Low Se intake has been associated with increased risk of cancer and may influence the risk of CVDReference Rayman1; however, the mechanisms are not well understood. Numerous hypotheses have been proposed to explain potential beneficial effects of increased Se intake; among these, increased expression of selenoproteins, induction of carcinogen metabolism and improved blood lipid profileReference Ip2–Reference Wadkar-Navsariwala, Prins, Swanson, Birch, Ray, Hedayat, Lantvit and Diamond5. Se compounds have been shown to affect different cellular processes and molecular pathways. Incorporated as selenocysteine, Se is essential for selenoprotein synthesis and function. More than twenty-five selenoproteins have been identified, among these the glutathione peroxidases (GPX), thioredoxin reductases (TrR) and selenoprotein P (SelP) which play a role in antioxidant defenceReference Saito, Sato, Hirashima, Takebe, Nagasawa and Takahashi6. By reducing oxidative damage to DNA, lipids and proteins, increased expression of selenoproteins may result in decreased oxidative stress, which has been linked to risk of cancer and CVD. Increased risk of breast and lung cancer has been reported in carriers of the variant allele of a GPX1 polymorphismReference Ratnasinghe, Tangrea, Andersen, Barrett, Virtamo, Taylor and Albanes7, Reference Hu and Diamond8 that is associated with lowered GPX activityReference Ravn-Haren, Olsen, Tjonneland, Dragsted, Nexo, Wallin, Overvad, Raaschou- Nielsen and Vogel9.
Bioavailability and effect on selenoprotein expression depends on the form in which the Se compound is present. Inorganic Se such as selenite and selenate is less effective in raising blood Se levels compared with organic Se sources such as selenomethionineReference Thomson, Burton and Robinson10–Reference Thomson, Robinson, Butler and Whanger13, but has been found equally effective in raising whole-blood GPX activity in long-term supplementation studies. Compared with erythrocyte and plasma GPX activities, thrombocyte GPX responds to Se supplementation in populations with higher Se status. While erythrocyte and plasma GPX activities plateau at Se intakes corresponding to plasma Se concentrations of about 70–90 μg/l and higher, plasma Se levels of about 140–200 μg/l are needed to obtain saturation of thrombocyte GPXReference Levander, Alfthan, Arvilommi, Gref, Huttunen, Kataja, Koivistoinen and Pikkarainen12–Reference Neve15. The response in thrombocyte GPX is dependent on Se source and it has been suggested that the slow response of thrombocyte GPX to organic Se sources is a result of selenomethionine being incorporated non-specifically into the protein pools before being converted into the precursor available for GPX synthesisReference Neve, Vertongen and Capel16, Reference Butler, Thomson, Whanger and Robinson17, whereas inorganic Se sources directly enter into the Se pool available for synthesis of selenoproteins. Thrombocyte GPX activity has been reported to correlate with liver Se and liver GPX activityReference Thomson, Steven, van Rij, Wade and Robinson18, and may be a more suitable measure to study the biological effects of Se supplementation over a wider range of Se intakes.
Bioavailability of Se from different food sources varies. Se from bread and meat were equally efficient in raising plasma Se level and resulted in a similar thrombocyte GPX activityReference van der Torre, Van, Schaafsma, Wedel and Ockhuizen19. In another study, Se-enriched wheat increased plasma and erythrocyte Se levels comparable with Se-enriched yeast but resulted in a higher thrombocyte GPX activity that was similar to selenateReference Levander, Alfthan, Arvilommi, Gref, Huttunen, Kataja, Koivistoinen and Pikkarainen12. Se from fish was found to be a good source of dietary Se, resulting in a better retention of Se compared with Se-enriched yeast and selenateReference Fox, Van den Heuvel and Atherton20. Dairy products are one of the main Se sources in the Danish diet, accounting for 18 % of dietary Se intakeReference Lyhne, Christensen and Groth21 and Se-enriched milk might provide a realistic means of improving dietary Se status in populations with low dietary Se intakes, especially since the bioavailability of milk Se is at least 66 %Reference Chen, Lindmark-Mansson, Drevelius, Tidehag, Hallmans, Hertervig, Nilsson and Akesson22.
The cancer-protective effects of Se have, based on numerous rodent cancer models, been linked to increased expression of protective enzymes. These include the selenoproteins GPX, thioredoxin reductase, SelP as well as phase 2 detoxification enzymes, such as NAD(P)H:quinone oxidoreductase (NQO1) and glutathione S-transferases (GST)Reference El Bayoumy and Sinha23. Reduced glutathione (GSH) is a cofactor for GPX and necessary for GSH conjugation by GST. The catalytic subunit of γ-glutamylcysteine ligase (GCLC) is involved in the de novo synthesis of GSHReference Gipp, Chang and Mulcahy24, Reference Gipp, Bailey and Mulcahy25. Common for GCLC and genes encoding phase 2 detoxification enzymes is the presence of electrophile response elements in the promoter region (TGACNNNGC), which mediate the transcriptional up regulation in response to a variety of inducing agentsReference Chen and Kong26. Fra1, a member of the Fos family of AP-1 transcription factors, has been shown to down regulate NQO1 and GCLC gene expressionReference Venugopal and Jaiswal27, Reference Jardine, MacNee, Donaldson and Rahman28. Se could modulate these gene responses through electrophile response elements. The induction of protective enzymes by Se is involved in keeping cellular components in their appropriate redox state, which could be important in reducing risk of cancer and CVD.
