2.1 Basin and Continental Framework
As briefly discussed in Section 1.8, the Gulf of Mexico (GoM) Mesozoic depositional history can be subdivided into a series of tectonostratigraphic phases (Figure 1.24). These phases reflect both the long-term tectonic evolution of the basin and its predecessors, as well as the shorter-term eustatic and climatic processes influencing sedimentation. While the Cenozoic phases have higher frequency (three phases over 66 million years), one can argue for three tectonostratigraphic phases over 170 million years or more since the suturing of Pangea and joining of Laurentia and Gondwana. The three phases that cover the post-Quachita–Marathon–Appalachian orogeny to end of Cretaceous (299 Ma to 66 Ma) are:
1. Post-Orogenic Successor Basin-fill and Rifting Phase
2. Middle Mesozoic Drift and Cooling Phase
3. Late Mesozoic Local Tectonic and Crustal Heating Phase.
We regard the first phase as a predecessor to formation of the GoM basin, but it is worthwhile to discuss this in detail as numerous tectonic and stratigraphic elements persisted into the Middle Mesozoic Drift and Cooling Phase, some extending into the Late Mesozoic Local Tectonic and Crustal Heating Phase. Galloway (Reference Galloway2009) has argued that the basin initiated with deposition of the Louann Salt, the first stratigraphic unit that spans much of the area that is today known as the GoM. As discussed below, salt deposition was probably underway at 170 Ma, at the start of the Drift and Cooling Phase. New plate tectonic models suggest that accelerated opening of the Gulf began as an intrusive phase of oceanic crust generation below the accumulating mass of evaporites and later extrusive separation of salt bodies between the northern and southern GoM (Norton et al. Reference Norton, Lawver and Snedden2018), a process also observed in other areas (Norton et al. Reference Norton, Carruthers and Hudec2015).
The end of the Late Mesozoic Local Tectonic and Cooling Phase, and the Mesozoic as a whole, was ushered in by the Chicxulub impact event at 66 Ma, which greatly altered the paleobathymetry and land surface of the GoM (Denne et al. Reference Denne, Scott and Eickhoff2013; Sanford et al. Reference Sanford, Snedden and Gulick2016). It also, to some degree, set up the basin configuration that the Cenozoic tectonostratigraphic phases modified by sediment input from the newly emerged Laramide highlands and rejuvenated Appalachian mountains (Galloway et al. Reference Galloway, Whiteaker and Ganey-Curry2011; Snedden et al. Reference Snedden, Galloway and Milliken2018a).
These three Mesozoic tectonostratigraphic phases naturally reflect the larger-scale geodynamics that controlled GoM basin opening and evolution. Plate tectonic forces drove the geodynamic systems that controlled subsidence and accommodation, uplift, and source terrane exposure, and even marine water entry to the nascent basin. Thus, our tectonostratigraphic scheme is based on current thinking regarding plate tectonics of the greater GoM basin since the breakup of Pangea, as described in detail in the following.
2.2 Plate Tectonic Reconstructions since 240 Ma
Geological views of the origin and evolution of the GoM are changing with evaluation of new deep-imaging seismic reflection and refraction data. The plate reconstructions of the GoM from 240 Ma to 140 Ma (Figure 2.1A–F) have evolved from research of the PLATES project at the University of Texas at Austin (www-udc.ig.utexas.edu/external/plates). The reconstructions are based on mapping of tectonic elements from multiple sources, including seismic, potential field, and geologic data. The main driver of GoM tectonics during this time is the motion between Yucatán and North America, driven by creation of oceanic crust. Deformation to the east in the Florida region separated the Yucatán motion from the central Atlantic, but we have no constraints on how this deformation was distributed. Another region of significant deformation was in Mexico. Since the earliest days of plate reconstructions it has been recognized that in a Pangea reconstruction the northwest part of South America has considerable overlap onto Mexico if both regions are mapped in their present-day geometries. We use a modified form of the megashear hypothesis (Anderson and Schmidt Reference Anderson and Schmidt1983) to move Mexico away from South America. We also recognize a single tectonic block in eastern Mexico that consists of a Permo-Triassic arc system that formed along the boundary between Pangea and the proto-Pacific Ocean (Norton et al. Reference Norton, Lawver, Snedden, Lowery and Snedden2016).

(A) Post-Collision, Successor Basin-Fill Phase, 240 Ma.

(B) Rift Phase, 200 Ma.

(C) Drift and Cooling Phase initiation, 180 Ma.

(D) Louann Salt deposition, 170 Ma.

(E) Late Drift and Cooling Phase, 160 Ma.
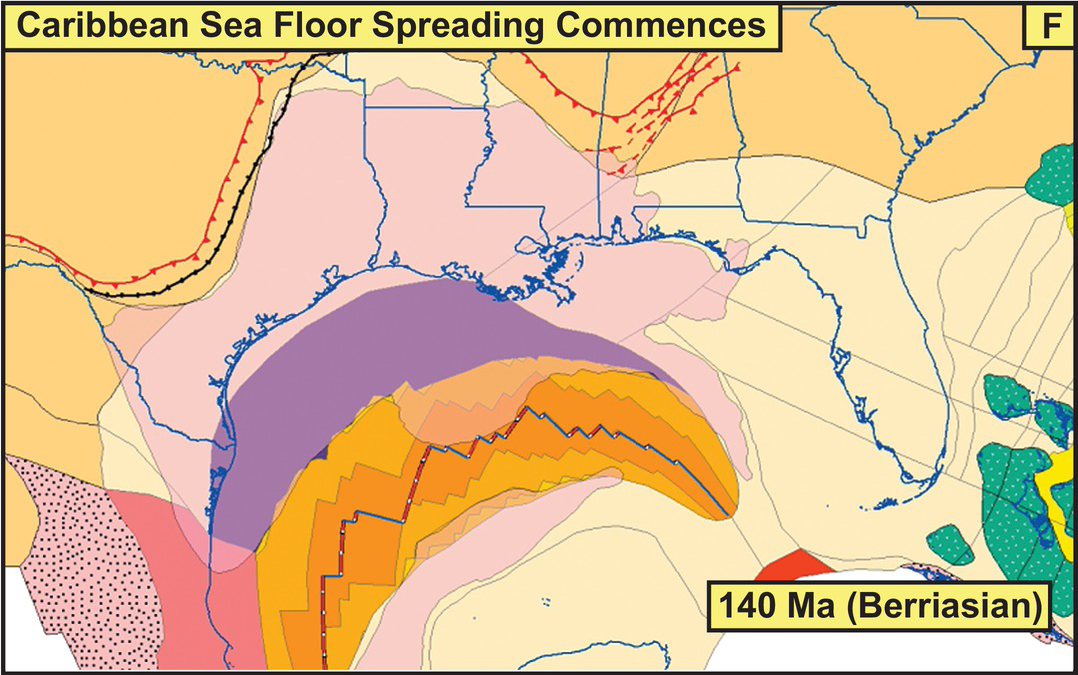
(F) Caribbean sea floor spreading commences, 140 Ma.
