem but further specimens are needed to test this attribution. Conrad [Reference Conrad59] also recovered the enigmatic Huehuecuetzpalli mixtecus (Albian, Mexico [Reference Reynoso107]) as a stem iguanian, although other researchers (e.g., [Reference Gauthier, Kearney, Maisano, Rieppel and Behlke6, Reference Reynoso107]) inferred that it lies on the squamate stem. Huehuecuetzpalli combines primitive characters (e.g., notochordal vertebrae, trunk intercentra, retained second distal tarsal) with derived ones (e.g., retracted nares, anterior parietal foramen). A stem-iguanian position was also recovered by Pyron [Reference Pyron12], evaluating both the Conrad [Reference Conrad59] and the Gauthier et al. [Reference Gauthier, Kearney, Maisano, Rieppel and Behlke6] matrices using time calibration and combined evidence. Such major inconsistencies in tree position are, unfortunately, rather common for Jurassic–Early Cretaceous taxa.
Xianglong zhaoi , a gliding lizard from the Lower Cretaceous (Barremian) of China was described as an acrodontan [Reference Li, Gao, Hou and Xu51], but this was based on the misinterpretation of the jagged broken edge of the crushed juvenile skull as an acrodont jaw (pers. obs.). Its affinities remain unknown. The Albian–Cenomanian amber of Myanmar has also yielded possible acrodontans [Reference Daza, Stanley, Wagner, Bauer and Grimaldi64], but this attribution is based on postcranial material and needs to be confirmed from well-preserved skulls. A stem chameleon described from the same deposits [Reference Daza, Stanley, Wagner, Bauer and Grimaldi64] is actually an albanerpetontid amphibian [Reference Matsumoto and Evans108]. Jeddaherdan aleadonta , a partial dentary from the Cenomanian of Kem Kem, in Morocco, is possibly an early acrodontan [Reference Apesteguia, Daza, Simões and Rage109], but Gueragama sudamerica (Turonian–Campanian, Brazil [Reference Simões, Wilner, Caldwell, Weinschutz and Kellner79]) is a partial dentary with teeth like those of scincoids or teiids, and is unlikely to be an acrodontan as proposed.
2.3.7 Mosasauria
This group encompasses the Mosasauroidea (Late Cretaceous mosasaurs and aigialosaurs), and several smaller, long-necked, long-bodied taxa (‘dolichosaurs’) from the Early–Late Cretaceous. Mosasaurians are included briefly in this review chapter for completeness (see the relevant chapters in this volume for a more detailed discussion).
Generally considered close relatives of varanids and/or lanthanotids within Anguimorpha (e.g., [Reference McDowell and Bogert110, Reference Carroll and De Braga111; Chapter 9]), several subsequent cladistic analyses distanced mosasaurians from varanids, albeit to varying degrees (e.g., [Reference Gauthier, Kearney, Maisano, Rieppel and Behlke6, Reference Caldwell, Carroll and Kaiser112–Reference Simões, Vernygora, Paparella, Jimenez-Huidobro and Caldwell114]). The proposal that mosasaurians were closely related to snakes (e.g., [Reference Caldwell113, Reference Lee115, Reference Lee116] and subsequent papers by the same authors and their collaborators) led Lee and Caldwell [Reference Lee and Caldwell117] to resurrect Cope’s [Reference Cope118] name Pythonomorpha for their mosasaurian + snake clade, and generated the associated, and more controversial, suggestion of a marine origin for snakes [Reference Lee and Caldwell117, Reference Lee119] (see also Chapter 7).
There is general agreement as to the monophyly of Mosasauroidea, but not of Aigialosauridae ([Reference Caldwell113] versus [Reference Lee115, Reference Caldwell120, Reference Dutchak121; Chapter 8]). Both groups have their earliest representatives in the Cenomanian [Reference Mekarski, Pierce and Caldwell122]. ‘Dolichosaurs’ are more problematic, mainly because their skulls are poorly known. Recent papers, often with overlapping groups of authors, disagree as to whether ‘dolichosaurs’ are monophyletic (e.g., [Reference Paparella, Palci, Nicosia and Caldwell123; Chapter 9]) or paraphyletic (e.g., [Reference Mekarski, Pierce and Caldwell122, Reference Pierce and Caldwell124]), how they are defined and diagnosed (contrast [Reference Palci and Caldwell125] with [Reference Paparella, Palci, Nicosia and Caldwell123, Reference Pierce and Caldwell124]), and whether they are closer to snakes (forming the Ophidiomorpha of [Reference Mekarski, Pierce and Caldwell122, Reference Palci and Caldwell125]) or to mosasauroids [Reference Paparella, Palci, Nicosia and Caldwell123]. Most ‘dolichosaur’ genera were recovered from shallow marine deposits associated with either the remnant Tethys seaway (Slovenia, Croatia, Kazakhstan, Lebanon, Palestine, United Kingdom, Germany, France, Spain) or the Western Interior Seaway (North America) [Reference Mekarski, Pierce and Caldwell122]. However, the earliest accepted ‘dolichosaurs’ are from freshwater deposits: in Spain (Barremian, vertebrae originally identified as snake [Reference Rage and Richter126]), Japan (Barremian–Albian Kaganaias [Reference Evans, Manabe, Noro, Isaji and Yamaguchi52]); and Australia (Albian [Reference Scanlon, Hocknull and Everhard127]). The enigmatic snake-like Tetrapodophis amplectus (Albian, Brazil [Reference Martill, Tischlinger and Longrich128]), also from a freshwater deposit, was recovered by Paparella et al. [Reference Paparella, Palci, Nicosia and Caldwell123] on the stem of a Mosasauroidea + Dolichosauridae clade that is proposed to be the sister group of snakes (see also Chapter 8).