Se supplementation (200 μg/d for 3 months) has previously been reported to decrease plasma and LDL oxidation, decrease levels of erythrocyte oxidised GSH, increase reduced GSH and increase glutathione reductase (GR) and GPX activity in subjects under oxidative stressReference Hussein, Rosenblat, Refael and Aviram3. These results suggest that dietary Se supplementation can activate the GSH system and decrease lipid oxidation, thereby possibly reducing the risk of CVD. However, not all studies support a beneficial effect of increased Se intake on blood lipids. In the SU.VI.MAX studyReference Hercberg, Bertrais and Czernichow29 where subjects were supplemented with low doses of antioxidants, including Se, for 7.5 years, no improved lipid profile was observed. On the contrary, results suggested prevalence of hypertriacylglycerolaemia in supplemented men and hyperlipidaemia in supplemented womenReference Hercberg, Bertrais and Czernichow29. Results from studies investigating the relationship between Se status and CVD are inconclusive and further studies are needed to clarify whether effects on lipoproteins are involved in the possible protective effect of Se.
The present study was initiated to examine the acute effects on oxidative defence and risk factors for CVD of supplementation with high doses of different forms of Se. Three different Se sources were used and compared with placebo: selenate, Se-enriched yeast and Se-enriched milk. Se status was assessed by serum Se levels and acute effects on markers of oxidative defence. Risk factors for CVD were determined by measuring activities of GPX, GR and GST in plasma, erythrocytes or thrombocytes, expression of GPX1, TrR1, SelP, GCLC, NQO1 and the transcription factor Fra1 in leucocytes, as well as plasma lipid resistance to oxidation, plasma TAG, C-reactive protein (CRP; a marker of inflammatory response), and total, LDL- and HDL-cholesterol.
Materials and methods
Subjects
Twenty healthy men aged 18–40 years were recruited by advertisement at local universities in the Copenhagen area. Exclusion criteria were smoking, obesity (BMI>30 kg/m2), family history of chronic diseases, use of any medication, heavy physical exercise (>10 h/week), high intake of fish (more than two times per week) and use of vitamin or mineral supplements 2 weeks and Se supplements 8 weeks before entry into the study. The subjects received oral and written information concerning the study before they gave their written consent. All twenty subjects completed the study. The research protocol was approved by the Scientific Ethics Committee of the municipalities of Copenhagen and Frederiksberg (01–135/04).
Study design
The study was performed as a randomised, double-blind, 4 × 1 week cross-over study. Subjects supplemented their usual diet with one of four test meals 8 weeks apart. Test meals were coded with different colours and given in random order. The code was not broken until all analyses were completed and the results were analysed statistically. On the first day of each intervention period, the subjects were served a standardised breakfast (which contained the test meal) and a standardised lunch at the Department of Human Nutrition at the University of Copenhagen, Frederiksberg, Denmark. Subjects were not allowed to consume other foods during the stay except for water. They were asked to finish breakfast within 30 min. On the second day of each intervention period they were served the test meal with breakfast immediately after blood sampling (t = 24 h) and were supplied with 5 litres frozen milk and five tablets for the last days of the intervention week. They were asked to keep daily records of test-meal consumption and wellbeing during the study. The four test meals consisted of 1 litre of milk (Se-enriched or control milk) and a tablet (Se-enriched yeast, selenate or placebo). Se-enriched milk (480 μg Se/d) was given with a placebo tablet, and control milk with Se-enriched yeast (300 μg Se/d), selenate (300 μg Se/d) or placebo tablets.
Validation of food-frequency questionnaire
An FFQ was used to estimate the dietary Se intake of the study participants before inclusion. The FFQ was validated against a 7 d diet record in a pilot study. Twelve subjects were asked to fill in the FFQ and to register all dietary intakes for seven consecutive days. The FFQ was filled in before the diet record.
The FFQ was divided into multiple categories with seven main groups of daily groceries, from where Danes usually cover their dietary Se intake. The main groups are: meat, fish for dinner, accompaniments for dinner, bread meals, food taken with bread, dairy products and grains, cereals, and vitamin–mineral supplements. There were sub-questions in every category, such as ‘how often’ a specific type of food and ‘how large’ a portion was consumed per meal. The frequency questions included: times per week, times per month, and rarely or never. Portion-size quantification was asked by use of a folio. When it was not possible to determine the weight with these methods, it was asked how much of a pack of meat was consumed, or how much of a whole chicken or chicken breast was consumed. The dose (μg Se/d) was determined after the interview from a result diagram. The concentration of Se per 100 g for specific food types was inserted beforehand in the diagram.
