Introduction
Medico-legal entomology represents a subsection of forensic entomology that uses insects to understand information surrounding a death, including the location of openings in the soft tissue (such as potential wounds), toxicological analysis, postmortem body movement, and, most importantly, the estimation of a minimum postmortem interval (Amendt et al. Reference Amendt, Richards, Campobasso, Zehner and Hall2011; Rivers and Dahlem Reference Rivers and Dahlem2014). Blow flies (Diptera: Calliphoridae) are arguably the most valuable insects within the necrophagous community based on their early colonisation behaviour and known developmental rates (Gennard Reference Gennard2012; Rivers and Dahlem Reference Rivers and Dahlem2014; Byrd and Tomberlin Reference Byrd and Tomberlin2020). Region-specific data on blow flies are valuable in all areas in which forensic entomology is used to provide baseline data for comparisons with crime-scene collections (Sanford Reference Sanford2017). The presence of species on a cadaver that are not typical to a region, area, or season may suggest the cadaver has been moved (Anderson Reference Anderson2015). As well, timing of gravidity is important in the early period of the insect season because this information helps to establish the start of the annual reproductive range for a species, which may occur after the adult becomes physically active (Denlinger Reference Denlinger2002; Rivers and Dahlem Reference Rivers and Dahlem2023).
Estimating the reproductive cycles of wild female blow flies is a challenge in forensic entomology. Environmental temperature influences the active and reproductive timing of flies, forming temporal ranges through an annual period (Saunders and Gilbert Reference Saunders and Gilbert1990; Numata and Shiga Reference Numata and Shiga1995). Many environmental cues, including increasing temperatures, promote emergence from diapause, with blow flies often becoming active in early spring but with reduced metabolic functioning (Wilde Reference Wilde1954; Denlinger Reference Denlinger2002; Rivers and Dahlem Reference Rivers and Dahlem2014) until temperatures have stabilised above a species-specific threshold, generally ranging from 0 to 10 °C, when females resume full metabolic activity and become sexually mature and capable of oviposition (Wilde Reference Wilde1954; Denlinger Reference Denlinger2002; Ames and Turner Reference Ames and Turner2003). This means that some species of blow flies may be present in early spring and collected as adults at crime scenes but may not yet be capable of oviposition.
Although some species of blow fly are relatively ubiquitous in North America, such as Phormia regina (Meigen) (Diptera: Calliphoridae) (Anderson Reference Anderson1995), many species exhibit distinct resource partitioning. Some species are found primarily in rural areas, such as Calliphora vomitoria (Linnaeus) (Hwang and Turner Reference Hwang and Turner2005), depending on naturally available carrion, whereas others are found primarily in urban environs, such as Lucilia sericata (Meigen) and Calliphora vicina Robineau-Desvoidy (Reiter Reference Reiter1984), having evolved to associate with human food waste and roadkill. However, these patterns can vary geographically, with more rural species being found in urban areas (Grassberger and Frank Reference Grassberger and Frank2004) and vice versa; it is therefore important to understand what species inhabit specific areas, especially because, as ranges change with global warming, previously understood ranges may change (Amendt Reference Amendt2021). In a baited trap study across Canada in urban and rural areas over a three-year period, a greater number and diversity of blow flies were caught in rural versus urban areas, and habitat was found to be more influential than ecozone (Langer et al. Reference Langer, Kyle, Illes, Larkin and Beresford2019). In England, habitats from the urban centre of London through suburbs and into rural grass and woodlands were surveyed using baited traps and showed that habitats could be separated based on blow fly species (Hwang and Turner Reference Hwang and Turner2005). Understanding blow fly habitat preferences is forensically valuable; for example, if species are found on remains outside their normal habitat, this potentially suggests that the body has been relocated to a second scene (Catts and Haskell Reference Catts and Haskell1990; Anderson Reference Anderson2020).
The goals of the present study were to understand Calliphoridae species composition in three regions (urban, rural, and mixed environments) in Metro Vancouver, British Columbia, Canada over a nine-month period and the timing of first attraction and first oviposition at the start of the insect season. In addition, we wished to ascertain whether adults collected in the traps were representative of those attracted to the trap by determining whether eggs were laid in the bait by species that were not caught as adults.
Materials and methods
Description of study sites
This study was conducted over a nine-month period within Metro Vancouver, British Columbia, Canada using three different locations. The Metro Vancouver area lies within the Coastal Western Hemlock biogeoclimatic zone (Pojar et al. Reference Pojar, Klinka and Demarchi1991). Locations were chosen to represent urban, rural, and mixed environments. Location 1 (“BP”) was in an urban residential area (Birkdale Place) in Burnaby, British Columbia (49.2714 N, –122.9423 W) at 103 m above sea level, consisting of a residential backyard that opens onto trees, shrubbery, and a stream (Fig. 1). Location 2 (“SFU”) was in a mixed urban–rural area on the Simon Fraser University, Burnaby campus (49.2758 N, –122.9148 W) at 330 m above sea level. Surroundings were a forested landscape with shrubbery; however, the trapping location was in an outdoor, fenced compound away from surrounding flora, and high-noise-level construction was being performed nearby. This site includes both urban and rural components, with a large human population and buildings, both professional and residential, but it is surrounded by forest (Fig. 2). Location 3 (“Rural”) was in a rural area of Langley, British Columbia (49.1019 N, –122.5435 W) at 90 m above sea level and consisted of a field and wooded landscape that were surrounded by similar properties on acreage (Fig. 3).

Fig. 1. The BP site, with one of the bottle traps in place.