Figure 2.1 Plate tectonic reconstructions of Greater GoM, 240 Ma to 140 Ma.
The GoM basin opening was preceded by the Successor Basin-fill and Rift Phase associated with Pangea breakup (Figure 2.1) following the Marathon–Ouachita–Appalachian orogeny. By 240 Ma (Ladinian Stage of the Triassic), the Yucatán (Mayan block) had already joined with the North American plate, possibly bounded on the west by the Burgos lineament (Figure 2.1A). South America was located to the south and Africa to the east. Thick continental crust (orange) follows the Marathon–Ouachita belt from Mexico to Southern Arkansas, across Mississippi–Alabama to Georgia, where it reaches the Appalachians. Thinner transitional (light yellow) continental crust covered the future location of the GoM basin. Permian–Triassic igneous complexes are present in both Yucatán and onshore Mexico (Lawton et al. Reference Lawton, Bradford, Vega, Gehrels and Amato2009; Xaio et al. Reference Xaio, Zhao and Liu2017). Gaps in the plate model are shown in Mexico as later megashear motions are required to assemble that area.
Continental crust extension began as Pangea breakup commenced soon after, with continental rifting occurring in the eastern USA (e.g., South Georgia rift [SGR] system; Heffner Reference Heffner2013). Back-arc rifting initiated in Mexico around 236 Ma (Lawton et al. Reference Lawton, Ruiz Urueña, Solari, Ingersoll, Lawton and Graham2018), indicating the continued influence of Pacific margin subduction of the Farallon plate (Martini and Ortega-Gutiérrez Reference Martini and Ortega-Gutiérrez2016). The result was a series of right-lateral transtensional grabens filled with largely continental to marginal marine siliciclastics, as documented in onshore outcrops (Lawton et al. Reference Lawton, Ruiz Urueña, Solari, Ingersoll, Lawton and Graham2018). As will be discussed in Section 2.3.2, there is little evidence for rifting in east Texas and Louisiana, implying a more ductile lithospheric flexure response and ensuing deposition of the Eagle Mills here in a post-orogenic successor basin. Further, there is little room for pre-salt sedimentation on the northern Yucatán margin, a pattern that continues to at least 170–180 Ma.
By 200 Ma (Hettangian Stage of the Early Jurassic; Figure 2.1B), igneous activity initiated, manifested as surface lava flows and pyroclastics, as well as subsurface dykes and sills (Kidwell Reference Kidwell1951). These may be linked to magnetic anomalies in both onshore Texas and offshore Mexico (Mickus et al. Reference Mickus, Stern, Keller and Anthony2009). These events are roughly contemporaneous with emplacement of Central Atlantic magmatic province (CAMP) igneous intrusions, as well as igneous bodies in the SGR (Heffner Reference Heffner2013). Sometime later, seaward dipping reflections (SDRs), indicating massive basalt outflows, were emplaced in both the northeastern GoM and north of the Yucatán margin (cross-section 7, Figure 1.19). These underlie pre-salt sedimentary rocks, inferred from seismic character (Miranda Peralta et al. Reference Miranda Peralta, Alvarado and Villalón2014; Curry et al. Reference Curry, Peel, Hudec and Norton2018).
Between 200 Ma (Figure 2.1B) and 170 Ma (Figure 2.1D) Yucatán moved in a southeasterly direction relative to North America. The crust between Yucatán and North America is colored dark blue in Figure 2.1C. There is some uncertainty about the composition of this crust, as it could be extended continental crust as predicted by the refraction models of Van Avendonk et al. (Reference Van Avendonk, Christeson, Norton and Eddy2015) and Eddy et al. (Reference Eddy, Van Avendonk and Christeson2014, Reference Eddy, Avendonk, Christeson and Norton2018). Alternatively, as it lies outboard of the coastal magnetic anomalies (Mickus et al. Reference Mickus, Stern, Keller and Anthony2009), it may be oceanic crust. Because of the thick sediments and salt overlying this area at present, seismic imaging (both reflection and refraction) is challenging and the differing interpretations are equally plausible. Final resolution will require more special-purpose data acquisition. For our tectonostratigraphic scheme, this marks the effective end of the Successor Basin-Fill and Rifting Phase, though there is only a fragmentary sedimentary record and a substantial age gap with the next phase, as discussed in the following.
Several important implications from these plate reconstructions are relevant to sediment routing of the pre-salt (Eagle Mills) depositional systems. Early in the continental stretching phase, the tight fit of Yucatán with North America limited space for sediment accommodation (Figure 2.1B,C). The east Texas/Louisiana/Arkansas area is the widest embayment north of the plate boundary where Eagle Mills deposition is well documented. The structural boundary could have controlled sediment routing, as discussed below. Later separation of the Yucatán (Mayan block) during and after the speculative first phase of sea floor spreading or simple rift extension (Figure 2.1C) may also mean that newly developed space was available for development of pre-salt deposition in the area north of Yucatán subbasin (see Section 1.5.7 and cross-section 7, Figure 1.19). The period of sediment accommodation in the pre-salt Yucatán subbasin may have been short, but accumulation rates must have been high, given kilometer-scale thickness of the interval between the possible SDRs and the base of salt in Mexico (Saunders et al. Reference Saunders, Gieger, Rodriguez and Hargreaves2016; Hudec and Norton Reference Hudec and Norton2018).
