Book contents
- Frontmatter
- Contents
- Preface
- Contributors
- 1 Introduction to the Schwann cell
- 2 Early events in Schwann cell development
- 3 The molecular organisation of myelinating Schwann cells
- 4 The role of the extracellular matrix in Schwann cell development and myelination
- 5 The biology of perisynaptic (terminal) Schwann cells
- 6 Cytokine and chemokine interactions with Schwann cells: the neuroimmunology of Schwann cells
- 7 Schwann cells as immunomodulatory cells
- 8 Mutations in Schwann cell genes causing inherited neuropathies
- 9 Guillain–Barré syndrome and the Schwann cell
- 10 Chronic idiopathic demyelinating polyneuropathy and Schwann cells
- References
- Index
- Plate section
- References
References
Published online by Cambridge University Press: 13 August 2009
- Frontmatter
- Contents
- Preface
- Contributors
- 1 Introduction to the Schwann cell
- 2 Early events in Schwann cell development
- 3 The molecular organisation of myelinating Schwann cells
- 4 The role of the extracellular matrix in Schwann cell development and myelination
- 5 The biology of perisynaptic (terminal) Schwann cells
- 6 Cytokine and chemokine interactions with Schwann cells: the neuroimmunology of Schwann cells
- 7 Schwann cells as immunomodulatory cells
- 8 Mutations in Schwann cell genes causing inherited neuropathies
- 9 Guillain–Barré syndrome and the Schwann cell
- 10 Chronic idiopathic demyelinating polyneuropathy and Schwann cells
- References
- Index
- Plate section
- References
Summary
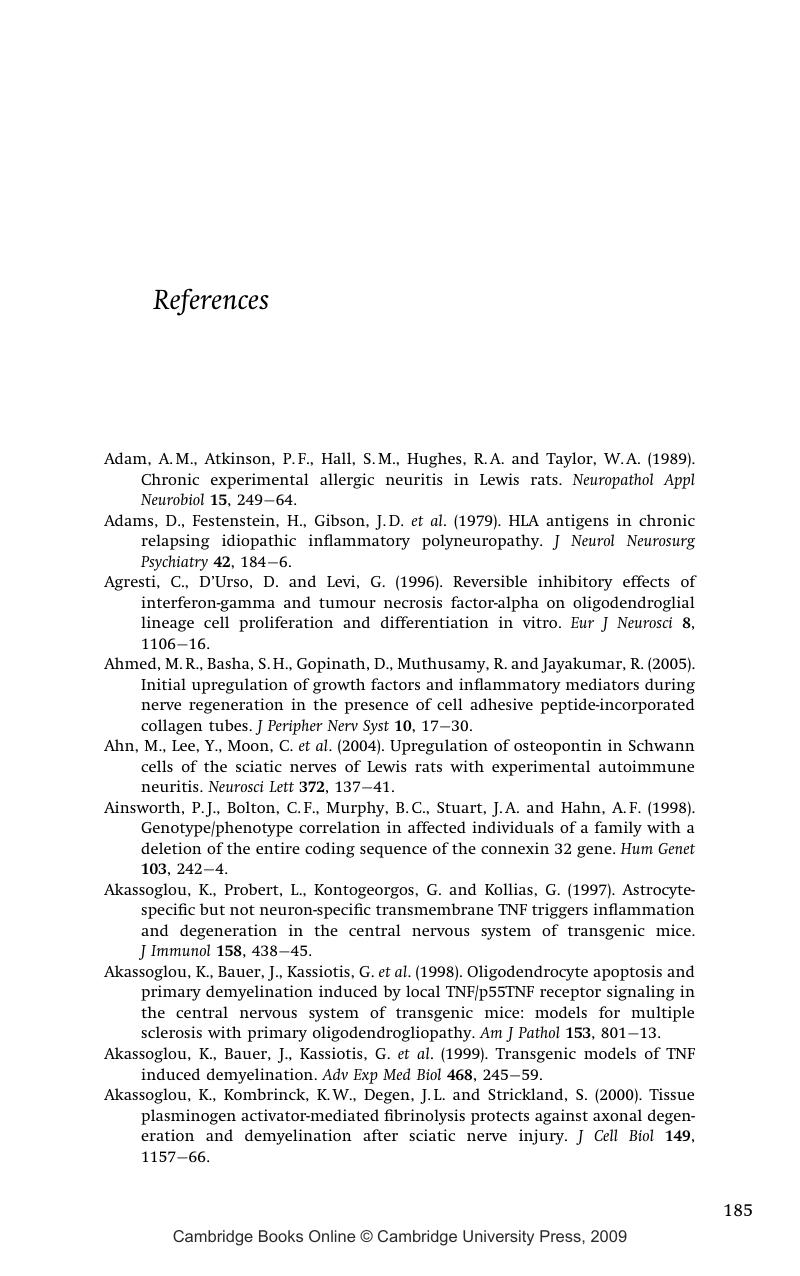
- Type
- Chapter
- Information
- The Biology of Schwann CellsDevelopment, Differentiation and Immunomodulation, pp. 185 - 246Publisher: Cambridge University PressPrint publication year: 2007