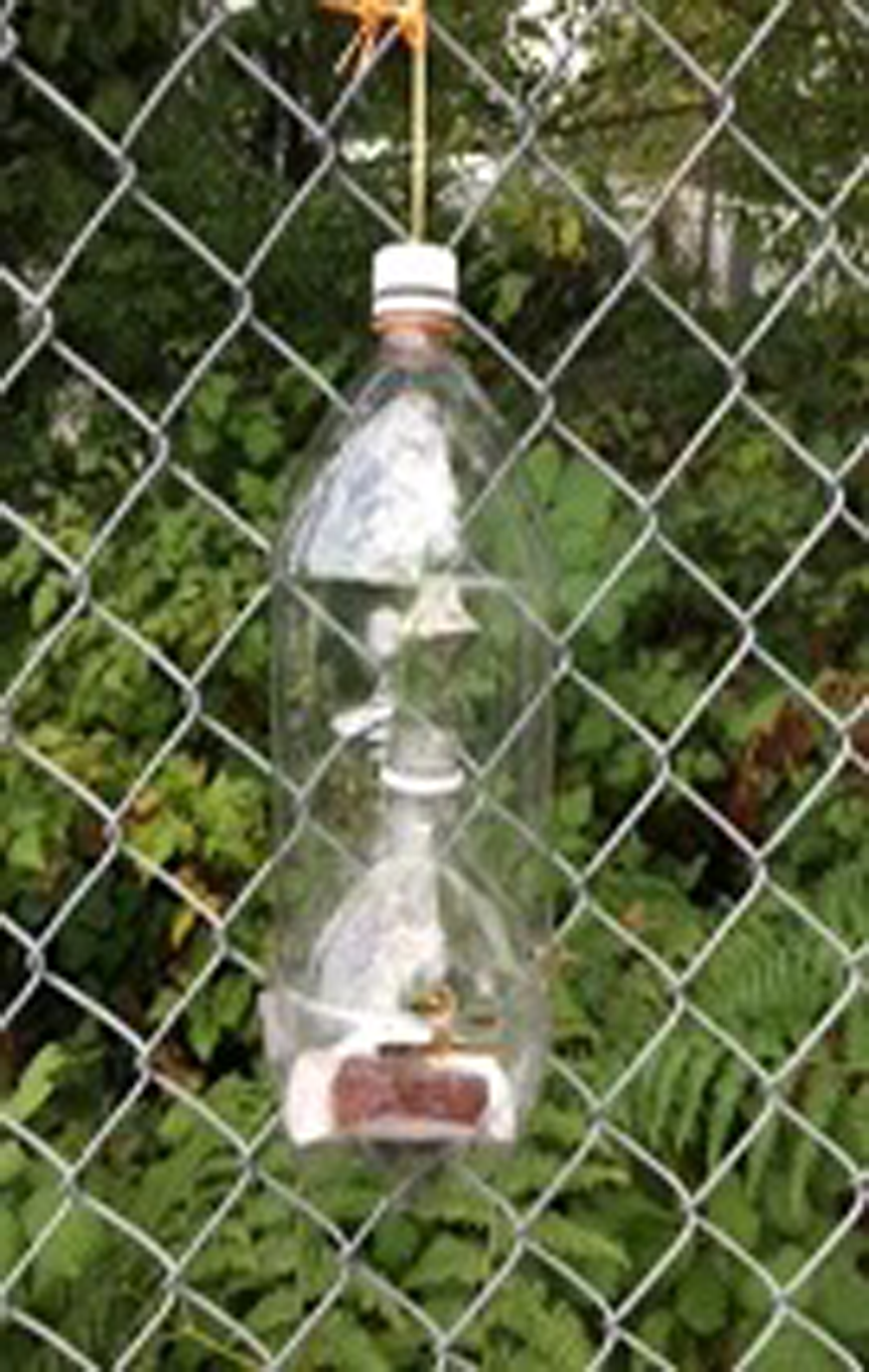
Fig. 2. The SFU site, with one of the bottle traps in place.

Fig. 3. The Rural site, with one of the bottle traps in place.
Bottle trap design
Modified bottle traps were built to capture blow flies based on a design by Hwang and Turner (Reference Hwang and Turner2005; Fig. 4). Each trap was constructed using two 2-L clear plastic cylindrical bottles. Approximate trap dimensions were a height of 28 cm, a circumference of 34.5 cm, and a diameter of 11.3 cm. To create a trap, one bottle was cut horizontally to produce two pieces (a 24-cm top piece, including the bottle cap, and 4-cm bottom piece), which formed the top and bottom of the trap. Using a scalpel, two 2-cm × 2-cm slices were cut diagonally into the bottom piece on opposite sides to form an “X” shape. These cut pieces were pushed inside the bottle to form a square tunnel. A 9- to 10-cm-wide and 3.5-cm-high opening (without removing the plastic) was cut in the same 4-cm-long bottom piece, and strips of Velcro® were stuck to the outside to form a sealing door for the placement of beef liver. A second bottle was cut horizontally at 10 cm from the top, and the bottle cap was removed to produce a funnel-shaped piece that was placed inside the two pieces of the first bottle. All the bottle pieces were joined together using metal paper fasteners. A 45-cm looped piece of string was tied to the bottle neck for hanging. This collection method was chosen because of its convenience to analyse necrophagous community composition during a short period, the ability to easily place and remove it in multiple locations, and its inexpensive production.

Fig. 4. A, Bottle trap used to catch necrophagous and necrophilous arthropods in Metro Vancouver area sites, March–December 2019. Trap is baited with bovine liver on filter paper. B, Trap design was modified from that used by Hwang and Turner (Reference Hwang and Turner2005).