At 170 Ma (Bajocian Stage of the Jurassic), we believe salt deposition commenced in the nascent Gulf basin (Figure 2.1D; Snedden et al. Reference Snedden, Norton, Hudec, Eljalafi and Peel2018c). Age dating of the Louann Salt is discussed further in Section 3.2.1. While a 170 Ma age for the Louann Salt is 7–8 million years earlier than previous estimates (e.g., Salvador Reference Salvador1987; Hudec et al. Reference Hudec, Norton, Jackson and Peel2013a), this is a time when the South and North American plates are in closer proximity than later on and thus conditions are more conducive to basin restriction and evaporation. This is also a time of well-documented sea floor spreading, beginning initially as an intrusive event below the original Louann Salt body (Norton et al. Reference Norton, Lawver, Snedden, Lowery and Snedden2016). The ensuing separation of the Campeche/Yucatán (Isthmian) salt bodies from the original Louann Salt body occurred as Yucatán rotated around a pole in the Florida Straits (e.g., Nguyen and Mann Reference Nguyen and Mann2016). Sea floor spreading transitions to an extrusive process, increasing the gap between salt bodies. This initiates the Middle Mesozoic Drift and Cooling Phase (Figure 1.24).
At 160 Ma (Oxfordian Stage of the Jurassic), the GoM basin opening reached a point where there is a direct, progressively widening connection to the world ocean through the Florida Straits to the Atlantic–Tethyan seas (Figure 2.1E). As will be discussed in Section 3.3.1, the transition from hypersaline basin water to more normal marine salinity may have taken more than five million years, as the first fully marine fauna and flora are found within the Upper Smackover Limestone (Godo Reference Godo, Merrill and Sternbach2017). Later gravity sliding in the northern Gulf allowed Louann (northern GoM) salt to overlap with oceanic crust in the central US Gulf basin, the so-called Walker Ridge salient (Hudec et al. Reference Hudec, Norton, Jackson and Peel2013a). However, seaward translation of the Campeche salt was probably limited by the BAHA high (hachured area in Figure 2.1D and E; Hudec and Norton Reference Hudec and Norton2018). Translation of various tectonic blocks along megashears in Mexico is thought to have continued assembly of Mexico south of the Tamaulipas Arch (Martini and Ortega-Gutiérrez Reference Martini and Ortega-Gutiérrez2016).
At 155 Ma to some time after 140 Ma, oceanic crust generation waned in the GoM, and sea floor spreading shifted to the Caribbean basin (Figure 2.1F). By 138 Ma, the Yucatán (Mayan) block has rotated into its present-day position, roughly coincident with a large influx of siliciclastics (Hosston and Travis Peak Formations) in the northern GoM (Galloway Reference Galloway and Hsu2008). This ends the Middle Mesozoic Drift and Cooling Phase. In several areas of the basin, angular unconformities or substantial lacunas mark the base of the Sligo–Hosston supersequence (McFarlan Reference McFarlan, Bebout and Loucks1977; Anderson Reference Anderson1979; Galloway Reference Galloway and Hsu2008; Ewing Reference Ewing2010).
The now fixed continental and oceanic crustal blocks in the GoM are soon affected by a series of sub-regionally focused crustal heating and uplift events that continue episodically until the end of the Cretaceous (Figure 1.24). Local crustal heating and igneous intrusions occur in south Texas, Louisiana, and Mississippi along with formation of angular unconformities in east Texas and the Mississippi Embayment (Ewing Reference Ewing2009). Collectively, we refer to this timespan as the Local Tectonic and Crustal Heating Phase (Figure 1.24). This phase continued until the end of the Mesozoic, when the Chicxulub impact event dramatically changed the land- and seascape of the greater GoM basin, paving the way for the Cenozoic.
Superimposed on plate and local tectonics described above are first-order sea-level variations, reflecting changes in mid-ocean ridge volumes and subduction of water, among other factors (Conrad Reference Conrad2013; Haq Reference Haq2014). Reexamination of Mesozoic sea-level variations in light of new chronostratigraphic information confirms prior work (Haq et al. Reference Haq, Hardenbol and Vail1987) that the Early Jurassic sea level began near present-day mean sea level, rising to a peak approximately 140 m above present-day mean sea level (pdmsl) in the Tithonian, and then stabilizing around 100–120 m above pdmsl until the Early Cretaceous (Haq Reference Haq2017). A trough (80 m above pdmsl) in the Cretaceous worldwide sea-level curve in the Mid-Valanginian stage is followed by peaks in the Barremian and highest point (250 m above pdmsl) just above the Cenomanian–Turonian boundary (Haq Reference Haq2014). As discussed in subsequent sections, the GoM Mesozoic record shows a variable response to these global sea-level changes, suggesting the stratigraphic record here is a convolved archive of tectonics and eustacy in a high sediment supply setting.
2.3 Tectonostratigraphic Models for Basin Precursor History
The general approach taken throughout this book is to present depositional models for the GoM that are supported by a preponderance of data currently available. The fragmentary sedimentary record that post-dates the breakup of Pangea but prior to formation of the basin and deposition of the Louann Salt is sufficiently unclear that we need to consider two alternative tectonostratigraphic models for the early Mesozoic (Figure 2.2A,B). The first is based on the more conventional model described in detail by Salvador (Reference Salvador1987, 1991; Figure 2.2A) and the other is a newly developed concept that departs from the conventional model both in terms of timing and kinematics (Figure 2.2B). This alternative model (Figure 2.2B) is based on new data on plate reconstructions (Section 2.2), seismic reflection data (Section 2.3.2), and detrital zircon provenance work (Section 2.3.3).


(B) New model for pre-salt, suggesting sedimentation in the central GoM fills a large-scale post-collision successor basin. Map restored to 240 Ma (courtesy I. Norton and UT PLATES project). Sediment routing trends based on key wells analyzed for detrital zircon geochronology as shown in Figure 2.6 are (1) Rizer #1; (2) McGee Unit 1; (3) McDonnell B3; (4) Exxon LV Ray Unit 1–2; (5) Superior McManus; (6) Amoco Stumberg. Potosi Fan outline from Dickinson et al. (Reference Dickinson, Gehrels and Stern2010).

(C) New model restored to 170 Ma, showing interpreted Mexico pre-salt province (light green polygon) and overlying Louann Salt just prior to initiation of sea floor spreading. The Mexico pre-salt province lies between the Yucatán shelf margin and the salt separation line (terminology of Hudec et al.Reference Hudec, Norton, Jackson and Peel2013a).