2.3.8 Pan-Serpentes
The morphological specializations of the snake skeleton and the lack of early fossil representatives have hampered attempts to resolve the relationships of snakes to extant lizards. McDowell and Bogert [Reference McDowell and Bogert110] argued that snakes were related to anguimorphs, particularly varanids and/or lanthanotids, and several cladistic analyses (e.g., [Reference Lee115, Reference Lee116, Reference Paparella, Palci, Nicosia and Caldwell123, Reference Pierce and Caldwell124, Reference Lee129]) supported this (snake–mosasaurian relationships notwithstanding). However, convergence between snakes and other limbless squamates (e.g., amphisbaenians, dibamids, limbless scincids, and anguids) tends to confound analyses based solely on phenotypic data (e.g., [Reference Gauthier, Kearney, Maisano, Rieppel and Behlke6, Reference Conrad59, Reference Lee116, Reference Simões, Caldwell and Kellner130]), leading to artificial groupings. Molecular and combined evidence analyses avoid this problem, and unite Serpentes, Anguimorpha, and Iguania within Toxicofera (e.g., [Reference Vidal and Hedges1]). Most analyses find Serpentes + [Iguania + Anguimorpha] (e.g., [Reference Vidal and Hedges1, Reference Pyron, Burbrink and Wiens8, Reference Zheng and Wiens10, Reference Streicher and Wiens11, Reference Burbrink, Grazziotin and Pyron13, Reference Mulcahy, Noonan and Moss131]) but the sister-group relationship between Iguania and Anguimorpha is not strongly supported. Furthermore, this does not resolve the position of mosasaurians with respect to snakes, because their common placement within an extended Anguimorpha (e.g., [Reference Lee115, Reference Lee116, Reference Paparella, Palci, Nicosia and Caldwell123, Reference Pierce and Caldwell124, Reference Lee132]) is incompatible with the molecular results. Pyron [Reference Pyron12] ran combined evidence analyses using either the matrix of Conrad [Reference Conrad59] or that of Gauthier et al. [Reference Gauthier, Kearney, Maisano, Rieppel and Behlke6] for the morphological component. Predictably, he obtained very different results. His optimal tree for the Conrad matrix placed mosasaurians as sister to varanids (i.e., within Anguimorpha) and only distantly related to snakes, whereas the equivalent tree for the Gauthier et al. [Reference Gauthier, Kearney, Maisano, Rieppel and Behlke6] matrix placed mosasaurians as the sister group of snakes, as did Reeder et al. [Reference Reeder, Townsend and Mulcahy9] (see Augusta et al. and Zaher et al., this volume, for further discussion of snake and mosasaurian relationships).
The first unequivocal snake fossils are isolated vertebrae from the Lower Cretaceous of Algeria (Albian–Cenomanian [Reference Hoffstetter133, Reference Cuny, Jaeger, Mahboubi and Rage134]), reportedly from both terrestrial (Lapparentophis sp.) and aquatic taxa [Reference Rage and Escuillié135], and isolated vertebrae referred to ‘Coniophis ’ sp. from North America (terrestrial, Aptian–Cenomanian [Reference Gardner and Cifelli136]). A much more extensive suite of snake fossils is recorded from the Cenomanian. This Cenomanian record is dominated (and biased) by aquatic taxa from the widespread shallow marine deposits of this period, notably the simoliophiids (or pachyophiids; see Zaher et al., this volume): Simoliophis spp. (France, Portugal, Egypt, Morocco, western Europe and North Africa [Reference Rage and Escuillié135, Reference Rage and Dutheil137]), Pachyophis woodwardi (Bosnia-Herzegovina [Reference Lee, Caldwell and Scanlon138]), Pachyrhachis problematicus (Palestine [Reference Haas139–Reference Zaher and Rieppel142]); Eupodophis descouensi (Lebanon [Reference Rage and Escuillié143]), and Haasiophis terrasanctus (Palestine [Reference Tchernov, Rieppel, Zaher, Polcyn and Jacobs144]), as well as the enigmatic aquatic Lunaophis aquaticus (Venezuela [Reference Albino, Carillo-Briceno and Neenan145]). The current Cenomanian record of terrestrial snakes is more limited, but this is likely to be a gross underestimate considering the global distribution of these taxa: Pouitella pervetus (France [Reference Rage146]), Xiaophis myanmarensis (Myanmar [Reference Xing, Caldwell and Chen147]), Najash rionegrina (Argentina [Reference Apesteguía and Zaher148, Reference Garberoglio, Apesteguia and Simões149]), Seismophis septentrionalis (Brazil [Reference Hsiou, Albino, Medeiros and Santos150]), and Norisophis begaa (Morocco [Reference Klein, Longrich, Ibrahim, Zouhri and Martill151]).