Field experiment design
Nine traps were used weekly for the study duration, three per location (BP, SFU, and Rural), acting as a single sample for each location to maximise catch number and record diversity. Traps were baited with a 35-g (± 2 g) piece of beef liver placed on a quantitatively calibrated (90-mm) piece of filter paper. Beef liver was purchased from Surrey Meat Packers, Surrey, British Columbia and was kept frozen until needed in a –20 °C freezer. On the day before collection, frozen beef liver was placed in a refrigerator to thaw. Traps were deployed once per week on optimal weather days (minimal precipitation or wind) for a 12-hour period from 07:00–08:00 to 07:00–08:00 (or until sundown; local time) from 5 March 2019 to 2 December 2019, accumulating a total of 40 trapping days. Traps were hung approximately 1.2 m above the ground using available structures (tree branches or fencing) to minimise predator disturbance and were spaced at least 6 m apart. Placement locations were chosen to maximise the amount of sunlight reaching the trap. At the end of a trapping interval, trap entrances were blocked with paper towel and were transported to the laboratory for analysis.
Storage protocols
In the lab, beef liver containing eggs was removed from the traps for rearing. Once removed, traps containing adult specimens were placed in a –20 °C freezer for 24 hours for killing. Specimens were removed from the freezer, air-dried for 1–2 days to minimise moisture and mould growth, and stored by trap (number and location) in loosely sealed, 80-mL sterile vials.
Larval rearing
All eggs laid in the traps from 1 July 2019 to 2 December 2019 were reared to adulthood. Rearing jars were filled with a 3- to 4-cm-deep base layer of sawdust to mimic soil for pupariation and were sprayed with water to create a slightly moist environment. The piece of filter paper containing the beef liver and eggs was placed on top of the sawdust base, and a fresh piece of beef liver, approximately 35 g, was added. The jars were sealed using a double layer of paper towel and elastic bands and were monitored once per day, adding additional liver as needed. Following pupariation, the beef liver and filter paper were removed from the jar. After adult emergence from pupal cases, jars were left until all specimens had died and then were transferred to a –20 °C freezer for a 24-hour period to ensure death. Reared flies were stored following the same procedures used for captured specimens.
Temperature and rainfall data collection
Temperature (°C) and rainfall (mm) were monitored using historical weather archives provided by two databases with the highest data availability and closest monitoring stations. The National Oceanic and Atmospheric Administration provided data for the Rural and SFU locations. The Rural location used data from the “Fort Langley Telegraph Trail, Environment Canada” weather station (49.15 N, –122.55 W), situated 76 m above sea level with 93% data coverage. The 366-m-above-sea-level “Burnaby Simon Fraser U, Environment Canada” weather station (49.2833 N, –122.9167 W) was used for the SFU location, with 90% data coverage. Environment Canada provided data for the BP location through the CCN-operated “Port Moody Glenayre” weather station (49o 16′ 45″ N, 122o 52′ 53″ W), at 129.50 m above sea level. The influence of mean temperature and precipitation on species composition was measured over time using descriptive statistics and bivariate analyses. As a result of using weather data, temperature and precipitation values were not available for all trapping days in certain locations, resulting in a total of 141 specimens missing temperature data over 11 trapping days and 91 specimens missing precipitation data over six trapping days.
Insect identification
All specimen identification was performed using a Leica EZ4 dissecting microscope (Wetzlar, Germany). Collected and reared specimens were identified to order (Millar et al. Reference Millar, Uys and Urban2000). Dipteran specimens were identified as Calliphoridae or non-Calliphoridae (Jones et al. Reference Jones, Whitworth and Marshall2019), with Calliphoridae identified to species. A subsample of Calliphoridae specimens was sent to Dr. Terry Whitworth (Washington State University, Pullman, Washington, United States of America), an expert in Calliphoridae identification, for confirmation of species identification. Specimens were sexed using two methods: (1) distance between the eyes and (2) examination of the genitalia (Jones et al. Reference Jones, Whitworth and Marshall2019). Females from traps were dissected to determine gravidity at the time of capture, based on the presence of eggs in the abdominal cavity.
Statistical analyses
All traps from each location were treated as a single sample to allow discussion of each location as a whole unit. Descriptive statistics assessed the distribution and normality of all variables. Skewness and kurtosis values indicated that all variables, except for precipitation, fell within the acceptable range (–2 to 2) of normality. Chi-square tests were performed to assess significant changes (P < 0.05) in the species ID variable against the variables of seasonal variation (month), geographic variation (location), sex, and gravidity within and between all trapping locations. The relationships between sex and trap location, trap number, collection date, and month were assessed using Chi-square tests, and one-way analyses of variance were run to understand the relationship between sex and temperature (minimum, maximum, and mean). The same bivariate analyses described for the variable of sex were conducted to assess the relationship of gravidity with all previously defined variables.
For significant differences (P < 0.05) obtained in Chi-square analyses, adjusted standardised residuals were measured to determine z-scores, and Bonferroni’s correction was applied to obtain an adjusted P-value to account for Type I error. For one-way analyses of variance, a Tukey’s test was performed, and pairwise comparisons were used for Kruskal–Wallis. The Shannon–Weiner Diversity Index (H) and Shannon Equitability Index (EH; measured on a scale of 0–1) were calculated manually to describe the diversity and evenness of species over time, with an E H value of 1 representing complete evenness of species’ abundance within a population.
Using Microsoft™ Excel, version 16.54 software, Hutcheson t-tests were performed to compare Shannon–Weiner Diversity Indices between all trapping months and between all trapping locations. Using reared Calliphoridae specimens, the rate of escape of female blow flies was calculated manually to understand the effectiveness of the trap design. Sex ratios were calculated manually for Calliphoridae from dominant species (those with more than 50 collected specimens) to understand sex disparities of early colonisers, and gravidity ratios were calculated to assess differences in gravidity between locations and across time. Unless stated otherwise, statistical analyses were performed using IBM SPSS® Statistics, version 24 software (https://www.ibm.com/products/spss-statistics).