Both models agree on the precursor to breakup, collision of Gondwana (South America, Africa, and Yucatán) with North America at the end of the Paleozoic to create Pangea (Figure 2.2). Deformation is recorded at the northern boundary to what will become the GoM basin, observed as a series of northward-directed thrust faults and foreland basin from the Marathon orogenic belt through the Ouachita Mountains to the Appalachians. Exposures of Pennsylvanian strata in the present-day Ouachita Mountains of Arkansas are an excellent archive for reconstructing this tectonic episode (Gleason et al. Reference Gleason, Gehrels, Dickinson, Patchett and Kring2007; Ewing Reference Ewing2016). In Texas, the Ouachita system is largely deeply buried below Mesozoic strata. A comprehensive analysis of wells penetrating the Paleozoic deformation is presented in the seminal work of Flawn et al. (Reference Flawn, Goldstein, King and Weaver1961). There are a few shallow outliers of this deformation, including the Sabine Island at the Texas–Louisiana border (see Section 3.4.2). Deep subthrust tests were drilled as recently as 1995 that tested the Ordovician Ellenburger below multiple thrust duplex structures. The Shell #1 Barrett well, drilled in Hill County (Well A in Figure 2.2A) tested a deep sub-Cretaceous structure called the Waco Uplift, which turned out to be largely Paleozoic metasediments (Rozendal and Erskine Reference Rozendal and Erskine1971; Vernon Reference Vernon1971; Nicholas and Waddell Reference Nicholas and Waddell1989). Pennsylvanian deformation transitioned to a period of subsidence in the Permian basin, accumulating as much as 14,000 feet (4270 m) of Permian strata in west Texas (Ewing Reference Ewing2016), though we logically exclude this interval from the GoM depositional fill.
What happens following the end of the Permian subsidence remains a matter of conjecture, due to the fragmentary stratigraphic record, with few early Mesozoic outcrops outside of Mexico or south of the Ouachita Mountains, and just a handful of wells drilled below the autochthonous Louann Salt in the onshore USA, particularly in the western part of the future GoM basin. General consensus among researchers suggests that the breakup of Pangea initiated in the early Triassic and separation of North and South America followed the Laurentian suture that can be traced from the Appalachians to the Ouachita–Marathon belt into Mexico. A particular scientific conundrum is the 90 million year hiatus between the Permian strata of west Texas (roughly 251 Ma) and the oldest ages of fully marine Upper Smackover strata (157–160 Ma) recorded in the Middle Mesozoic Drift and Cooling Phase of the Gulf basin (see also Section 3.3.1).
Understanding these pre-Louann or “pre-salt” depositional patterns is important for several reasons. First, recent pre-salt discoveries have opened new exploration frontiers in Brazil and Angola and added large hydrocarbon reserves (Arbouille et al. Reference Arbouille, Andrus, Piperi and Xu2013). Second, newly acquired seismic data in deepwater Mexico has provided superb imaging of a newly identified pre-salt province off of northern Yucatán that has been considered for leasing by Commision de Nacional Hydrocarbons (CNH) (Saunders et al. Reference Saunders, Gieger, Rodriguez and Hargreaves2016). Further, in 2017 Pemex announced plans for drilling a deep test (Yaaxtaab-1) of the pre-salt interval in the Bay of Campeche (CNH 2017a). Thus, the pre-salt of the northern GoM may be a depositional or tectonic analog for this new exploration frontier, as described in Section 1.5.7 and illustrated in Figure 1.20.
2.3.1 The Conventional GoM Early Mesozoic Rift Model
The presence of a post-Paleozoic, pre-Louann interval has been known in the northern GoM since the 1930s (Weeks Reference Weeks1938; Scott et al. Reference Scott, Hayes and Fietz1961; Gawloski Reference Gawloski1983; Salvador Reference Salvador1987, Reference Salvador and Salvador1991b). Lithologies include red to greenish-gray shales and white sandstones and red dolomites (Woods and Addington Reference Woods and Addington1973). Red bed successions, known as the Eagle Mills Formation (named after a well in Arkansas) have been encountered in a large number of oil and gas and even water wells (Salvador Reference Salvador and Salvador1991b). The uncertainty of a Permian or Triassic age was resolved, in part, by the identification of a single leaf fossil (Macrotaeniopteris magnifolia) in the Humble #1 Royston, of Arkansas (Scott et al. Reference Scott, Hayes and Fietz1961). The same leaf fossil is present in the Chinle Formation of Arizona and in the Newark Supergroup of Virginia. Later palynological analysis of the fossil algae Coenobium plaesiodictyon in a Cass County, Texas well confirmed a Triassic (Carnian) age for the Eagle Mills (Wood and Benson Reference Wood and Benson2000). Fossil plants from red beds of the Eagle Mills equivalent La Boca Formation (Huizachal Group) in northern Mexico are less diagnostic, broadly indicating a Late Triassic to Early Jurassic age (Mixon Reference Mixon1963).
Linkage to the red beds of the Newark Supergroup is also appealing on the basis of lithology and tectonic process (Salvador Reference Salvador and Salvador1991b). A model of the Eagle Mills red beds filling a series of discrete rift and graben systems during the Pangea breakup was adapted not just for the eastern GoM but the basin as a whole (Salvador Reference Salvador and Salvador1991b; Figure 2.2A). This has evolved into what may be described as the conventional temporal model for the nascent GoM basin. In this chronostratigraphic scheme, rifting began soon after the end of the Permian (240 Ma), extending to about 205 Ma where there is a large (40 million year) hiatus until post-rift deposition of the Louann Salt beginning around 162 Ma (Salvador Reference Salvador1987, Reference Salvador and Salvador1991b). The cause of the missing stratal interval is unclear, though it has been suggested that rifting was continuous until salt deposition, but shifted to the area under the present-day salt canopy where there are no well penetrations below autochthonous salt.
The Wood River Formation of the South Florida basin has yielded zircons with a maximum depositional age of 195–235 Ma from U–Pb analyses, but only partially covering the stratigraphic gap (Wiley Reference Wiley2017). However, the zircon sample counts of Wiley (Reference Wiley2017) also tend to be low (often less than n = 100 per sample), raising questions about statistical significance of the results. The age of the Louann Salt may also be older than Callovian, as recent 87/86Sr analysis has suggested an age approaching 170 Ma (see Section 3.2.1). Nonetheless, this gap of 90 million years or more remains puzzling. South Florida basin zircons show an affinity with Gondwana sources (Suwannee terrane), indicating proximity to the African continent, a pattern that continues into the Oxfordian (Lovell and Weislogel Reference Lovell and Weislogel2010; Lisi Reference Lisi2013; Wiley Reference Wiley2017).
