Introduction: prostate cancer: prevalence, risk factors and treatments
Prostate cancer is the most common cancer in men, affecting 1·1 million men worldwide in 2012. In the same year, there were 47 300 new cases of diagnosed prostate cancer, and 10 800 deaths from the disease in the UK( 1 ). While there are no clear modifiable risk factors for prostate cancer, family history, diet and lifestyle and ageing have all been linked to its development, with 54 % of men diagnosed in the UK being over 70 years of age. Prostate cancer may be classified as an adenocarcinoma of the prostate gland which can progress from a relatively normal, low-grade prostatic intra-epithelial neoplasm to a high-grade metastatic cancer, with metastases occurring typically in the seminal vesicles, bladder and bones of the pelvis, upper legs and lower spine. The progression of prostate cancer is typically slow, earning it the reputation of being a cancer that men die ‘with’, rather than ‘from’, but there are subtypes of the disease that are aggressive and develop rapidly. Prostate cancer is also associated with a high rate of recovery, with 84 % of men in the UK surviving for 10 years or longer after diagnosis( 2 ).
The growth and function of the prostate is heavily influenced by androgens, and chiefly testosterone, which plays a key role in promoting tumour growth. Hence, effective anti-cancer therapy for locally advanced disease employs systematic approaches to reduce exposure to testosterone by the surgical removal of the testes that produce 90 % of the hormone (orchiectomy), or by the administration of drugs that suppress the production of testosterone (androgen deprivation therapy; ADT). These approaches are used in combination with localised treatments that include brachytherapy or external beam radiotherapy. ADT includes anti-androgen drugs that act at the level of the testes, and gonadotrophin-releasing hormone (GnRH) agonists and antagonists, which suppress the production of testosterone through the hypothalamic–pituitary–gonadal axis. While ADT is highly effective as a systemic treatment as part of combined modality therapy, and has been proven to reduce cancer-specific mortality, its use, and specifically the use of GnRH agonists, has been associated with the co-morbidity of increased risk of CVD( Reference Davis, Rajala and Tyldesley 3 , Reference O’Farrell, Garmo and Holmberg 4 ). This CVD risk is characterised by increased cardiometabolic abnormalities which have a rapid onset( Reference Morote, Gómez-Caamaño and Alvarez-Ossorio 5 ) and underlie an increased prevalence of premature cardiac events such as myocardial infarction, chronic heart failure and stroke in prostate cancer patients within 6 months after ADT has commenced( Reference Jespersen, Nørgaard and Borre 6 – Reference Gandaglia, Sun and Popa 8 ). While clinical recognition of an association between ADT and increased risk of cardiac events comes in the form of contraindications on ADT drug labels, and the United States Food and Drug Administration’s guidelines for the management and targeting of ADT in prostate cancer patients in clinical practice( Reference Levine, D’Amico and Berger 9 ), evidence for a causal relationship between ADT and CVD mortality remains contentious. Reasons for this include confounding of the evidence by the advanced age of patients, cross-cultural differences in response to ADT, and general lack of data from randomised controlled trials (RCT), all of which have increased uncertainty as how best to reverse ADT-related cardiometabolic risk. This review examines the origins and underlying pathophysiology of ADT-related cardiometabolic risk, and asks whether existing definitions of the metabolic syndrome (MetS) under-report cardiometabolic risk for men with prostate cancer, and if this risk could be mitigated through dietary intervention.