Results
Seasonal and geographical trends
A total of 1334 specimens (n = 1334) from 22 taxa (Table 1) were collected from all traps. Table 1 includes Calliphoridae and also all collected noncalliphorid Diptera, such as Pollenia spp., as well as Arachnida, Coleoptera, Hemiptera, Hymenoptera, and Lepidoptera. Diptera were most abundant (77.4%; n = 1033), followed by Hymenoptera (22.1%; n = 295) and Coleoptera (0.2%; n = 3), whereas Hemiptera (0.1%; n = 1), Lepidoptera (0.1%; n = 1), and arachnids (0.1%; n = 1) were rarely collected.
Table 1. Species composition of adult specimens collected from all trapping locations, March–December 2019, in three locations, Rural, SFU (mixed rural/urban), and BP (urban), in Metro Vancouver, British Columbia, Canada. Genus and species reported for Calliphoridae (Diptera). All others identified to higher taxonomic levels.

Of the dipteran specimens, 69.8% (n = 721) were Calliphoridae (and closely related Pollenia spp.), representing 16 taxa, and the rest (30.2%) were non-Calliphoridae Diptera (n = 312). Pollenia specimens were included within Calliphoridae; however, only six specimens were collected. Within the collected Calliphoridae, Calliphora vicina (Robineau-Desvoidy) (34.95%; n = 252), Lucilia sericata (Meigen) (24.41%; n = 176), Calliphora latifrons Hough (19.69%; n = 142), and Lucilia illustris (Meigen) (11.79%; n = 85) were dominant species. Large numbers of hornets and yellowjackets (Hymenoptera: Vespidae) were captured in the traps and were frequently observed killing and eating adult Diptera as well as eggs. In many cases, parts of insects were also recovered in the traps, indicating predation.
Although trapping was conducted from 5 March 2019 to 2 December 2019, insects were collected in the traps only from 18 March to 19 November 2019, indicating the active insect period. Total species abundance changed seasonally and regionally (Fig. 5), with the highest abundance observed in the summer months, from June to September, and at the Rural location.

Fig. 5. Frequency of adult specimen collection from all taxa across monthly intervals in three locations, Rural, SFU (both mixed rural/urban), and BP (urban), in Metro Vancouver, British Columbia, Canada.
A statistically significant relationship (χ2 = 755.299, df = 168, P = 0.000, Cramer’s V = 0.266) between species ID and month indicated that species composition shifted over time, with differential proportions of species contributing to the observed monthly abundance. Analysis within each trapping location also highlighted significant (P < 0.05) differences, indicating that species composition of Calliphoridae shifted within each environment over time (Figs. 6 and 7). A bimodal curve was observed for C. latifrons (Fig. 6A), not only showing peak capture levels in June (n = 31) and September (n = 27) but also generating a significant proportion of the collected abundance in May. Similar results were found for C. vicina (Fig. 6B), with peak capture levels occurring in June (n = 55) and September (n = 51) and peak populations between trapping locations occurring in other months. Calliphora vomitoria (n = 6; Fig. 6C) was captured only in the spring (April, May) and fall months (September, October). Similarly, Cynomya cadaverina Robineau-Desvoidy (Diptera: Calliphoridae) (n = 18; Fig. 6D) was collected largely in the fall. Capture of L. illustris (n = 85; Fig. 7A) and L. sericata (n = 176; Fig. 7B) peaked in August. Hymenopterans (n = 295; Fig. 7C), consisting of yellowjackets and hornets (Vespidae), were collected throughout the study, with elevated capture occurring in the summer months and peaking (n = 118) in August. Non-Calliphoridae Diptera (Fig. 7D) were commonest at the Rural site. Overall, species were found to have preferred periods of annual activity, which created unique species compositions between seasons.

Fig. 6. Abundance of adult A, Calliphora latifrons, B, Calliphora vicina, C, Calliphora vomitoria, and D, Cynomya cadaverina over time in three locations, Rural, SFU (both mixed rural/urban), and BP (urban), in Metro Vancouver, British Columbia, Canada.

Fig. 7. Abundance of adult A, Lucilia illustris, B, Lucilia sericata, C, Hymenoptera, and D, non-Calliphoridae Diptera over time in three locations, Rural, SFU (both mixed rural/urban), and BP (urban), in Metro Vancouver, British Columbia, Canada.
Of the 1334 specimens collected, more than half (52.2%; n = 697) were recorded at the Rural site. The remaining specimens were closely divided between the BP (urban; 27%; n = 360) and SFU (mixed rural/urban; 20.8%; n = 277) sites. Each trapping location had a distinct species composition (Table 1), with differing dominant species that shifted over time. Analysis of location and species demonstrated a statistically significant relationship (χ2 = 281.351, df = 42, P = 0.000, Cramer’s V = 0.325), implying that the overall species composition of at least two trapping locations differed. Post hoc tests identified significant (P < 0.000757) differences in the abundance of C. latifrons, C. vicina, L. illustris, L. sericata, other Diptera, and Hymenoptera between trapping locations relative to the overall abundance from each location.
At the Rural location, 15 groups were identified from the 697 specimens collected. The dominant group was “Other Diptera” (n = 204), which represented 29.3% of specimens, and the dominant Calliphoridae species was L. sericata (n = 150; 21.5%). Post hoc testing revealed that Lucilia sericata, L. illustris, and other dipteran species exhibited higher densities (n = 150, n = 70, and n = 204, respectively) at the Rural site than their combined totals collected from the other trapping locations. Calliphora vicina was found in high abundance at all locations, with the largest proportion (38.1%; n = 96) captured rurally. Post hoc testing, however, revealed a higher percentage of C. vicina at the BP and SFU locations. Calliphora vomitoria was collected in equal abundance at all locations but in low numbers. However, this is not surprising because C. vomitoria is known to prefer larger carcasses and therefore is probably less attracted to the small baits used in this study (Davies Reference Davies1990; Reference Davies1999; Greenberg and Tantawi Reference Greenberg and Tantawi1993).