North of the Ouachita–Marathon orogenic belt, outcrops of the Dockum Group stand in stark contrast to the entirely subsurface Eagle Mills of Texas. The Dockum Group and equivalent units of the Chinle outcrop in a belt from north Texas to Nevada, a distance of 2000 km, allowing detailed sedimentological analysis, paleocurrent measurements, and provenance work using detrital zircon (Mickus et al. Reference Mickus, Stern, Keller and Anthony2009; Dickinson et al. Reference Dickinson, Gehrels and Stern2010). Paleocurrents show fluvial transport to the northwest, likely coming from source terranes in the paleotopographic highs of the Ouachita orogenic belt on the south (Thomas Reference Thomas2011). Provenance analysis using U–Pb zircons shows expected Grenville sources from the Ouachita orogenic belt, with mixtures from other terranes (Dickinson and Gehrels Reference Dickinson and Gehrels2008). Zircon analysis shows maximum depositional ages of 200–234 Ma (Umbarger, Reference Umbarger2018), maintaining the enigmatic hiatus between the Louann Salt and the Triassic interval of the basin.
In Mexico, the Triassic to Middle Jurassic record, mainly archived in outcrop intervals, includes the Zacatecas, Nazas, and La Joya Formations of Mesa Central and Huizachal Group of the Sierra Madre Oriental (Barboza-Gudiño et al., Reference Barboza-Gudiño, Zavala-Monsiváis, Venegas-Rodríguez and Barajas-Nigoche2010). The Potosi submarine fan is believed to be connected to the El Alamar paleo-river of the Huizachal Group, influenced by the tectonics of the east Mexico Permo-Triassic continental arc (Stern and Dickinson Reference Stern and Dickinson2010).
2.3.2 Alternative Model for Early Mesozoic Successor Basin-Fill and Rifting
Several new and even some older observations are inconsistent with the long-standing, conventional model of Salvador (1991). Closer examination of seismic data from Arkansas, Louisiana, and Texas in the area of Salvador’s (1991) graben trend fails to show unequivocal evidence of a buried rift system (Figures 2.3 and 2.4). In north Texas, the Eagle Mills onlaps the deformed Top Paleozoic interval (see also Milliken Reference Milliken1988), suggesting depositional infilling of preexisting accommodation, not rift-grabens (Figure 2.5). Toplap stratal terminations against the base of the Louann Salt indicate a disconformable contact, not an angular unconformity. The Mexia–Talco fault zone, a breakaway fault zone at the landward termination of the salt, is noted just southward of the crest of the Waco Uplift (Figure 2.3).
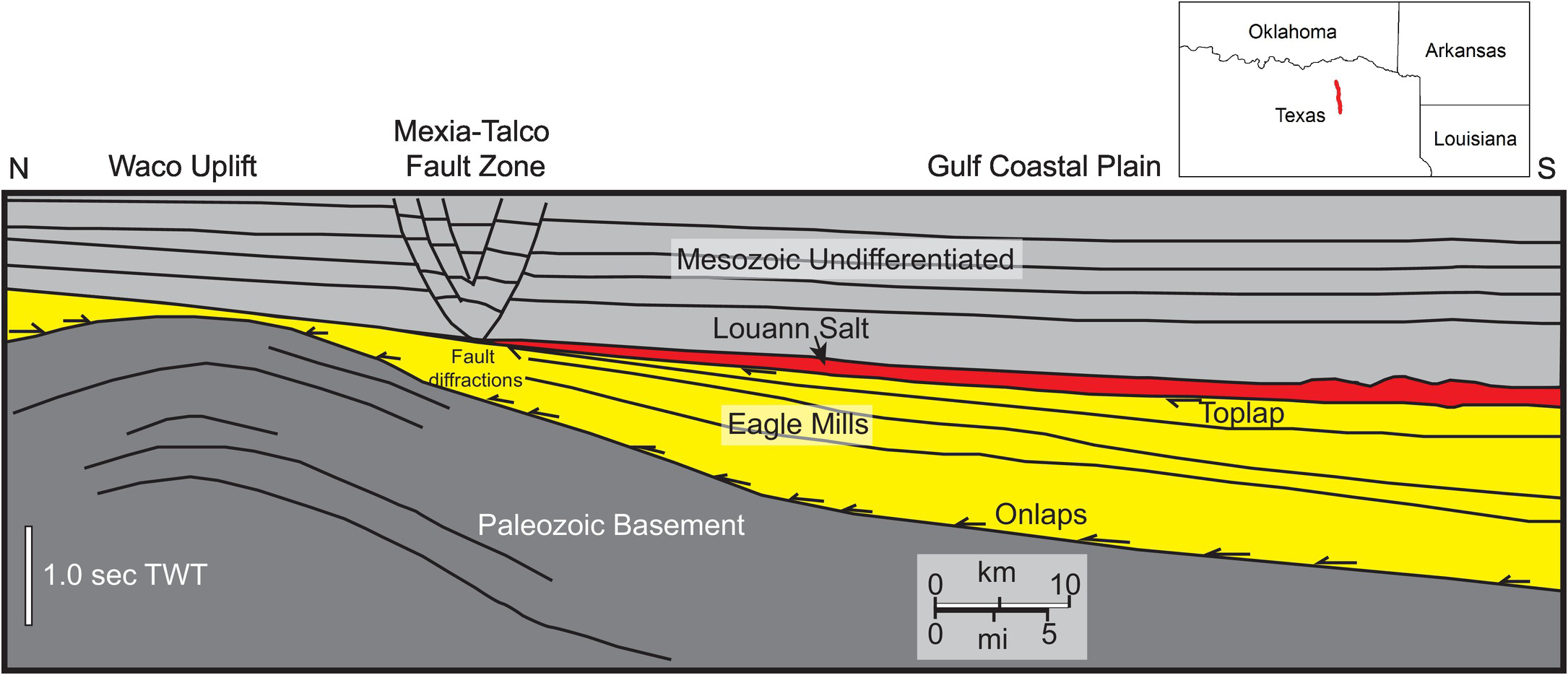
Figure 2.3 Cross-section across north Texas including the Waco Uplift. The cross-section shows Eagle Mills onlapping the Paleozoic basement structure, thinning onto the Waco Uplift, and is toplapped by the base Louann Salt.

Figure 2.4 Seismic line interpretation across east Texas area including Exxon-Fina LV Ray well of Upshur, County, Texas Waco.

Figure 2.5 Eagle Mills isopach map based on well control and seismic mapping. Contours in feet.