Dietary Se intakes based on the 7 d food records were calculated using the Dankost program version 3000 (Dansk Catering Center A/S, Herlev, Denmark; http://www.dankost.dk).
Subjects were issued with diet record books and calibrated electronic scales. Where a meal was not displayed with precise ingredients, a similar standard meal was used, which was encoded in the Dankost program. Where the amount was not displayed, for example one piece of bread, a ‘typical weight of food’ was usedReference Andersen, Jensen and Haraldsdottir30. Where a specific food type was not encoded in the Dankost program, a table from the food database (http://www.foodcomp.dk) from the National Food Institute (Mørkhøj, Søborg, Denmark) was used.
Selenium-enriched milk
Se-enriched milk was produced at the Faculty of Agricultural Sciences, Foulum, Denmark. Eight dairy cows were supplemented with about 100 mg Se in the form of Se yeast (SelPlex; Alltech Inc., Nicholasville, KY, USA) per d for 3 d, and milk from the fourth day of supplementation was pasteurised, adjusted to 1·5 % fat, and bottled at a local dairy plant (Osteriet Hinge, Kjellerup, Denmark). Control milk was produced in parallel from eight dairy cows that received no extra Se supplementation. Initial Se analyses of the milk showed that the Se-enriched milk contained about 350 μg Se/l compared with control levels of about 12 μg/l. On the basis of these results, subjects were daily supplemented with Se contained in 1 litre of milk. Milk was frozen at − 20°C until used. Control analyses of the milk performed at two independent laboratories after the intervention study was terminated suggested that the Se concentration of both Se-enriched and control milk was higher than first anticipated. These results showed a mean Se concentration of about 480 and about 25 μg/l for the Se-enriched milk and control milk, respectivelyReference Sloth, Larsen, Bugel and Moesgaard31.
Blood sampling and sample handling
Fasting blood samples were drawn in the morning of day 1 (t = 0), 2 (t = 24 h), 8 (t = 1 week) and after 4 weeks (t = 4 weeks). On day 1, postprandial blood samples were taken 3 h (t = 3 h) and 6 h (t = 6 h) after the test meal was served. All blood samples were taken after 10 min supine resting. Breakfast was served after the first blood sample was taken (t = 0) and lunch immediately after the 3 h blood sample.
Blood samples drawn in plain tubes (Becton Dickinson, Franklin Lakes, NJ, USA) were centrifuged (3000 g; 15 min at room temperature) and serum isolated for analysis of Se status. Blood samples were collected in 10 ml EDTA-coated tubes (Becton Dickinson) for isolation of plasma, thrombocytes and erythrocytes for measurement of lipid oxidation, plasma lipids and enzyme activities, and buffy coat for determination of gene expression. Samples were centrifuged for 15 min (150 g at room temperature) and thrombocytes isolated from the thrombocyte-rich plasma (1500 g; 5 min at room temperature). The thrombocyte pellet was washed twice in 0·9 % NaCl and re-suspended in 0·5 ml 0·9 % NaCl. Erythrocytes and buffy coat were isolated by centrifugation (1500 g; 10 min at 4°C). The pearly white buffy-coat layer containing leucocytes was carefully removed from the top for RNA purification and remaining erythrocytes were lysed by adding an equal amount of MilliQ water to the tube. Total RNA was extracted using a QIAamp RNA blood mini kit (QIAgen, Ballerup, Denmark). Serum, plasma, thrombocytes, erythrocytes and RNA were stored at − 80°C until analysis.
Serum selenium status
Se in serum was measured by inductively coupled plasma (ICP)–MS using the standard mode as described by PruszkowskiReference Pruszkowski32. Serum was diluted twenty times before aspiration into the ICP–MS. Seronorm (Nycomed Pharma Diagnostics, Oslo, Norway) was used as reference material. The assigned value was 83 μg Se/l and values between 79 and 86 μg Se/l were obtained. Se was determined as 82Se with a CV < 5 %. All serum samples from one individual were analysed in the same run to minimise the influence of inter-assay variation.
Plasma lipid oxidation
Plasma lipid resistance to oxidation was determined according to Mayer et al. Reference Mayer, Schumacher, Brandstatter, Wagner and Hermetter33. The time-dependent decrease in fluorescence intensity was followed using a Wallac 1420 multilabel counter from Perkin Elmer (Life Sciences, Allerød, Denmark). The fluorescent marker (1-palmitoyl-2-((2-(4-(6-phenyl-trans-1,3,5-hexatrienyl)-phenyl)ethyl)-carbonyl)-sn-glycero -3-phosphocholine) was purchased from Chemica Technologies (Bend, OR, USA). The inter-day variation in lag time for a control plasma sample was 4 %.