Snakes had clearly diversified, both ecologically and geographically, by the mid-Cretaceous. This is consistent with molecular divergence estimates that place the separation of stem snakes from other toxicoferans during the Jurassic (e.g., [Reference Zheng and Wiens10, Reference Pyron12]). Nonetheless, there remains a significant gap in the fossil record. Filling this gap, Caldwell et al. [Reference Caldwell, Nydam, Palci and Apesteguia30] re-interpreted specimens referred to the Middle Jurassic–Early Cretaceous lizard Parviraptor [Reference Evans27] as stem snakes. Based only on jaw fragments, Caldwell et al. [Reference Caldwell, Nydam, Palci and Apesteguia30] named Eophis underwoodi (Middle Jurassic, UK, dentary symphysis), Diablophis gilmorei (Upper Jurassic, Morrison Formation, USA, maxilla), and Portugalophis lignites (Upper Jurassic, Portugal, maxilla). However, this revision excluded most of the non-dental elements originally attributed to Parviraptor [Reference Evans27], based on the claim that Parviraptor as originally described was a chimaera of several different lizard taxa. New associated parviraptorid material from the Middle Jurassic of Skye confirms the original attribution of elements, both the preserved association on the Lower Cretaceous holotype block and the inferred association from Kirtlington (Middle Jurassic). These elements include paired frontals and parietals, the latter enclosing a parietal foramen, short palatines, and vertebrae that are notochordal and amphicoelous in immature individuals, becoming procoelous with maturity [Reference Evans27]. Work on the new Skye material and other parviraptorids is ongoing but Parviraptor as originally diagnosed is not a chimaera, and phylogenetic analyses must therefore include all of the attributed skeletal elements, rather than selecting only those (maxillae, dentaries) consistent with a particular hypothesis. Preliminary analyses (work in progress) do not support the inclusion of parviraptorids within Pan-Serpentes, and the Middle Jurassic age of the first recorded parviraptorid should therefore not be used to date snake origins in molecular divergence estimates (e.g. [Reference Harrington and Reeder152]).
2.4 Discussion and Conclusion
Although the squamate stem probably extended back into the Early Triassic, morphologically diagnosable squamates are currently unknown prior to the Middle Jurassic of Eurasia and North Africa. Molecular divergence estimates predict that major squamate clades had arisen by this time but, as yet, none of the currently known Middle Jurassic squamates can be placed confidently into the crown. A few Late Jurassic taxa, notably the dorsetisaurs (Anguimorpha), the paramacellodids (Scincoidea), and perhaps Eichstaettisaurus (Gekkota), may lie on the stems of crown clades, but the first unequivocal snake fossils currently date from the mid Cretaceous. The near global distribution of both terrestrial (France, USA, Gondwana) and aquatic (mostly Tethyan) snakes by the Cenomanian provides strong evidence of an earlier origin, but recent reports of Jurassic snake fossils [Reference Caldwell, Nydam, Palci and Apesteguia30] are based on misconception (see above). Given the close relationship between iguanians, anguimorphs and snakes, as strongly supported by molecular data, stem iguanians and stem anguimorphs have the potential to shed light on the expected morphology of the earliest stem snakes – given that stem toxicoferans presumably resembled one another morphologically until they diverged. Taxa like the dorsetisaurs, Huehuecuetzpalli , Hoyalacerta , and perhaps Parviraptor may therefore provide insights into early toxicoferan morphology, and may help to refine ideas on ancestral traits (e.g., [Reference Da Silva, Fabre and Savriama153]). Moreover, if mosasaurians (in total or in part) are genuinely the sister group of snakes (but see Augusta et al. and Zaher et al., this volume), then stem members of that clade also need to be identified, probably from terrestrial and/or freshwater deposits.
Further progress in unravelling the early history of squamates generally, and snakes in particular, will therefore require a combination of new fossil material; accurate (and objective) re-description of existing material using CT scan technology where possible; and a concerted effort to recover early squamate material from biogeographically and ecologically diverse deposits. Unfortunately, most Jurassic and Early Cretaceous squamate taxa are phylogenetically unstable when analysed in matrices combining living and extinct taxa. The topographic position of Mesozoic squamates is sensitive to analysis variables like data input (character choice and definition, ingroup and outgroup taxa sampled, incorporation of temporal data), and the use of ordering, weighting, or molecular constraints. Moreover, apomorphic skeletal features that characterize individuals of modern clades may be absent in early or stem representatives of those clades, further complicating their phylogenetic placement, especially when incomplete. Improving tree resolution is not simply a question of adding more phenotypic characters. We need to develop a greater understanding of those characters, and of the developmental and functional relationships between them.