Evidence that androgen deprivation therapy increases CVD morbidity and mortality; explanations for the inconsistency between observational studies and randomised controlled trials
Keating et al. ( Reference Keating, O’Malley and Smith 10 ) and others( Reference Tsai, D’Amico and Sadetsky 11 ) were amongst the first to show that ADT, and specifically GnRH agonists, increased risk of incident diabetes and CVD (adjusted hazard ratios 1·44 and 1·16, respectively), and the cardiac events of myocardial infarction and sudden cardiac death (adjusted hazard ratios 1·11 and 1·16, respectively). These findings were soon corroborated by further primary studies, and a highly publicised meta-analysis of observational prospective cohort studies that showed GnRH agonists to be associated, unequivocally, with an increased risk of fatal and non-fatal CVD( Reference Bosco, Bosnyak and Malmberg 12 ). These associations have been linked to the adverse effects of ADT on body composition, insulin sensitivity and other cardiometabolic risk factors described below( Reference Jespersen, Nørgaard and Borre 6 ), and also to the specific effects of GnRH agonists in precipitating cardiac events by promoting vascular dysfunction( Reference Smith, Bennett and Evans 13 – Reference Oka, Utsumi and Endo 15 ) and destabilising atherosclerotic plaque( Reference Knutsson, Hsiung and Celik 16 ). Nevertheless, RCT have generally failed to substantiate a direct, and thus causal, link between ADT/GnRH agonists and cardiac mortality( Reference Roach, Bae and Speight 17 – Reference Voog, Paulus and Shipley 21 ) (Fig. 1). One meta-analysis of RCT identified several possible confounding factors that could have contributed to its negative outcome( Reference Nguyen, Je and Schutz 19 ). These factors included failure to record early cardiac events that occur characteristically within 6 to 24 months after ADT has commenced in a 10-year follow-up period, the misdiagnoses of cardiac death as a clinical endpoint, and the recruitment of men onto RCT who are healthier than their counterparts in observational studies. The latter is of special importance because the incidence of ADT-induced cardiovascular mortality has been shown to be significantly higher in men with pre-existing CVD and/or history of CVD events( Reference Bosco, Bosnyak and Malmberg 12 , Reference