Blood lipids and plasma C-reactive protein
Plasma cholesterol, TAG, and HDL- and LDL-cholesterol levels were determined using commercially available kits from Roche Diagnostics, Basel, Switzerland (catalogue no. 12016630 122, 12016648 122, 03030024 122 and 03038777 122, respectively). Analyses were performed on a Cobas Mira-plus (Roche Diagnostics), with inter-day variations of < 3 %.
Plasma CRP levels were determined on an Immulite 1000 analyser (Diagnostic Products Corporation, Los Angeles, CA, USA) using a chemiluminescent enzyme immunometric assay (catalogue no. LKCR-10; Diagnostic Products Corporation), with a CV < 7 %.
Defence enzyme activities in erythrocytes, plasma and thrombocytes
GR and GPX activities were determined spectrophotometrically on a Cobas Mira analyser (Roche Diagnostics, Basel, Switzerland) in erythrocyte lysates, plasma and thrombocyte lysates according to Wheeler et al. Reference Wheeler, Salzman, Elsayed, Omaye and Korte34, using t-butyl-hydroperoxide as substrate for GPX. GST activity was measured in erythrocyte and thrombocyte lysates as previously described using 1-Cl-2,4-dinitrobenzene as substrateReference Dragsted, Pedersen and Hermetter35. Activities measured in erythrocytes were related to the amount of Hb in the sample, whereas activities determined in plasma and thrombocytes were related to protein concentrations. Hb and protein contents were determined using commercially available kits (catalogue no. HG 980; Randox, Ardmore, UK, and catalogue no. A11A01669; ABX Diagnostics, Montpellier, France, respectively). Control samples (erythrocytes, plasma and thrombocytes) were included for every tenth sample analysed. Inter- and intra-day variations for the control samples were 11·9 and 6·9 %, and 6·4 and 3·8 % for plasma GPX and GST respectively, 8·9 and 2·8 %, and 5·6 and 3·3 %, and 17 and 4 % for erythrocyte GPX, GR and GST respectively, and 17 and 8 %, and 19 and 4 % and 19 and 7·6 % for thrombocyte GPX, GR and GST, respectively.
Leucocyte mRNA quantification of GPX1, TrR1, SelP, GCLC, NQO1 and Fra1
Total leucocyte RNA was quantified spectrophotometrically by measuring optical density (OD)260 and the purity was tested by measuring OD260:280 ratios using the NanoDrop® ND-1000 UV-Vis spectrophotometer. First-strand cDNA was synthesised from 500 ng of total RNA in a total volume of 10 μl with Random Hexamer primer and Superscript™ II RNase H-RT according to the manufacturers (Invitrogen, Taastrup, Denmark).
Primers and TaqMan® probes were designed using the primer analysis software Primer Express v. 1.5 (Applied Biosystems, Stockholm, Sweden), based on sequences from the Genbank database (Table 1). Whenever possible, the forward and reverse primers were placed in exons separated by an intron. A primer and probe set for the eukaryotic 18 s rRNA endogenous control was obtained from Applied Biosystems (Stockholm, Sweden).
Table 1 Primers and probes used for real-time polymerase chain reaction analysis

GPX1, glutathione peroxidase 1; TrR1, thioredoxin reductase 1; SelP, selenoprotein P; GCLC, γ-glutamylcysteine ligase catalytic subunit; NQO1, NAD(P)H:quinone oxidoreductase 1; Fra1, FOS-like antigen 1; 6-FAM, 6-carboxyfluorescein; MGB, minor groove binding.
TaqMan PCR assays for each of the six gene targets were performed in triplicate on cDNA samples in ninety-six-well optical plates on a 7900HT FAST Real-Time PCR System (Applied Biosystems). The PCR reactions were performed in a total volume of 10 μl containing 2 ng cDNA according to protocols from Applied Biosystems. Primer and probe concentrations were 900 and 300 nm, respectively. The amplification conditions were: 95°C for 20 s, forty-five cycles of 95°C for 1 s and 60°C for 20 s.
The results were calculated using the comparative Ct method with 18 s rRNA as endogenous control as described previously (Applied Biosystems, 2001, User Bulletin no. 2, ABI PRISM 7700 Sequence Detection System; Applied Biosystems, Foster City, CA, USA).
PCR efficiency was tested for each target gene normalised to 18 s rRNA. A value of the slope less than 0·1 was obtained for all genes.
Inter-plate variation for a control sample was 3·4, 2·8, 0·7, 2·3, 2·4 and 2·8 % for GPX1, TrR1, SelP, GCLC, NQO1 and Fra1, respectively.