At the BP location, 17 groups were identified from the 360 specimens collected. Hymenopterans (n = 107) were the dominant group, comprising 29.7% of collected insects. Hymenoptera were collected at high frequencies at all locations, with the greatest proportion (36.3%; n = 107) captured at the BP location. At 22.5%, C. vicina (n = 81) was the dominant Calliphoridae species.
The SFU location yielded 277 specimens from 14 identified groups. With 31.4% of the total, Hymenoptera (n = 87) were the most abundant, whereas C. vicina (n = 75; 27.1%) was the dominant Calliphoridae species. The highest proportion of C. latifrons (38%; n = 54) was collected from the SFU location.
Effect of temperature and precipitation on trap capture rates
Table 2 shows the wide scope of mean temperatures observed over the duration of this study, ranging from 5.8 to 23.0 °C, with a mean value of 16.8 °C when considering all sites. Examination of mean temperatures at each trapping location using a one-way analysis of variance revealed statistically significant differences (F = 5.490, df = 2, P = 0.004), indicating that mean temperatures differed between at least two of the sites. Normality was assumed for the variables based on skewness and kurtosis values, but Levene’s test was determined to be significant (P = 0.000); therefore, homogeneity of variances could not be assumed. To counteract this violation of assumptions, a nonparametric Kruskal–Wallis test was performed on the variables, showing a statistically significant (χ2 = 22.115, df = 2, P = 0.000) result between the variables. A post hoc analysis revealed that the mean temperature significantly (P < 0.05) differed between the SFU and Rural sites (P = 0.003) and between the SFU and BP sites (P = 0.000) but did not significantly differ between the Rural and BP sites (P = 0.065). Over the duration of the study, the lowest temperatures, according to mean ranks, were recorded at the SFU location (Table 2), ranging from 0 to 25 °C, whereas the warmest temperatures were observed at the BP location (Table 2), ranging from 2.7 to 28.8 °C. Bivariate analyses of species ID (χ2 = 171.202, df = 21, P = 0.000) and month of collection (χ2 = 786.589, df = 8, P = 0.000) revealed similar trends, with both variables showing a statistically significant relationship to mean temperature. This result indicates that there is a difference in mean temperature observed between some of the months of collection, with the lowest recorded mean temperature observed in November and highest in July, followed by August, according to mean ranks. Post hoc testing revealed that all months showed significant (P < 0.05) differences in mean temperatures between them, with the exception of May and July (P = 0.753), September and June (P = 0.140), and September and March (P = 0.615). When examining these findings in relation to the recorded abundance of collected specimens across months, a similar pattern is observed, where the highest abundance is recorded for the high mean temperature months of July and August, compared with lower abundance in the colder mean temperature months of March, April, and November. The correlation of these two variables with recorded abundance of necrophagous insects indicates that mean temperature is likely a key influencer of seasonal variation of the necrophagous community. For the variable of species ID, the statistically significant result indicates that different species are more likely to be collected at different mean temperatures. Post hoc testing revealed that the majority of species had differences in mean temperature during capture. However, the dominant species, L. sericata and L. illustris, did not present significant differences (P = 0.580) in mean temperature, indicating that these species likely have similar temperature requirements. Examination of the differences in ranks of dominant Calliphoridae species revealed that C. vicina was recorded most often at lower mean temperatures, followed by C. latifrons, L. sericata, and L. illustris at the highest mean temperature of these four species. This result, in conjunction with the observed activity range (Table 2), indicates that Calliphora species are better adapted to cold environments than Lucilia species.
Table 2. Temperature data for March–November 2019.

Weather station data were used to determine the precipitation values for collection days at each site. However, the weekly collection days were chosen based on the absence of rainfall, when possible, to increase capture rate. As a result of this preferential selection, the results were skewed towards collection on days that accumulated no precipitation, with skewness and kurtosis values that exceeded the ranges for a normally distributed variable. Therefore, although the expected trend occurs, with lower collected abundance of specimens during higher periods of rainfall, determining from the data whether this trend has been tailored based on the choice of the researcher is not possible. Overall, this measure introduced a biased result for the variable of precipitation, making it difficult to accurately explain the influence of rainfall on trap capture rate. For this reason, the relationship between precipitation and early carcass colonisation was not analysed further.
Reproductive range of Calliphoridae species
More nongravid females were recorded at the SFU location than at the BP and Rural locations (Table 3). A higher ratio of nongravid females was collected at the beginning of the year (March, April) than in later months.
Table 3. Gravidity ratios of female Calliphoridae captured over time in three locations (Rural, SFU (mixed rural/urban), and BP (urban)) in Metro Vancouver, British Columbia, Canada.

Only significant (P < 0.05) variables were assessed for gravidity ratios. Date of collection was not included because overall collection from a single trapping day was often minimal and not accurately representative. Gravidity ratios indicate the number of non-gravid females per 100 gravid.
Active start date (when a male or female is first collected, regardless of gravidity) and reproductive start date (when a gravid female is first collected) within dominant species of Calliphoridae were compared (Table 4). Lucilia illustris and C. vicina exhibited the same range for activity and reproduction. Active and reproductive ranges for C. latifrons and L. sericata differed, with reproductive start dates (8 April 2019 and 29 April 2019, respectively) beginning later in the year than active start dates (25 March 2019 and 1 April 2019, respectively).