Reviewing the original illustrations of the block-faulted Triassic strata in Arkansas used by Scott et al. (Reference Scott, Hayes and Fietz1961) and later repeated by Woods and Addington (Reference Woods and Addington1973), it is apparent that these models are not matched by seismic interpretations in the area. For example, a seismic profile across southwestern Arkansas depicted by Nicholas and Waddell (Reference Nicholas and Waddell1989) shows the Eagle Mills is only separated from the underlying Pennsylvanian interval by a disconformity with no obvious rifting or erosional surface. Further, there is no system of half-grabens as seen in the SGR (e.g., Heffner Reference Heffner2013).
In east Texas, Exxon and Fina jointly drilled the LV Ray GU 1–2 well below allochthonous salt and encountered the Eagle Mills siliciclastic interval, reaching total depth (TD) at 18,498 ft (5640 m; White et al. Reference White, Blanke and Clawson1999; Figure 2.4). Based on limited age information and long-distance correlation to the Mexico and the eastern USA Newark Supergroup, a rift model was proposed, albeit one with marine flooding of the axial portion of the rift system in east Texas to form a restricted marine evaporite unit within the Eagle Mills, called the Rosewood after a local field name (White et al. Reference White, Blanke and Clawson1999). The syn-rift model offered by White et al. (Reference White, Blanke and Clawson1999) emphasizes a half-graben structural morphology, based on older 2D seismic data from the field area. Review of newly reprocessed regional 2D seismic lines that tie LV Ray GU 1–2 well and nearby Eagle Mills penetrations does not show a rift system half-graben morphology (Figure 2.4). The Eagle Mills (pre-salt) interval appears to drape an irregular deep (Paleozoic?) basement topography. An angular unconformity between the shallower Paluxy–Washita supersequence and overlying Eagle Ford–Tuscaloosa supersequence coincides with the basement high point. Thus, part of the basement structure post-dates the Eagle Mills (Figure 2.4), probably related to salt evacuation. The Louann Salt has a roughly similar morphology to the Eagle Mills, thickening into lows and thinning onto basement highs. Faulting is not common in the deep interval, with later folding, rotation, and shallow salt evacuation more apparent. Little syntectonic thickening into the rare observed faults is evident.
Examination of the cuttings from the Rosewood evaporite interval reveals an alternation of thin-bedded anhydrites and gray siltstones rather than a single thick evaporite unit as depicted by White et al. (Reference White, Blanke and Clawson1999). In contrast to conventional views of the Eagle Mills (Salvador Reference Salvador1987, Reference Salvador and Salvador1991b) red beds are rare in the Eagle Mills here as dark gray siltstones are more common to the pre-salt interval. Detrital zircon U–Pb geochronology from the Eagle Mills interval just below the Louann Salt in this well is discussed in Section 2.3.3.
Milliken (Reference Milliken1988) mapped a large area of northeast Texas using a grid of older 2D seismic data. His Eagle Mills isopach map (Figure 2.5) shows a general basinward thickening, with local patterns following Paleozoic basement trends, for example, thinning over the Sabine Uplift and thickening in the east Texas salt basin. No expanded asymmetrical isopach thicks in half-grabens are evident. The erosional limit of the Eagle Mills on the west side of the successor basin follows but is well south of the Ouachita frontal thrust.
These observations of the deep Eagle Mills structure of the central GoM onshore contrast with the well-documented South Georgian rift system (Heffner Reference Heffner2013). In the SGR, half-grabens with changing polarity are the norm, with prominent syn-rift expansion and stratal rotation (Withjack et al. Reference Withjack, Schlische, Olsen, Renault and Ashley2002). Continental red beds are common in the SGR, as seen in the deep Rizer #1 well of South Carolina (Goggin and Rine Reference Goggin and Rine2014; Rine Reference Rine2014; Rine et al. Reference Rine, Hollon, Fu, Houghton and Waddell2014).
Salvador (Reference Salvador and Salvador1991b) and Thomas (Reference Thomas2011) show a significant offset in the trend of the Iapetan rifted margin of southern Laurentia. The SGR-graben systems shift along a major transform (called the Bahamas fracture zone), in order to link with the Texas–La–Ark rift system to the west (Figure 2.3). This offset, later called the Florida Lineament, must be considered highly interpretative as it is loosely based on alignment with onshore trends such as the Pickens–Gilbertown–Pollard fault system.
An alternative hypothesis, first described by Norton et al. (Reference Norton, Lawver and Snedden2018), is based on the concept that a significant portion of the Eagle Mills of the Texas–La–Ark area (Ouachita Embayment of Thomas Reference Thomas2011) is not filling a rift system of half-grabens but instead a successor basin or set of basins developed on the post-collision Ouachita structural surface. As discussed earlier, Eagle Mills strata of the Ouachita Embayment appear to dip monoclinally toward the south, presumably to a pinch-out or termination where the Yucatán block meets North America (e.g., Figure 2.3). This idea is also consistent with models suggesting a soft collision of Gondwana with Laurentia in the central GoM onshore, in contrast to the hard collision elsewhere (Heffner Reference Heffner2013). Further, it mirrors the observed accommodation pattern of the Dockum Group that appears to fill a low-relief depositional basin to the northwest of the uplifted Ouachita highlands source terrane (Riggs et al. Reference Riggs, Lehman, Gehrels and Dickinson1996; Dickinson and Gehrels Reference Dickinson and Gehrels2008). Dickinson et al. (Reference Dickinson, Gehrels and Stern2010) suggested that Triassic uplift of this central Texas pre-rift area was mainly a thermal precursor to the Jurassic opening of the GoM basin.
Several important implications emerge from the interpretation that the SGR system does not extend into the Louisiana–Arkansas–Texas area (Figure 2.2B). It is likely that the drainage systems of the SGR and central GoM onshore are not shared, axially or otherwise. This is confirmed by U–Pb age spectra (Figure 2.6; Section 2.3.3) showing at least two different source terranes and thus differing catchments and sediment routing patterns. It follows, then, that drainage catchments in Louisiana–Arkansas–Texas accessed a variety of source terranes and sediment routing was unconstrained by rift-graben topography. The influence of the North Atlantic basin opening (Withjack et al. Reference Withjack, Schlische and Olsen1998; Schlische Reference Schlische, LeTourneau and Olsen2003) apparently did not extend to areas west of the SGR. Thus, it is our conclusion that central Gulf onshore pre-salt deposition is more a reflection of the deposition upon the deformed Ouachita belt, as a post-orogenic successor basin-fill.