Statistical analysis
All data are presented as mean values and standard deviations. Statistical analyses were performed using SAS (SAS Institute, Cary, NC, USA). Treatment effects were analysed using PROC MIXED in the SAS System, with treatment (placebo, selenate, Se-enriched yeast or Se-enriched milk), order (the order in which the four test meals were provided) and period (four periods) as fixed factors, subject as a random factor and baseline measurements and weight as covariate. If period effects were statistically significant, period was excluded from the model statement and included in the repeated statement together with subject (subject × period). Data that could not meet the criteria of variance homogeneity and normal distribution (determined by residual plot examination) were log transformed. For all tests a P value less than 0·05 was considered statistically significant. Spearman or Pearson correlations were used to identify correlating variables.
Results
Validation of food-frequency questionnaire
Validation of the FFQ used showed that it was comparable with the use of a 7 d food record (r 0·815; P = 0·001). However, in the present study we found no correlation between serum Se levels and dietary Se intake estimated using the FFQ (P = 0·213).
Baseline characteristics of study participants
Baseline characteristics for the study participants were as follows: age 26·8 (sd 5·04) years; weight 78·9 (sd 9·22) kg; BMI 23·4 (sd 1·97) kg/m2 and estimated dietary Se intake 49·8 (sd 13·6) μg/d. No extreme values for habitual dietary Se intake were reported.
Compliance
Based on the participant's record on milk and tablet intake, only one participant failed to finish 1 litre of milk because the milk was sour. When the code was broken after the end of the analytical work, this milk turned out to be control milk, and the data were not excluded from the statistical analyses.
Serum selenium levels
No increase in serum Se levels was observed with any Se source within the first 24 h (Table 2), but after 1 week of Se supplementation, significant increases in serum Se levels were observed for all three Se sources. The organic Se sources, Se-enriched yeast and Se-enriched milk, increased serum Se levels most after 1 week (P < 0·0001 for both compared with placebo) and more than selenate (P = 0·0008 and P < 0·0001, respectively, compared with selenate), while selenate supplementation resulted in smaller yet still statistically significant increase in serum Se level (P = 0·0109, compared with placebo). Serum Se levels after Se-enriched yeast or Se-enriched milk supplementation were not significantly different (P = 0·2093), but were still significantly higher than controls after 3 weeks washout, whereas levels after selenate supplementation quickly returned to baseline.
Table 2 Effect of selenium supplementation on serum selenium levels and on activities of glutathione reductase (GR), glutathione peroxidase (GPX) and glutathione S-transferase (GST) measured in plasma, erythrocytes or thrombocytes†
(Mean values and standard deviations)
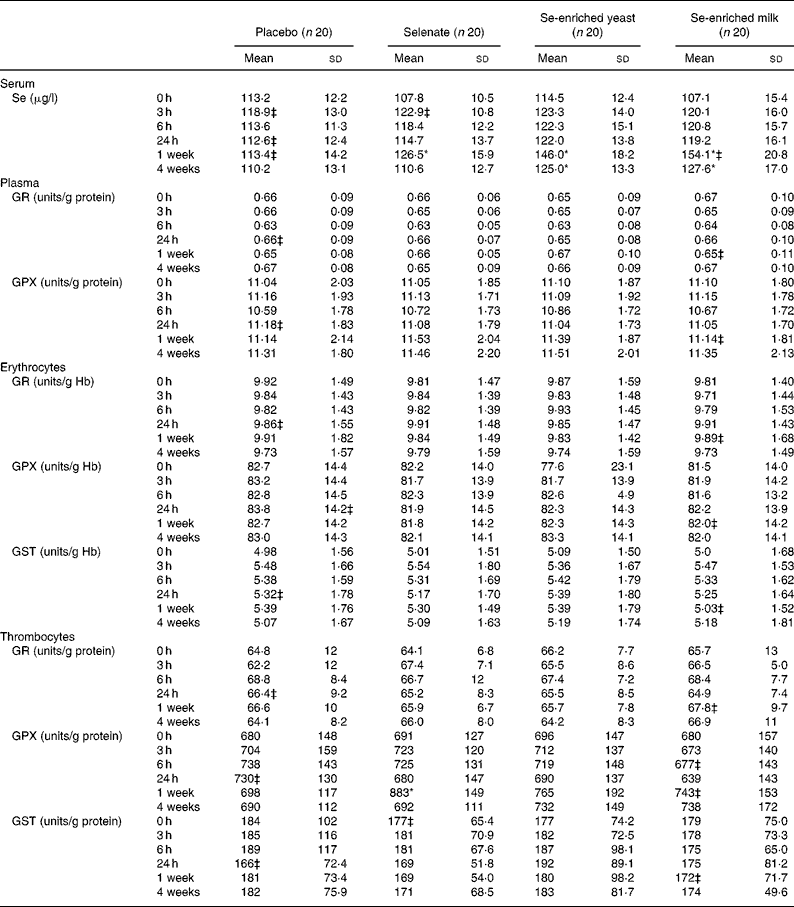
* There was a significant treatment effect (P < 0·05; PROC MIXED).