Albertsen, Klotz and Tombal 22 – Reference Monzó-Gardiner and Herranz-Amo 24 ). This finding does not exclude men without a previous history of CVD, in which GnRH agonists are also associated with premature cardiac events( Reference Gandaglia, Sun and Popa 25 ), but has led to a consensus of opinion that all future RCT should stratify patients according to cardiac co-morbidity at baseline. It is also noteworthy that observational, prospective cohort studies produce evidence for associations between external factors or treatments and disease outcomes, but not evidence for causality which requires a higher level of evidence, from RCT.
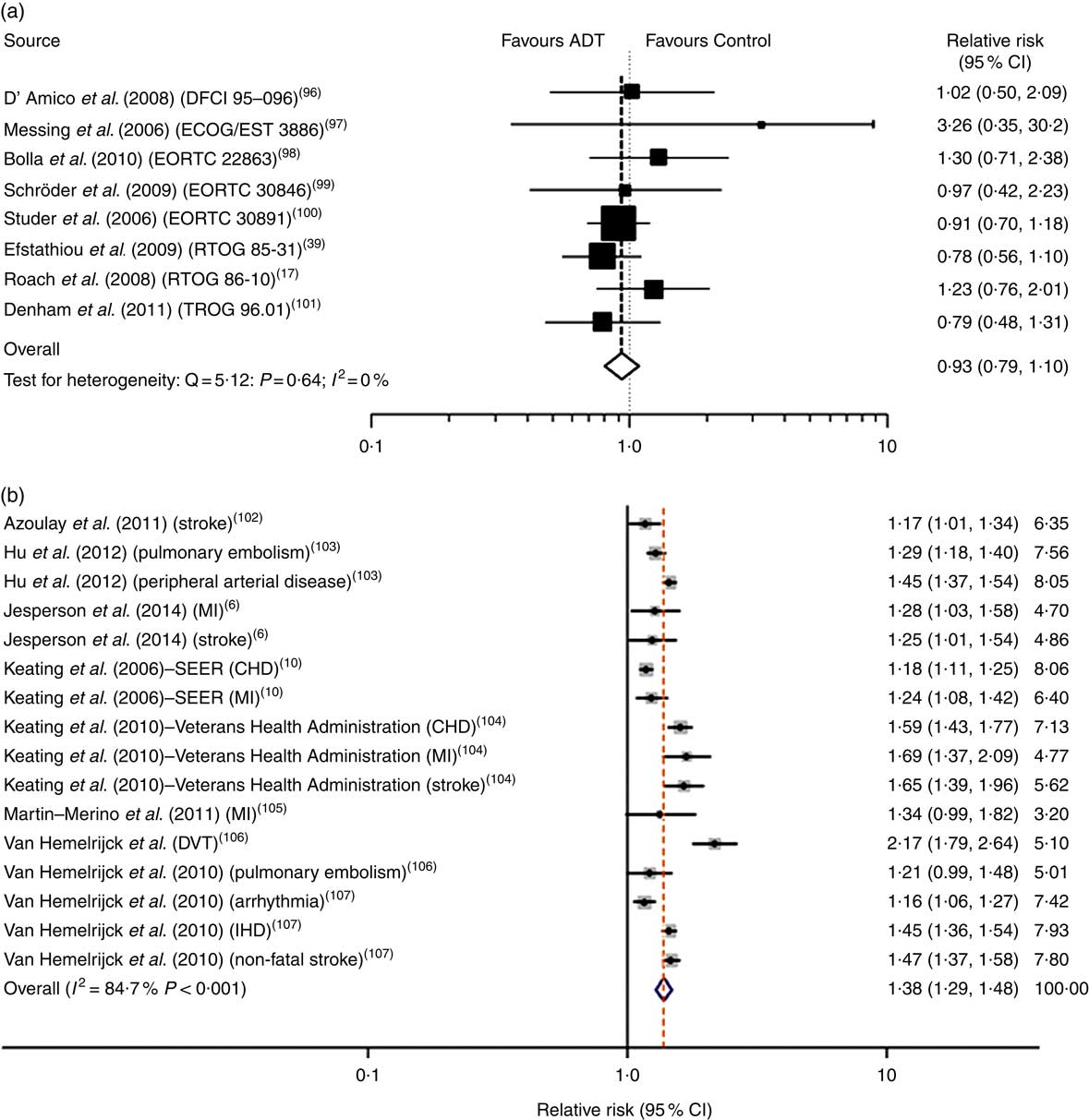
Fig. 1 Androgen deprivation therapy (ADT) and relative risk of CVD mortality in meta-analyses of randomised controlled trials (a) and prospective cohort studies (b). Data taken from Nguyen et al. ( Reference Nguyen, Je and Schutz 19 ) and Bosco et al. ( Reference Bosco, Bosnyak and Malmberg 12 ). Values on the far right in (b) are study weights in %. DFCI, Dana-Farber Cancer Institute; ECOG, Eastern Cooperative Oncology Group; EORTC, European Organisation for Research and Treatment of Cancer; RTOG, Radiation Therapy Oncology Group; TROG, Trans Tasman Radiation Oncology Group; MI, myocardial infarction; SEER, Surveillance, Epidemiology, and End Results; DVT, deep vein thrombosis.
How do the origins and characteristics of androgen deprivation therapy-related cardiometabolic risk differ from classic metabolic syndrome?
Classic metabolic syndrome
Cardiometabolic risk can be defined as a constellation of metabolic risk factors that increase the risk of developing and dying prematurely from CVD( Reference Rutter, Meigs and Sullivan 26 ). These factors provide the defining criteria for the MetS, which originates primarily from an imbalance between energy intake and expenditure that promotes the accumulation and long-term storage of excess body fat in subcutaneous adipose tissue (SAT) and visceral adipose tissue (VAT) and ectopic sites. This increased adiposity also provides an aetiological link between the MetS and many cancers( Reference Lohmann, Goodwin and Chlebowski 27 , Reference Hopkins, Gonclaves and Cantley 28 ), which explains the high prevalence of the MetS in many cancer survivors( Reference de Haas, Oosting and Lefrandt 29 ).