Table 4. Active and reproductive ranges of Calliphoridae from dominant species collected, March–December 2019, in three locations, Rural, SFU (both mixed rural/urban), and BP (urban), in Metro Vancouver, British Columbia, Canada.

* Active range applies to both males and females of the species.
†Reproductive range refers only to females of the species.
Trap escape rates
A total of 5218 blow flies were reared to the adult stage from the eggs laid on the baits and consisted of five identifiable species (C. latifrons, C. vicina, C. cadaverina, L. illustris, and L. sericata). Findings showed a higher likelihood of C. vicina (n = 1995; 38.23%) and L. sericata (n = 1684; 32.27%) oviposition than in other collected Calliphoridae species. Differences in species composition observed between trapped adult females and eggs reared during a trapping interval indicated escape following oviposition. We suspected that many flies were escaping from the traps, as evidenced by species raised from eggs, when no adults of the hatched species were collected on that date. There was no method to determine whether males escaped, but comparison of egg masses with captured females could be made. We recorded the species of blow flies reared from each trap on each trapping date and correlated this with whether an adult female was collected in that trap on that date. If even a single female of that species was collected in that trap on that date, then no escape was noted. However, if, for example, C. vicina were reared from eggs in the trap but no C. vicina adult female specimen was collected during that day from that trap, then it was noted that escape occurred. To get our total escape rates for each species, these data were compiled over all the days, and averages were calculated. This clearly underestimates possible escape because the trap may have collected several females of that species that laid eggs and then escaped, but if only one was present, we marked that as “no escape”, and male escape could not be assessed. Analyses of these data (Table 5) revealed a high rate of female escape (35.45%) for all examined species, with the highest rates of escape found in C. cadaverina (66.66%) and C. latifrons (60.00%) populations.
Table 5. Estimated escape rate of female blow flies from traps July–December 2019, in three locations, Rural, SFU (both mixed rural/urban), and BP (urban), in Metro Vancouver, British Columbia, Canada, based on the presence of identified Diptera collected as eggs versus adult female specimens during a trapping interval.

* Unknown specimens refer to all flies that could not be identified to species. Groups were defined based on the species captured within a specific trap during a trapping interval. For example, Calliphora latifrons reared from a trap on 1 July 2019, with the designator “Rural 2” acted as a singular group.
Of the collected blow flies and cluster flies (n = 721), 695 intact specimens were sexed, with 81.7% (n = 568) identified as female and 18.3% (n = 127) as male, indicating that most blow flies attracted to the bait were female.
Discussion
Seasonal and geographical trends
Each environment provides a unique species composition of Calliphoridae that shifts over time based on changing climatic conditions and insect adaptations, which can impact entomological evidence assessed in criminal investigations. Before our study, little information was available regarding the effects of seasonal variation on Calliphoridae community composition during early colonisation within the Lower Mainland of British Columbia, Canada. This area is within the Coastal Western Hemlock biogeoclimatic zone (Meidinger and Pojar Reference Meidinger and Pojar1991) and is, in itself, different from other biogeoclimatic zones in British Columbia and Canada (Anderson and VanLaerhoven Reference Anderson and VanLaerhoven1996; LeBlanc and Strongman Reference LeBlanc and Strongman2002; Sharanowski et al. Reference Sharanowski, Walker and Anderson2008).
Analysis of total species composition in the present study demonstrated a typical pattern of attraction to carrion, with necrophagous or necrophilous Diptera collected most often, followed by Hymenoptera and Coleoptera. Necrophagous Diptera are often the first to colonise a body in the early stages of decomposition, followed sequentially by coleopterans and hymenopterans (Catts and Goff Reference Catts and Goff1992; Carvalho et al. Reference Carvalho, Thyssen, Goff and Linhares2004; Goff Reference Goff2009; Byrd and Tomberlin Reference Byrd and Tomberlin2020), although some hymenopterans may arrive very early to feed on the carcass and on Diptera eggs. In the Diptera, the Calliphoridae are among the earliest to be attracted to carrion, arriving within minutes of death (Catts and Goff Reference Catts and Goff1992; Carvalho et al. Reference Carvalho, Thyssen, Goff and Linhares2004). This finding held true in our study, with Calliphoridae representing 69.8% of dipteran specimens. This is similar to a United Kingdom study in which 78.6% of Diptera collected in baited traps across habitats were Calliphoridae (Hwang and Turner Reference Hwang and Turner2005). Although studies highlight environmental diversity, the majority of collected samples often include three to four key species (Schroeder et al. Reference Schroeder, Klotzbach and Puschel2003; Hwang and Turner Reference Hwang and Turner2005; Sabanoğlu and Sert Reference Sabanoğlu and Sert2010; Brundage et al. Reference Brundage, Bros and Honda2011; Benbow et al. Reference Benbow, Lewis, Tomberlin and Pechal2013; Weidner et al. Reference Weidner, Jennings, Tomberlin and Hamilton2015). This finding was also observed in our study, with the majority of Calliphoridae represented by C. vicina, C. latifrons, L. illustris, and L. sericata, which are forensically important species in British Columbia (Anderson Reference Anderson2000). At least nine other Calliphoridae species were encountered, most of which are common and widespread throughout North America, with the exceptions of Calliphora montana (Shannon) and Angioneura spp. (Jones et al. Reference Jones, Whitworth and Marshall2019).
Differences in necrophagous species composition were observed between trapping locations, with the highest abundance observed at the Rural location and highest diversity observed at the BP (urban) location. This is similar to a baited trap study comparing rural and urban areas across Canada over three years, which found that not only were greater numbers of blow fly species collected in rural areas but diversity was much greater, with 14 species found in rural areas and only eight in urban areas (Langer et al. Reference Langer, Kyle, Illes, Larkin and Beresford2019). Geographic locations with plentiful resources tend to have less competition and higher population densities (Denno and Cothran Reference Denno and Cothran1976; Brundage et al. Reference Brundage, Bros and Honda2011). These environments are often rural, where species are less likely to have to compete for space and resources (Vitousek et al. Reference Vitousek, Mooney, Lubchenco and Melillo1997), although urban environments also provide multiple food sources.
