Book contents
- Environmental Impacts from the Development of Unconventional Oil and Gas Reserves
- Environmental Impacts from the Development of Unconventional Oil and Gas Reserves
- Copyright page
- Contents
- Figures
- Tables
- Contributors
- Preface
- Part I Overview
- Part II Environmental Analysis
- Part III Case Studies
- Index
- References
Part II - Environmental Analysis
Published online by Cambridge University Press: 28 July 2022
- Environmental Impacts from the Development of Unconventional Oil and Gas Reserves
- Environmental Impacts from the Development of Unconventional Oil and Gas Reserves
- Copyright page
- Contents
- Figures
- Tables
- Contributors
- Preface
- Part I Overview
- Part II Environmental Analysis
- Part III Case Studies
- Index
- References
Summary
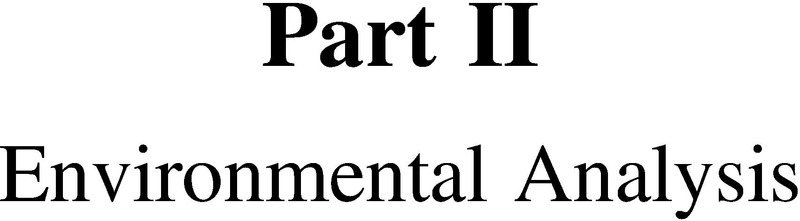
- Type
- Chapter
- Information
- Publisher: Cambridge University PressPrint publication year: 2022