The various components of the MetS are expressed in a heterogeneous fashion between individuals, and for this reason the syndrome has many definitions, which vary according to the emphasis placed on one or more components( Reference Kahn 30 ). All definitions consist of at least five principal components, three of which should be expressed to qualify as having the MetS. These are central adiposity (increased waist circumference), mild hypertension, hyperglycaemia, dyslipidaemia and insulin resistance. While vascular dysfunction, as marked by a loss of endothelial vasodilation capacity and elasticity or stiffening of larger vessels, is not one of the principal components, it has been described as an integrated marker of cardiometabolic risk and early prerequisite of atherosclerosis that develops under the combined influence of other risk factors. The expression of abnormalities in glucose and lipid metabolism is particularly marked in the postprandial phase. This applies especially to the impaired removal of plasma TAG, the time course of which can take over 6 h after a fat-containing meal( Reference Jackson, Walden and Murray 31 ). This extended time interval overlaps naturally with the frequency of food intake and absorption of dietary fat, placing most healthy individuals in a postprandial state to some degree throughout the day and during sleep.
There are marked ethnic and cultural differences in how the type and distribution of body fat contributes to the development and expression of cardiometabolic risk. The accumulation of deep, VAT is a major prerequisite for the development of cardiometabolic risk and diabetes in white Caucasians and South Asians, and other ethnic groups who adopt and adapt to a Westernised diet and inactive lifestyle( Reference Wahi and Anand 32 ). Possible explanations for why VAT should be so closely linked to cardiometabolic risk are that adipocytes in VAT express greater insulin resistance and higher rates of intracellular lipolysis that elevates serum levels of NEFA that can promote further insulin resistance. VAT is also in close proximity to key metabolic organs like the liver and pancreas, and produces adipokines that confer a pro-inflammatory phenotype on this type of adipose tissue( Reference Ouchi, Parker and Lugus 33 ). In contrast, the anatomically more superficial SAT is believed to have a role in acting as a reservoir or ‘fat sink’ to protect peripheral tissues and the viscera from being overloaded with fat( Reference Sattar and Gill 34 ). As such, the variable capacity of SAT to either retain or release fat may be a major determinant of preventing or promoting the accumulation of visceral and ectopic fat, respectively. Interestingly, these determinants of classic MetS may not be the same progenitors of ADT-related cardiometabolic risk.
Androgen deprivation therapy-related cardiometabolic risk
ADT-related cardiometabolic risk shares several of the components of classic MetS – insulin resistance, dyslipidaemia, mild hypertension and vascular dysfunction( Reference Smith, Bennett and Evans 13 , Reference Nguyen, Jarolim and Basaria 35 ) – but is also distinct from the latter as it develops more rapidly from a single metabolic cause, which is the deficiency or relative absence of testosterone. The pathophysiological effects of testosterone deficiency are of fundamental importance to the unique expression of ADT-induced cardiometabolic risk. At a molecular level, testosterone deficiency alters the pattern of cell differentiation, so that pluripotent stem cells develop into subcutaneous adipocytes rather than skeletal muscle myocytes( Reference Grossmann and Wittert 36 ). This effect underlies the earliest adverse effect of ADT on body composition, which is characterised by a pronounced loss of skeletal muscle (sarcopenia)( Reference Smith, Saad and Egerdie 37 ) and accumulation of body fat. The latter is marked by the specific accumulation of SAT within 3 months of commencing ADT, as distinct from VAT in classic MetS( Reference Saylor and Smith 38 ) (Fig. 2). This has been followed within 6–12 months after commencing ADT in cohort studies by the development of cardiometabolic risk factors and significant increase in the onset of premature cardiac events (for example, myocardial infarction, stroke) in some( Reference Jespersen, Nørgaard and Borre 6 – Reference Gandaglia, Sun and Popa 8 ), but not other studies( Reference Roach, Bae and Speight 17 , Reference Alibhai, Duong-Hua and Sutradhar 18 , Reference Voog, Paulus and Shipley 21 , Reference Efstathiou, Bae and Shipley 39 , Reference Punnen, Cooperberg and Sadetsky 40 ). One notable feature of ADT that differs from classic MetS is the preservation or even elevation of serum HDL. However, the high incidence of premature cardiac events on ADT suggests that any cardioprotective effects of HDL may be offset by the adverse effects of ADT on other CVD risk factors. Evidence for the effects of ADT on vascular function in humans has been mixed, with reports of increased arterial stiffness and loss of endothelial cell function in some studies( Reference Smith, Bennett and Evans 13 – Reference Oka, Utsumi and Endo 15 ), but not in others( Reference Nguyen, Jarolim and Basaria 35 , Reference Wall, Galvão and Fatehee 41 ). There is evidence, exclusively from animal models, that acute ADT promotes inflammation, atherosclerosis and destabilises plaque( Reference Knutsson, Hsiung and Celik 16 ), and that this relates specifically to GnRH agonists and not antagonists. A comparison between the components of classic MetS and ADT-induced cardiometabolic risk factors is shown in Table 1.