† Activities measured in plasma or thrombocytes are related to the amount of protein in the samples, while activities determined in erythrocytes are related to the amount of Hb.
‡ n 19.
Enzyme activities
There was a statistically significant treatment effect on thrombocyte GPX activity (P = 0·0152). Thrombocyte GPX activity was significantly increased after 1 week (P = 0·0052), but depended on Se source. No increase in thrombocyte GPX activity was found when supplementing with either Se-enriched yeast or Se-enriched milk (P = 0·2258 and P = 0·4394, respectively, compared with placebo). Selenate was the only Se source resulting in increased thrombocyte GPX (P = 0·0007 compared with placebo). None of the forms of Se supplementation affected activities of plasma GR or GPX, erythrocyte GR, GPX or GST, or GR or GST measured in thrombocytes (Table 2) (P>0·05, for all). Serum Se concentrations correlated weakly but positively with plasma and thrombocyte GPX at baseline (r 0·176 and r 0·451, respectively; P < 0·0001 for both), while weak correlation in the opposite direction was observed with erythrocyte GPX activity (r − 0·134; P = 0·008).
Gene expression
We found no effect of any form of Se on expression of the selected selenoproteins (GPX1, SelP and TrR1; P>0·05, for all) or genes involved in carcinogen metabolism (GCLC, NQO1 and Fra1; P>0·05, for all) in leucocytes (Table 3). There was a statistically significant effect of time on all expression markers except Fra1, an effect that was independent of Se.
Table 3 Effect of selenium supplementation on leucocyte gene expression of selenoproteins, and genes involved in phase 2 detoxification*
(Mean values and standard deviations)
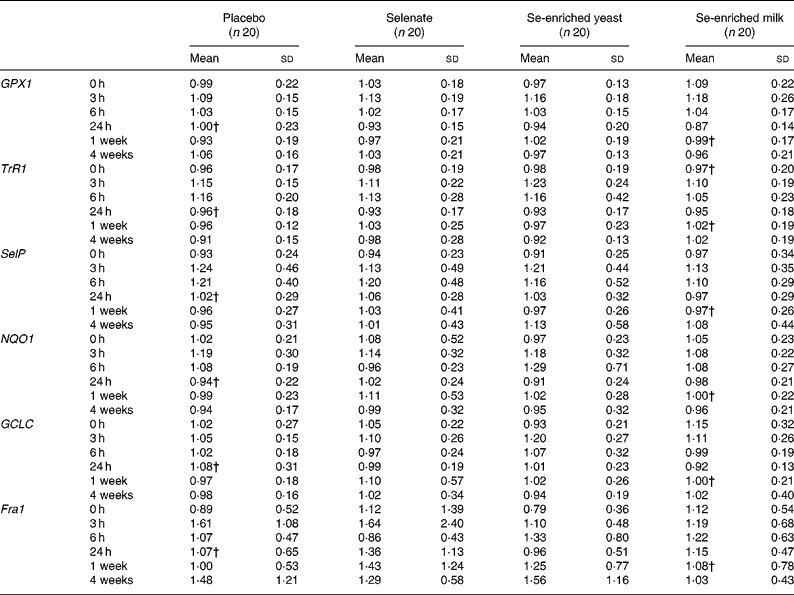
GPX1, glutathione peroxidase 1; TrR1, thioredoxin reductase 1; SelP, selenoprotein P; NQO1, NAD(P)H:quinone oxidoreductase 1; GCLC, γ-glutamylcysteine ligase catalytic subunit; Fra1, FOS-like antigen 1.
* Gene expression of target genes is related to expression of the endogenous 18s rRNA.
† n 19.
Plasma lipid resistance to oxidation and plasma lipids and cholesterol
We found no treatment effect on plasma lipid resistance to oxidation, plasma TAG, CRP, and total, LDL- and HDL-cholesterol (P>0·05, for all) (Table 4). In spite of the lack of effect of Se supplementation, we found weak yet statistically significant positive correlations between serum Se and plasma TAG, and total- and LDL-cholesterol (r 0·359, r 0·392 and r 0·330, respectively; P < 0·0001, for all) at baseline. The correlation between serum Se levels and HDL-cholesterol was much weaker (r 0·107; P = 0·032). After 1 week's supplementation, only total and LDL-cholesterol were still positively correlated with serum Se levels (r 0·277, P = 0·014 and r 0·246, P = 0·03, respectively).
Table 4 Effect of selenium supplementation on plasma C-reactive protein (CRP), lipid resistance to oxidation (LOX) and lipid profile
(Mean values and standard deviations)

* n 19.