At the Calliphoridae species level, we observed differences between locations, indicating that preferences for specific habitat types may exist. Lucilia sericata and L. illustris were classified as rural species, based on higher recorded frequencies at the Rural location. Anderson (Reference Anderson2000) found that L. illustris was commonly detected in rural habitats, agreeing with the current finding; however, Hwang and Turner (Reference Hwang and Turner2005) observed the opposite in the United Kingdom, recording L. illustris in greater numbers in urban areas. Anderson (Reference Anderson2000) also noted that L. sericata was typically captured in urban environments, a finding corroborated by others (Anderson and VanLaerhoven Reference Anderson and VanLaerhoven1996; Hwang and Turner Reference Hwang and Turner2005; Brundage et al. Reference Brundage, Bros and Honda2011; Weidner et al. Reference Weidner, Jennings, Tomberlin and Hamilton2015). The opposite result observed in this study indicates that Lucilia spp. habitat preference may have shifted in Metro Vancouver or that the Rural location has become more urbanised over time. As discussed by Weidner et al. (Reference Weidner, Jennings, Tomberlin and Hamilton2015), the high abundance of L. sericata in urban areas may be based on a preference for high human densities, whereas the Rural site involved properties no smaller than one acre.
Calliphora latifrons was classified as a synanthropic species based on a higher frequency in urban locations. Anderson (Reference Anderson2000) also recorded elevated levels of C. latifrons on the Simon Fraser University campus, indicating preference likely does exist for this urban, higher-elevation habitat. However, C. latifrons has been collected across many habitats, indicating that this species’ spatial range may be largely influenced by environmental conditions or resource availability (Anderson and VanLaerhoven Reference Anderson and VanLaerhoven1996; Brundage et al. Reference Brundage, Bros and Honda2011). Cynomya cadaverina was also collected most frequently at the SFU location in the present study but showed no preference for urban or rural locations. Although C. vicina was collected most frequently at the Rural location, all locations showed high abundances, with C. vicina acting as the dominant species at both urban locations (BP and SFU). This finding indicated that C. vicina prefers urbanised environments. Hwang and Turner (Reference Hwang and Turner2005) have shown C. vicina in higher densities in urban habitats.
Noncalliphorid Diptera comprised a large proportion (23.4%) of our total specimen collection. This finding suggests that more effort may need to be put into identifying other early arriving species because there may be Dipteran species outside of the Calliphoridae that could provide useful information in forensic investigations.
Reproductive range of Calliphoridae species
Carcass colonisation is recognised as a female-driven process for blow flies, used to provide a protein meal for sexual organ development and as a site for oviposition (Norris Reference Norris1965; Janaway et al. Reference Janaway, Percival and Wilson2009; Mohr and Tomberlin Reference Mohr and Tomberlin2014a; Rivers and Dahlem Reference Rivers and Dahlem2014). A female-biased sex ratio was observed across all dominant calliphorid species regardless of geographic variation, seasonal variation, or other tested variables, suggesting that sex differentials during initial attraction may result from calliphorid behaviour rather than environmental influence. Similar sex ratios have been noted in other colonisation studies, highlighting a pattern among blow flies (Baz et al. Reference Baz, Cifrián, Díaz-äranda and Martín-Vega2013; Martin-Vega and Baz Reference Martin-Vega and Baz2013; Mohr and Tomberlin Reference Mohr and Tomberlin2014a, Reference Mohr and Tomberlin2014b). Hwang and Turner (Reference Hwang and Turner2005) recorded a significant disproportionate catch rate, with a calculated sex ratio of 1:23.7 (male:female) for C. vicina. Martin-Vega and Baz (Reference Martin-Vega and Baz2013) confirmed this bias in catch rate within other necrophagous Diptera families, including the Muscidae and Sarcophagidae. They suggested that sex ratios may be influenced in part by male behaviour, with those that use perching behaviour over meeting behaviour experiencing greater bias in female capture rate (Martin-Vega and Baz Reference Martin-Vega and Baz2013).
Blow fly species are known to have unique activity ranges throughout an annual period, based primarily on their preferred temperature range and the process of diapause (Rivers and Dahlem Reference Rivers and Dahlem2014). A lag occurs between the start of activity and reproduction because reproductive organs require metabolic reactivation following emergence from diapause (Wilde Reference Wilde1954; Denlinger Reference Denlinger2002; Ames and Turner Reference Ames and Turner2003). Although the minimum threshold for activity is around 0–10 °C for most species, the base temperature for oviposition is typically higher (Wilde Reference Wilde1954; Ames and Turner Reference Ames and Turner2003). Temperature shifts and stabilisation to an optimal range restarts these metabolic functions, allowing oviposition (Wilde Reference Wilde1954; Ames and Turner Reference Ames and Turner2003). The role of season and habitat on gravidity was observed within the present study, with gravid individuals being collected more frequently in the summer months and at the Rural location. These conditions are favourable for reproductive reactivation, as archetypal summers provide stable, high temperatures above the minimum threshold of most species, whereas areas with lower elevations such as the Rural location are typically warmer and less prone to rapid temperature fluctuations than higher-elevation sites are (Wall et al. Reference Wall, French and Morgan1992; Donovan et al. Reference Donovan, Hall, Turner and Moncrieff2006; Gruner et al. Reference Gruner, Slone and Capinera2007; Grytnes and McCain Reference Grytnes and McCain2007; Brundage et al. Reference Brundage, Bros and Honda2011). Starting capture dates revealed differences between the active and reproductive range for C. latifrons and L. sericata populations; however, no differences were observed in the C. vicina and L. illustris ranges. Wall et al. (Reference Wall, French and Morgan1992) stated that L. sericata requires a base temperature of 11 °C to oviposit, explaining why L. sericata were first observed at the start of April but gravid females were not encountered until the end of the month as warmer temperatures stabilised. In comparison, C. vicina has a lower temperature threshold for oviposition, being capable of laying eggs at temperatures as low as 10 °C, which aligns with the overlapping start dates observed for activity and reproduction (Ody et al. Reference Ody, Bulling and Barnes2017). Lucilia illustris and C. latifrons followed the trends of their respective genera, with L. illustris having a later reproductive range in the annual period than C. latifrons had. No data on ovipositional temperature thresholds for L. illustris and C. latifrons were found to support or refute these findings, highlighting a novel area of research for exploration.
