Book contents
- Frontmatter
- Dedication
- Contents
- Acknowledgements
- Preface
- Notation
- 1 Introduction
- 2 Overview
- 3 Theoretical Background
- 4 Periodic Solids and Electron Bands
- 5 Uniform Electron Gas and sp-Bonded Metals
- 6 Density Functional Theory: Foundations
- 7 The Kohn–Sham Auxiliary System
- 8 Functionals for Exchange and Correlation I
- 9 Functionals for Exchange and Correlation II
- 10 Electronic Structure of Atoms
- 11 Pseudopotentials
- Overview of Chapters 12–18
- 19 Quantum Molecular Dynamics (QMD)
- 20 Response Functions: Phonons and Magnons
- 21 Excitation Spectra and Optical Properties
- 22 Surfaces, Interfaces, and Lower-Dimensional Systems
- 23 Wannier Functions
- 24 Polarization, Localization, and Berry Phases
- 25 Topology of the Electronic Structure of a Crystal: Introduction
- 26 Two-Band Models: Berry Phase, Winding, and Topology
- 27 Topological Insulators I: Two Dimensions
- 28 Topological Insulators II: Three Dimensions
- Appendix A Functional Equations
- Appendix B LSDA and GGA Functionals
- Appendix C Adiabatic Approximation
- Appendix D Perturbation Theory, Response Functions, and Green’s Functions
- Appendix E Dielectric Functions and Optical Properties
- Appendix F Coulomb Interactions in Extended Systems
- Appendix G Stress from Electronic Structure
- Appendix H Energy and Stress Densities
- Appendix I Alternative Force Expressions
- Appendix J Scattering and Phase Shifts
- Appendix K Useful Relations and Formulas
- Appendix L Numerical Methods
- Appendix M Iterative Methods in Electronic Structure
- Appendix N Two-Center Matrix Elements: Expressions for Arbitrary Angular Momentum l
- Appendix O Dirac Equation and Spin–Orbit Interaction
- Appendix P Berry Phase, Curvature, and Chern Numbers
- Appendix Q Quantum Hall Effect and Edge Conductivity
- Appendix R Codes for Electronic Structure Calculations for Solids
- References
- Index
- References
References
Published online by Cambridge University Press: 18 September 2020
- Frontmatter
- Dedication
- Contents
- Acknowledgements
- Preface
- Notation
- 1 Introduction
- 2 Overview
- 3 Theoretical Background
- 4 Periodic Solids and Electron Bands
- 5 Uniform Electron Gas and sp-Bonded Metals
- 6 Density Functional Theory: Foundations
- 7 The Kohn–Sham Auxiliary System
- 8 Functionals for Exchange and Correlation I
- 9 Functionals for Exchange and Correlation II
- 10 Electronic Structure of Atoms
- 11 Pseudopotentials
- Overview of Chapters 12–18
- 19 Quantum Molecular Dynamics (QMD)
- 20 Response Functions: Phonons and Magnons
- 21 Excitation Spectra and Optical Properties
- 22 Surfaces, Interfaces, and Lower-Dimensional Systems
- 23 Wannier Functions
- 24 Polarization, Localization, and Berry Phases
- 25 Topology of the Electronic Structure of a Crystal: Introduction
- 26 Two-Band Models: Berry Phase, Winding, and Topology
- 27 Topological Insulators I: Two Dimensions
- 28 Topological Insulators II: Three Dimensions
- Appendix A Functional Equations
- Appendix B LSDA and GGA Functionals
- Appendix C Adiabatic Approximation
- Appendix D Perturbation Theory, Response Functions, and Green’s Functions
- Appendix E Dielectric Functions and Optical Properties
- Appendix F Coulomb Interactions in Extended Systems
- Appendix G Stress from Electronic Structure
- Appendix H Energy and Stress Densities
- Appendix I Alternative Force Expressions
- Appendix J Scattering and Phase Shifts
- Appendix K Useful Relations and Formulas
- Appendix L Numerical Methods
- Appendix M Iterative Methods in Electronic Structure
- Appendix N Two-Center Matrix Elements: Expressions for Arbitrary Angular Momentum l
- Appendix O Dirac Equation and Spin–Orbit Interaction
- Appendix P Berry Phase, Curvature, and Chern Numbers
- Appendix Q Quantum Hall Effect and Edge Conductivity
- Appendix R Codes for Electronic Structure Calculations for Solids
- References
- Index
- References
Summary
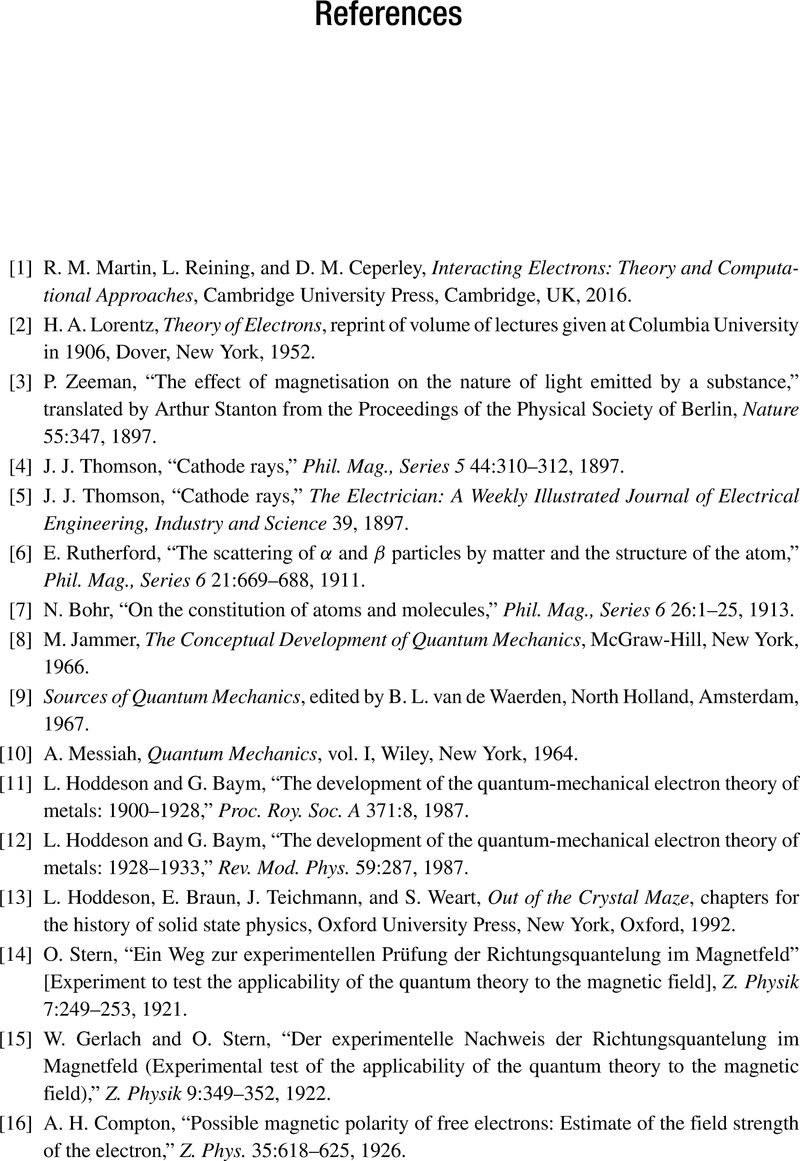
- Type
- Chapter
- Information
- Electronic StructureBasic Theory and Practical Methods, pp. 704 - 755Publisher: Cambridge University PressPrint publication year: 2020