Book contents
- Fetal Therapy
- Fetal Therapy
- Copyright page
- Dedication
- Contents
- Contributors
- Foreword
- Section 1: General Principles
- Section 2: Fetal Disease: Pathogenesis and Treatment
- Red Cell Alloimmunization
- Structural Heart Disease in the Fetus
- Fetal Dysrhythmias
- Manipulation of Fetal Amniotic Fluid Volume
- Fetal Infections
- Fetal Growth and Well-being
- Preterm Birth of the Singleton and Multiple Pregnancy
- Complications of Monochorionic Multiple Pregnancy: Twin-to-Twin Transfusion Syndrome
- Complications of Monochorionic Multiple Pregnancy: Fetal Growth Restriction in Monochorionic Twins
- Complications of Monochorionic Multiple Pregnancy: Twin Reversed Arterial Perfusion Sequence
- Complications of Monochorionic Multiple Pregnancy: Multifetal Reduction in Multiple Pregnancy
- Fetal Urinary Tract Obstruction
- Pleural Effusion and Pulmonary Pathology
- Surgical Correction of Neural Tube Anomalies
- Fetal Tumors
- Congenital Diaphragmatic Hernia
- Fetal Stem Cell Transplantation
- Gene Therapy
- Section III: The Future
- Index
- References
Fetal Infections
from Section 2: - Fetal Disease: Pathogenesis and Treatment
Published online by Cambridge University Press: 21 October 2019
- Fetal Therapy
- Fetal Therapy
- Copyright page
- Dedication
- Contents
- Contributors
- Foreword
- Section 1: General Principles
- Section 2: Fetal Disease: Pathogenesis and Treatment
- Red Cell Alloimmunization
- Structural Heart Disease in the Fetus
- Fetal Dysrhythmias
- Manipulation of Fetal Amniotic Fluid Volume
- Fetal Infections
- Fetal Growth and Well-being
- Preterm Birth of the Singleton and Multiple Pregnancy
- Complications of Monochorionic Multiple Pregnancy: Twin-to-Twin Transfusion Syndrome
- Complications of Monochorionic Multiple Pregnancy: Fetal Growth Restriction in Monochorionic Twins
- Complications of Monochorionic Multiple Pregnancy: Twin Reversed Arterial Perfusion Sequence
- Complications of Monochorionic Multiple Pregnancy: Multifetal Reduction in Multiple Pregnancy
- Fetal Urinary Tract Obstruction
- Pleural Effusion and Pulmonary Pathology
- Surgical Correction of Neural Tube Anomalies
- Fetal Tumors
- Congenital Diaphragmatic Hernia
- Fetal Stem Cell Transplantation
- Gene Therapy
- Section III: The Future
- Index
- References
Summary
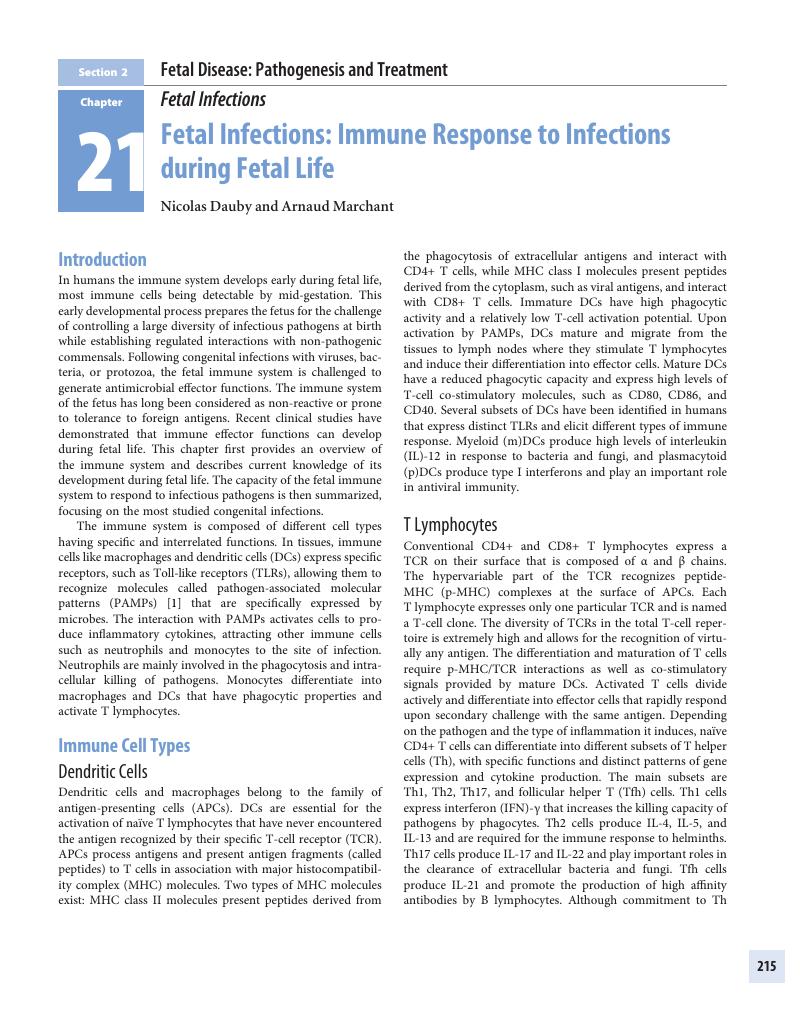
- Type
- Chapter
- Information
- Fetal TherapyScientific Basis and Critical Appraisal of Clinical Benefits, pp. 215 - 247Publisher: Cambridge University PressPrint publication year: 2020