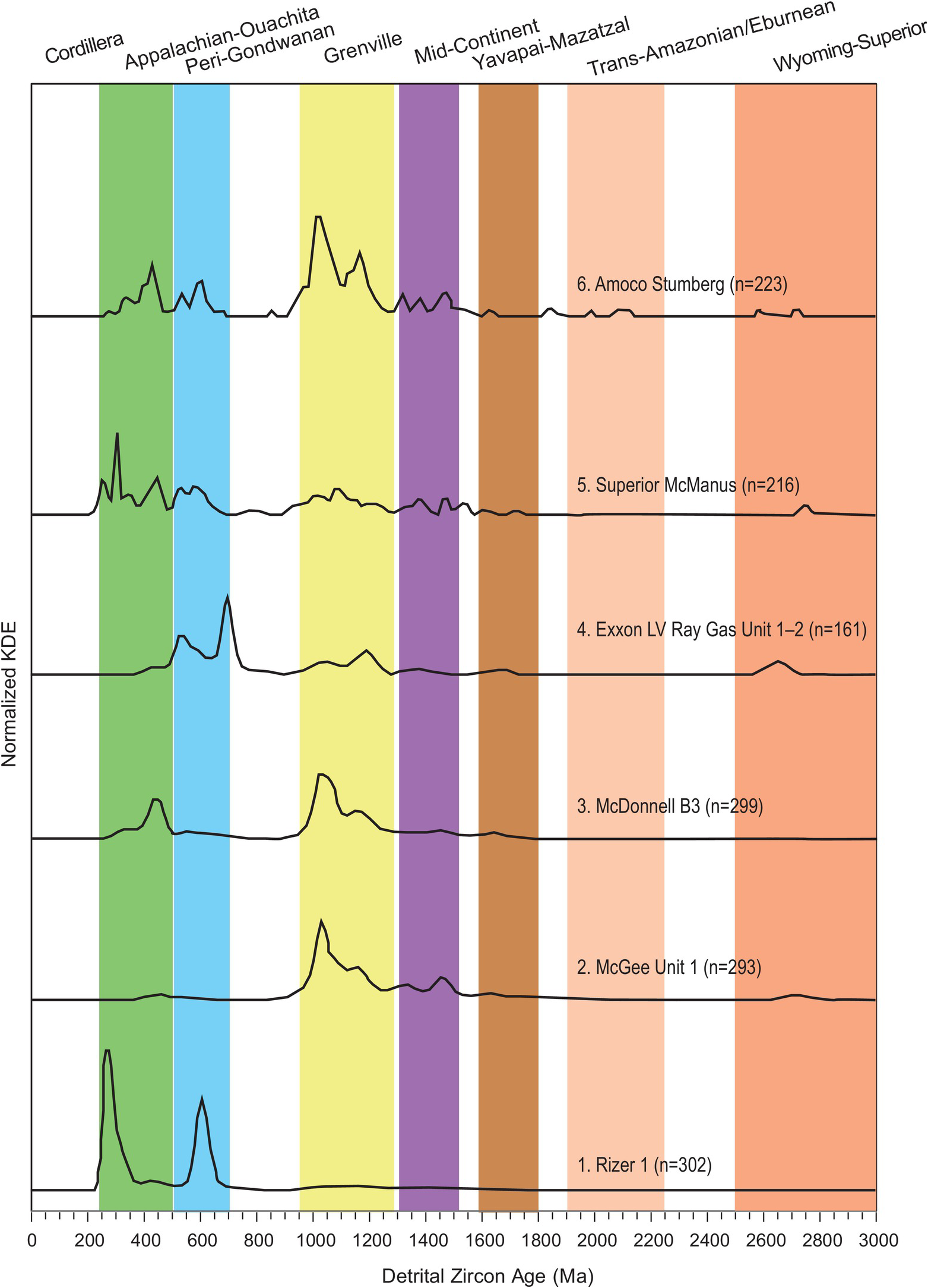
Figure 2.6 Comparison of detrital zircon age spectra from among Eagle Mills well penetrations in the northern GoM.
An alternative temporal sequence of events extending from the breakup of Pangea to the rift–drift transition is shown in Figure 2.2B. The seismic observations from east Texas and Louisiana are more consistent with this alternative framework than the conventional view of GoM rifting as described by Salvador (Reference Salvador and Salvador1991b) and other authors. A revised model for the pre-salt (Eagle Mills) interval, founded on the plate tectonic evolution described in Section 2.2 (Figure 2.1) would follow this progression:
1. Pangea breakup and continental stretching results in development of successor basins in the central GoM, reflecting a more ductile lithospheric response than elsewhere in Mexico or the eastern GoM (Figure 2.2B). Like the roughly contemporaneous Dockum Group, Eagle Mills deposition in the central GoM onshore initiated with the Late Triassic uplift of a central Texas Ouachita source terrane (Mickus et al. Reference Mickus, Stern, Keller and Anthony2009; Dickinson et al. Reference Dickinson, Gehrels and Stern2010), but other basement terranes were exposed. Eagle Mills sediments were routed to the drainage to the south, east, and west, bounded by the Yucatán block and magmatic material between Yucatán and North America (Figure 2.2B). The southern limit of central GoM Eagle Mills deposition is defined by the Gulf Coast Magnetic Anomaly, thought to indicate the Early Jurassic volcanic margin–rift axis (Mickus et al. Reference Mickus, Stern, Keller and Anthony2009). Catchments of interior drainage systems extended across a variety of North American basement source terranes, as evidenced in the diverse U–Pb age spectra from pre-salt wells (Section 2.3.3). The continental divide was located at the uplifted Marathon–Ouachita belt; Dockum Group and Chinle equivalent fluvial systems fed sediment to the north and west. Thus, a large portion of the Eagle Mills of the Central GoM is successor basin sedimentation, westward of and separate from the SGR. Some early but local rifting initiated in northern Mexico, with deposition of the Cerro El Carrizalillo Member of the Plomosas Formation in a half-graben (Lawton et al. Reference Lawton, Ruiz Urueña, Solari, Ingersoll, Lawton and Graham2018). Maximum depositional age from U–Pb zircons here is 236 ± 1 Ma (Figure 1.24). This may also be related to back-arc effects from the Pacific plate, unrelated to GoM opening sensu stricto (Martini and Ortega-Gutiérrez Reference Martini and Ortega-Gutiérrez2016). Rifting in eastern North America soon commenced, including the SGR, and continued from 240 Ma to 200 Ma (Heffner Reference Heffner2013). Deposition of the Eagle Mills and Dockum Group proceeded through the Norian Stage in Texas and adjacent areas (Dickinson et al. Reference Dickinson, Gehrels and Stern2010).