Discussion
A low Se intake has been associated with increased risk of oxidative stress-related chronic diseases, such as cancer and CVDReference Rayman1. The potential beneficial effects of increased Se intake may be linked, through selenoproteins, to antioxidant effects, to induced carcinogen metabolism and to alterations in lipid profile. Dietary Se intake estimated with the FFQ has previously been reported to correlate with plasma Se concentrationsReference Pedersen36, Reference Jørgensen37, but we were not able to show this in the present study. However, daily dietary Se intake calculated using the FFQ reflected the intake of Danish adults, according to the latest surveyReference Lyhne, Christensen and Groth21. In the present study, supplementing healthy young men with 300 μg Se/d as selenate, Se-enriched yeast or 480 μg Se/d as Se-enriched milk for 1 week significantly increased serum Se concentrations, with Se-enriched milk = Se-enriched yeast >selenate. The organic Se sources resulted in the highest responses, as reported previouslyReference Levander, Alfthan, Arvilommi, Gref, Huttunen, Kataja, Koivistoinen and Pikkarainen12–Reference Alfthan, Aro, Arvilommi and Huttunen14, Reference Burk, Norsworthy, Hill, Motley and Byrne38. However, in spite of a nearly 50 % higher Se dose with Se-enriched milk supplementation compared with Se-enriched yeast, the former did not result in significantly higher serum Se concentrations, suggesting that Se in the forms found in milk is less absorbable than Se-enriched yeast. Alfthan et al. Reference Alfthan, Aro, Arvilommi and Huttunen14 found that supplementation with 200 μg selenate/d for 16 weeks in subjects with baseline plasma Se concentrations of about 106 μg/l did not affect plasma Se concentrationsReference Alfthan, Aro, Arvilommi and Huttunen14. We found that selenate supplementation (300 μg/d for 1 week) increased serum Se concentrations significantly in subjects with baseline serum Se concentrations comparable with the baseline concentrations in the study by Alfthan et al. Reference Alfthan, Aro, Arvilommi and Huttunen14, i.e. about 111 μg Se/l. Increases in plasma Se following selenate supplementation have been reported in subjects supplemented with 200 μg Se/d who had baseline plasma Se concentrations less than 77 μg/lReference Levander, Alfthan, Arvilommi, Gref, Huttunen, Kataja, Koivistoinen and Pikkarainen12.
Se absorption from different food varies. Fox et al. Reference Fox, Atherton, Dainty, Lewis, Langford, Baxter, Crews and Fairweather-Tait39 found that Se from wheat and garlic was better absorbed compared with Se from fish. Absorption of Se from pork and shrimps has been reported to be >80 %, and like Se from mushrooms, these food sources of Se had low bioavailability assessed as changes in blood Se levels and GPX activitiesReference Mutanen40–Reference Bugel, Sandstrom and Skibsted42. The present study suggests that Se status in subjects supplemented with Se-enriched milk is comparable with the Se status obtained with Se-enriched yeast supplementation, in spite of the Se dose being nearly 50 % higher. Whether this is a result of increased excretion, storage of Se in tissues other than blood or due to the composition of the Se compounds present in the milk needs further investigation.
No effect of Se supplementation was observed on plasma and erythrocyte GPX activities. Effects on erythrocyte GPX were not expected in such a short intervention study, considering the 120 d life span of the blood cells, and the lack of effect on plasma GPX activity is in agreement with earlier studies reporting saturation of plasma GPX activity in subjects with plasma Se concentrations above 70 μg/lReference Levander, Alfthan, Arvilommi, Gref, Huttunen, Kataja, Koivistoinen and Pikkarainen12, Reference Alfthan, Aro, Arvilommi and Huttunen14, Reference Tarp, Hansen, Overvad, Thorling, Tarp and Graudal43. Thrombocyte GPX activity plateaus at higher plasma Se concentrationsReference Alfthan, Xu, Tan, Aro, Wu, Yang, Liang, Xue and Kong44 and is therefore a more sensitive marker for short-term Se intake in populations such as the Danes with dietary intakes high enough to obtain saturation of GPX activity in both plasma and erythrocytes. Plasma Se concentrations vary with the form of Se supplementation, and we have previously found in a 5-year supplementation study with Se-enriched yeast that thrombocyte GPX activity is not saturated until plasma Se concentrations reach 165–220 μg/l (G Ravn-Haren, BN Krath, K Overvad, S Cold, S Moesgaard, EH Larsen & LO Dragsted, unpublished results). Since the thrombocyte has a short circulation time of 7–10 d, relatively rapid changes in inducible enzymes might take place. Only selenate supplementation increased thrombocyte GPX activity. The lack of effect after supplementation with organic Se sources in the present study could be due to the short length of supplementation, and the need to catabolise Se from selenomethionine in body proteins before it can be converted into the precursor available for selenoprotein synthesis. Since GPX protects thrombocytes against lipid hydroperoxides, increased thrombocyte GPX activity could modulate thrombocyte function by limiting the stage of thrombocyte activation (a marker of cardiovascular risk).
