Book contents
- Genome Editing and Engineering
- Genome Editing and Engineering
- Copyright page
- Dedication
- Contents
- Contributors
- Foreword
- Preface
- Part I Biology of Endonucleases (Zinc-Finger Nuclease, TALENs and CRISPRs) and Regulatory Networks
- Part II Genome Editing in Model Organisms
- Part III Technology Development and Screening
- 11 CRISPR Genome Editing in Mice
- 12 Detection of Insertion/Deletion (Indel) Events after Genome Targeting: Pros and Cons of the Available Methods
- 13 Application of TAL Proteins and the CRISPR System to Purification of Specific Genomic Regions for Locus-specific Identification of Chromatin-associated Molecules
- 14 Application of CRISPR for Pooled, Vector-based Functional Genomic Screening in Mammalian Cell Lines
- 15 Generation and Utilization of CRISPR/Cas9 Screening Libraries in Mammalian Cells
- Part IV Genome Editing in Stem Cells and Regenerative Biology
- Part V Genome Editing in Disease Biology
- Part VI Legal (Intellectual Property) and Bioethical Issues of Genome Editing
- Index
- Plate Section (PDF Only)
- References
11 - CRISPR Genome Editing in Mice
from Part III - Technology Development and Screening
Published online by Cambridge University Press: 30 July 2018
- Genome Editing and Engineering
- Genome Editing and Engineering
- Copyright page
- Dedication
- Contents
- Contributors
- Foreword
- Preface
- Part I Biology of Endonucleases (Zinc-Finger Nuclease, TALENs and CRISPRs) and Regulatory Networks
- Part II Genome Editing in Model Organisms
- Part III Technology Development and Screening
- 11 CRISPR Genome Editing in Mice
- 12 Detection of Insertion/Deletion (Indel) Events after Genome Targeting: Pros and Cons of the Available Methods
- 13 Application of TAL Proteins and the CRISPR System to Purification of Specific Genomic Regions for Locus-specific Identification of Chromatin-associated Molecules
- 14 Application of CRISPR for Pooled, Vector-based Functional Genomic Screening in Mammalian Cell Lines
- 15 Generation and Utilization of CRISPR/Cas9 Screening Libraries in Mammalian Cells
- Part IV Genome Editing in Stem Cells and Regenerative Biology
- Part V Genome Editing in Disease Biology
- Part VI Legal (Intellectual Property) and Bioethical Issues of Genome Editing
- Index
- Plate Section (PDF Only)
- References
Summary
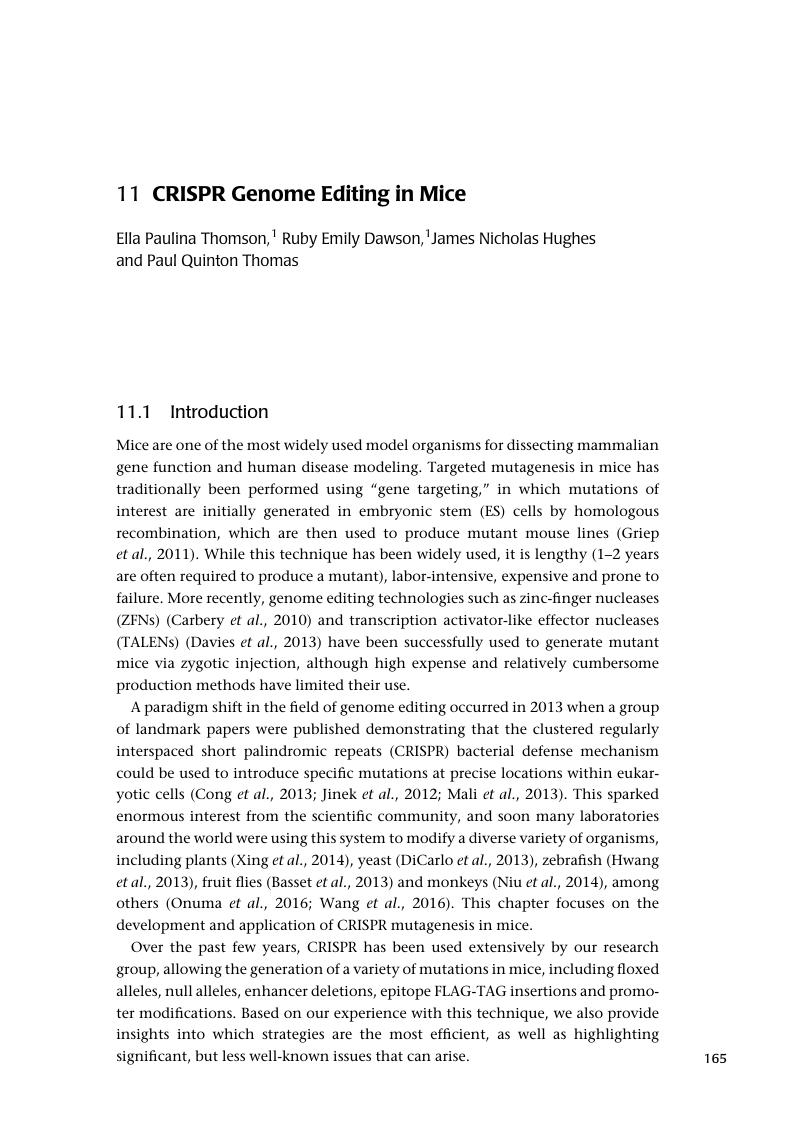
- Type
- Chapter
- Information
- Genome Editing and EngineeringFrom TALENs, ZFNs and CRISPRs to Molecular Surgery, pp. 165 - 180Publisher: Cambridge University PressPrint publication year: 2018