2. GoM continental stretching in a northwest–southeast direction caused southward migration of the Yucatán block (Figure 2.2C). This in turn created space and accommodation for basalt outflows (seaward dipping reflections) and later pre-salt sedimentary deposits in the offshore northeastern Gulf and Yucatán northern margin (see also Section 1.5.7, cross-section 7, Figure 1.19). In northern Mexico, back-arc rift-graben filling depositional units contain earliest dated volcanic U–Pb zircons of 191–193 Ma (Martini and Ortega-Gutiérrez Reference Martini and Ortega-Gutiérrez2016). In the northern GoM, rifting was limited to the area south of the present-day north-central GoM shelf that was later deeply buried below the Cenozoic strata (Van Avendonk et al. Reference Van Avendonk, Christeson, Norton and Eddy2015).
3. Massive Central Atlantic Magmatic Province (CAMP) volcanism began, signaling termination of rifting in the Atlantic basin and initiation of sea floor spreading there at 200 Ma (Olsen Reference Olsen1997; Figure 2.1B).
4. By contrast, the main phase of sea floor spreading in the northern GoM commenced about 30 million years later than in the Atlantic, initiated around 170 Ma (Figure 2.1D). This is a clear indication of temporal separation of the plate tectonic processes and thus sedimentary processes in the GoM and North Atlantic basins.
5. Pre-salt deposition in northern Yucatán forms a seaward dipping wedge of continental deposition derived from erosion of exposed Yucatán basement (Figure 2.2C). Plate reconstructions suggest sedimentation here terminated around 170 Ma, coincident with initiation of major sea floor spreading.
6. Rifting persisted in northern Mexico onshore areas with ignimbrites in graben-fills dated as young as 176 ± 1 Ma (Lawton et al. Reference Lawton, Ruiz Urueña, Solari, Ingersoll, Lawton and Graham2018; Figure 1.24). Alternatively, syntectonic deposition here was related to transtensional motion as various Mexican basement blocks moved into position. Movement of the Mayan block allowed pre-salt deposition in the northern Yucatán margin, forming a seaward dipping wedge of continental deposition likely derived from erosion of exposed Yucatán basement.
2.3.3 Pre-salt (Eagle Mills) Sediment Routing
U–Pb zircon analyses, an advanced provenance technique (see Box 1.2), from northern GoM pre-salt wells reveals the complexity of sediment sourcing from various basement terranes, as well as differences between the central GoM onshore and the SGR (Figure 2.6). The typical SGR geochronological signature is that of Appalachian/Allegheny sources, as indicated by a peak at 250 Ma and a peri-Gondwana (Suwannee terrane) peak at 650 Ma (e.g., Rizer #1 well; Figure 2.6). Wells representing the central northern GoM onshore successor basin-fill (e.g., McDonnell B3 and McGee Unit 1; Figure 2.6) show prominent peaks in the 1000–1250 Ma range, indicative of Grenville basement sources (cf. Blum and Pecha Reference Blum and Pecha2014). By contrast, this Laurentian basement signature is absent in the Rizer #1 well. Pre-salt strata in the Exxon LV Ray Gas Unit 1–2 has a weak Grenville signature and a significant pan-African (peri-Gondwanan) set of peaks on the detrital zircon age spectra (Figure 2.6).
These age spectra support the view that the SGR and central northern GoM onshore pre-salt (Eagle Mills) interval was deposited by multiple drainage systems, some of which were probably small but steep rivers (Figure 2.2B). Even within the central northern GoM onshore pre-salt province, distinct differences over short distances indicate contributions of different tributaries whose catchment headwaters were anchored in different source terranes. On the southwestern margin is the Superior McManus #1 well (well 5 in Figure 2.2B) that shows contributions from multiple sources, but also confirms paleoflow to the southwest along the Yucatán/North America plate boundary (Figure 2.2B). Sediment drainage systems probably converged at the effective sedimentary boundary (northern GoM sedimentary limit of Figure 2.2B), defined by the volcanic margin coincident with the Gulf Coast Magnetic Anomaly (Mickus et al. Reference Mickus, Stern, Keller and Anthony2009). This wide dispersion of pre-salt sediments is at odds with the conventional model of a more focused, half-graben controlled thickness trend that would be expected in a classic rift system.
The Amoco Stumberg well, located in Dimmit County, Texas near the Mexico border, has some similarities with late collisional succession formed prior to Eagle Mills deposition (Figure 2.6). Age spectra are comparable to the Pennsylvanian Age Haymond Formation of the Marathon basin (cf. Gleason et al. Reference Gleason, Gehrels, Dickinson, Patchett and Kring2007), suggesting contributions from the north. Yet the Amoco Stumberg detrital zircon geochronology also has similarities to that of the Superior McManus age spectra, with aligned peaks of Appalachian, peri-Gondwanan, and Mid-Continent terranes. It is therefore possible that both wells also reflect a Yucatán basement block source, with prominent peri-Gondwanan peaks in the range of 400–600 Ma. This contrasts with earlier work suggesting that the Potosi fan, as sourced by the Yucatán block, was limited to drainage systems south of the Tehuantepec paleotransform (Ortega-Flores et al. Reference Ortega-Flores, Solari, Lawton and Ortega-Obregón2014). The Amoco Stumberg and Superior McManus wells detrital zircon age spectra thus may point to a long-lived Yucatán source terrane feeding both Mexico and south Texas (Figure 2.2B). These fluvial networks may have served as tributaries for the El Alamar paleo-river (Barboza-Gudiño et al. Reference Barboza-Gudiño, Zavala-Monsiváis, Venegas-Rodríguez and Barajas-Nigoche2010) that fed the Potosi submarine fan at the paleo-Pacific margin (B. Frederick, pers. comm.) More detailed statistical analyses are currently underway (Frederick et al. Reference Frederick, Blum and Sneddenin review).
As explained in the alternative model discussion (Section 2.3.2), the pre-salt interval of the northern Yucatán subbasin is considerably younger than the Eagle Mills of the northern GoM, as accommodation for this deposition was probably not available until Yucatán had rotated sufficiently to create space between the Yucatán shelf and North America (Figure 2.2C). Prior emplacement of the possible SDRs, as noted on cross-section 7 (Figure 1.19), likely around 190–200 Ma, does help constrain the basal age of this younger pre-salt interval. This younger age of the Mexico pre-salt deposition, plus its location well away from higher heat flow continental crust, are both more favorable conditions for higher reservoir quality than commonly observed in the northern GoM.