Recently, Burk et al. Reference Burk, Norsworthy, Hill, Motley and Byrne38 reported no correlation between plasma Se concentrations and plasma GPX activity or SelP levels in subjects with a baseline plasma Se concentration of about 122 μg/l. They found that selenite supplementation at doses up to 380 μg/d for 16 weeks did not increase plasma Se concentrations. In the young Danish men in the present study, who had serum Se concentrations of about 111 μg/l, we found weak but significant correlations at baseline between serum Se concentrations and GPX activity in all three blood compartments. We also found significant increases after just 1 week's supplementation with a lower dose of selenate (300 μg/d). Se absorption from selenate has been reported to be higher compared with selenite, but retention of the two inorganic Se sources is comparableReference Van Dael, Davidsson, Munoz-Box, Fay and Barclay45. Since plasma GPX activity did not increase in the present study and selenate directly enters the Se pool, the increase in serum Se after selenate supplementation suggests that the concentration of another plasma selenoprotein has increased. SelP might be a possible candidate; however, we did not observe an increase in SelP gene expression measured in leucocytes. It has been reported that SelP concentrations plateau at higher plasma Se concentrations than plasma GPX activityReference Xia, Hill, Byrne, Xu and Burk46, so whether this increase in serum Se is caused by increased SelP concentrations or simple non-specific incorporation into albumin or other small plasma proteins needs further investigation.
Se supplementation has previously been observed to increase cellular synthesis of both selenoproteins and other proteins, especially detoxification enzymesReference El Bayoumy and Sinha23. Acute induction of gene expression and increased activities of detoxification enzymes were found in mouse liver following treatment with high doses of different Se compoundsReference El-Sayed, boul-Fadl, Lamb, Roberts and Franklin47. No common pattern of effects was observed with similar Se compounds and there was rarely concordance between effects on mRNA and enzyme activity. We found no effect of the different Se compounds on gene expression in human leucocytes and activity of phase 2 enzymes. This does not exclude a possible effect on carcinogen metabolism in liver, but raises questions on the validity of the use of blood samples as a surrogate marker of Se-induced gene expression in other target organs.
A high level of serum Se has been associated in a prospective study with reduced oxidative stress to lipids determined as excreted isoprostanesReference Helmersson, Arnlov, Vessby, Larsson, Alfthan and Basu48. Here, we have investigated the short-term effects of high doses of Se on a marker of plasma lipid resistance to oxidation but we did not find a similar effect.
Elevated levels of CRP, and total and LDL-cholesterol, decreased levels of HDL-cholesterol and oxidised LDL are risk factors for CVD. Studies of Se and CVD risk are not conclusive. In former skin-cancer patients, supplementation with 200 μg Se/d as Se-enriched yeast significantly decreased the risk of cancer, while no effect was seen on the prevention of CVD during 7.6 years of follow-upReference Stranges, Marshall, Trevisan, Natarajan, Donahue, Combs, Farinaro, Clark and Reid49. In the SU.VI.MAXReference Hercberg, Bertrais and Czernichow29 study where subjects were supplemented with nutritional doses of antioxidants, including 100 μg Se/d, with a median follow-up time of 7.5 years, no effect was reported on total cholesterolReference Hercberg, Bertrais and Czernichow29. They concluded that long-term supplementation with antioxidants did not improve the lipid profile. On the contrary they found prevalence of hypertriacylglycerolaemia with antioxidant supplementation in men. Subjects in the present study were healthy and had normal CRP, normal total, HDL- and LDL- cholesterol and TAG levels at inclusion and we found no acute effect of Se supplementation on plasma total, HDL- and LDL-cholesterol, CRP or plasma TAG levels. Both negative and no associations between CRP and Se levels have been reported in subjects with various pathological conditions compared with healthy subjectsReference Maehira, Luyo, Miyagi, Oshiro, Yamane, Kuba and Nakazato50, Reference Mayland, Allen, Degg and Bennet51. In healthy subjects, serum Se concentrations were predicted by serum total cholesterol, CRP concentrations and dietary SeReference Ghayour-Mobarhan, Taylor, New, Lamb and Ferns52, but results from the present study do not support any short-term effect of high Se intake on risk factors for CVD. The duration of the intervention (1 week) may not be long enough to affect blood lipids. However, the weak but highly significant positive correlations between serum Se levels and blood lipids could be due to long-term effects or reflect effects of a dietary Se source that are independent of Se. The present study does not suggest any short-term beneficial effects of increased Se intake on these risk markers and further investigation is needed to conclude whether Se supplementation has any effects on blood lipids that could result in decreased risk of CVD.
Acknowledgements
We thank all the individuals who volunteered for the study and Vibeke Kegel, Ella Jessen, Berit Hoielt, Kira Larsen, Hanne L. Pedersen, Hanne Jensen, Elin Skytte and Marianne Hansen for excellent technical support. The study was financially supported by a grant from the Committee for research and development of the Öresund region. University of Copenhagen and Biomedical Nutrition at Lund University are members of the European Union NoE Environmental Cancer Risk, Nutrition and Individual Susceptibility (ECNIS; no. 513943), which partly supported the study.