Hymenoptera can exert high predation on blow flies, decreasing their overall catch yield (Catts and Goff Reference Catts and Goff1992; Frederickx et al. Reference Frederickx, Dekeirsschieter, Verheggen and Haubruge2013). The frequency of Hymenoptera observed in this study exceeded the normal frequency of collection in previous studies (Dillon Reference Dillon1997; Carvalho et al. Reference Carvalho, Thyssen, Goff and Linhares2004; Wang et al. Reference Wang, Ma, Jiang, Wang, Li and Yin2016). These predatory Hymenoptera (hornets and yellowjackets) entered the traps and were observed actively killing and feeding on adult blow flies and on eggs, causing a reduction in the abundance and diversity of recorded species, as Archer and Elgar (Reference Archer and Elgar2003) saw in Australia and Moretti et al. (Reference Moretti, Giannotti, Thyssen, Solis and Godoy2011) and Somavilla et al. (Reference Somavilla, Souza, Da Silva and Keppler2019) saw in Brazil.
Trap escape rates
Small baited traps are one of the main methods used to analyse Calliphoridae colonisation in field settings (Anderson Reference Anderson2000; Hwang and Turner Reference Hwang and Turner2005; Brundage et al. Reference Brundage, Bros and Honda2011; Farinha et al. Reference Farinha, Dourado, Centeio, Oliveira, Dias and Rebelo2014; Weidner et al. Reference Weidner, Gemmellaro, Tomberlin and Hamilton2017). These traps provide a contained, cost-effective, and easy-to-manage system (Farinha et al. Reference Farinha, Dourado, Centeio, Oliveira, Dias and Rebelo2014; Weidner et al. Reference Weidner, Gemmellaro, Tomberlin and Hamilton2017). Studies testing efficacy have shown that small baited traps are useful predictors of necrophagous insect composition, making them a viable resource for the field study of Calliphoridae (Farinha et al. Reference Farinha, Dourado, Centeio, Oliveira, Dias and Rebelo2014; Weidner et al. Reference Weidner, Jennings, Tomberlin and Hamilton2015, Reference Weidner, Gemmellaro, Tomberlin and Hamilton2017). However, there may be differences in collections from bait traps versus carrion (LeBlanc et al. Reference LeBlanc, Boudreau and Moreau2021). In addition, it is possible that flies may be attracted to a trap, oviposit on the bait, and yet escape capture. Escapes were prevalent in the present study: species reared versus those trapped as adults showed female escape rates of more than one-third for all species, with some species as high as two-thirds. In addition, this method of determining escape rate was specific to the blow flies that laid eggs in the traps: it did not determine the rate of escape of male blow flies, how many female blow flies of an identified species escaped, or how many escaped without laying eggs, nor did it provide information on the escape of blow flies in the traps earlier than 1 July 2019. Therefore, the rate of escape may have been higher than calculated with the available data. The consumption of female blow flies by high numbers of hymenopteran predators in the traps may have increased the calculated rate of escape based on their perceived absence in a trap upon collection. Overall, these results demonstrated that female blow flies were capable of escaping from the bottle traps following oviposition, indicating that better trap designs are needed to accurately measure the abundance of necrophagous insects within an environment and perhaps also explaining why some studies have collected different species directly from carrion.
Conclusion
This study documented the distribution of Calliphoridae in the Lower Mainland of British Columbia, highlighting shifts in species abundance and diversity relative to habitat type and seasonal changes. In addition, the female-biased sex ratio of Calliphoridae and the reproductive ranges of gravid females were shown to be important in colonisation. This and similar studies on the necrophagous community are continually needed to provide baseline databases because changing climatic conditions cause species to migrate to previously uninhabited areas and can result in location-specific adaptation, which may alter temperature thresholds. In addition, our understanding of calliphorid reproductive and active ranges is limited, requiring further research to assess what implications these range differences could have in our conceptualisation of colonisation by blow flies. These data form a critical part of forensic entomology analysis in criminal investigations and are essential to the accurate assessment of evidence within differing locales.
Acknowledgements
The authors acknowledge Fionn Lai, Cassandra Chowdhury, Payten Smith, and Lucas Angelozzi for their help in conducting this experiment. They also thank Dr. Terry Whitworth for examining and confirming specimen identifications and are also extremely grateful to the reviewers for their comments.
Funding
The Natural Sciences and Engineering Research Council of Canada helped to fund this project through their contribution of the Alexander Graham Bell Scholarship to C.A.R.S., provided through the Canada Graduate Scholarships–Master’s Program (CGS–M) for the 2019–2020 period. Simon Fraser University helped to fund this project through a Graduate Fellowship to C.A.R.S.
Competing interests
The authors declare they have no competing interests.