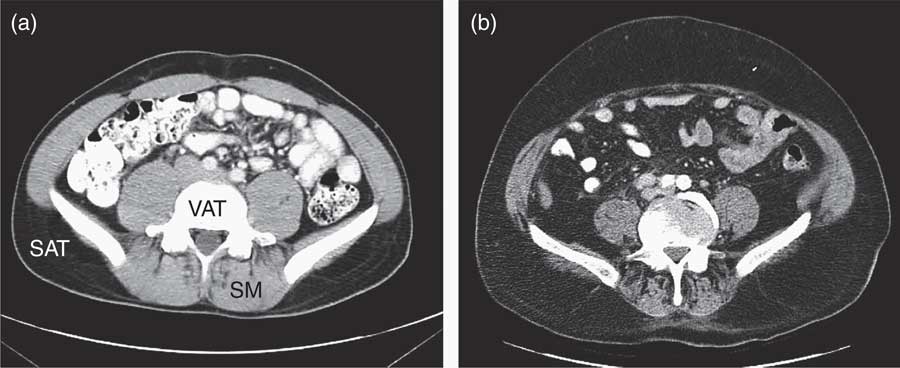
Fig. 2 Abdominal MRI before (a) and after (b) androgen deprivation therapy (ADT)( Reference Saylor and Smith 38 ), showing the accumulation of subcutaneous adipose tissue (SAT), and relative depletion of visceral adipose tissue (VAT) and loss of skeletal muscle (SM) after ADT.
Table 1 Physical and biochemical characteristics associated with classic metabolic syndrome and androgen deprivation therapy (ADT)-related cardiometabolic risk
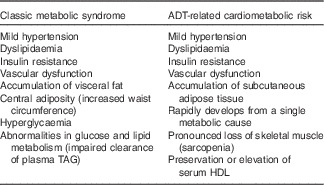
Relevance of sarcopenia and sarcopenic obesity to cardiometabolic risk
The age-related decline in skeletal muscle mass and function is defined as sarcopenia, which when superimposed on obesity gives rise to sarcopenic obesity. Sarcopenia and sarcopenic obesity have both been related to insulin resistance and dysglycaemia( Reference Srikanthan, Hevener and Karlamangla 42 ), and more recently to a higher risk of the MetS in a Korean cohort( Reference Lee, Hong and Shin 43 ), and are common findings in response to ADT( Reference Cheung, Zajac and Grossmann 44 ). From a mechanistic standpoint, insulin resistance is believed to produce a metabolic milieu that imbalances the anabolism and catabolism of skeletal muscle protein and leads to sarcopenia. The subsequent loss of skeletal muscle then reduces the capacity to store glucose as glycogen, and to a lesser extent TAG, but also to a decline in the metabolic capacity to oxidise glucose and fatty acids that will ultimately promote fat storage. These metabolic events have the potential to fuel a vicious cycle that increases cardiometabolic risk in sarcopenic obesity to a greater extent than can be found in either condition alone. Moreover, ageing will also contribute to the risk of developing sarcopenia and cardiometabolic risk through the natural decline of testosterone production, as was evident in a prospective study from the expression of hyperglycaemia and type 2 diabetes at 10, 20 and 30 years before the development of sarcopenia( Reference Ma, Hwang and McMahon 45 ).
Importance of ectopic fat and partitioning of fat between subcutaneous adipose tissue and visceral adipose tissue
When alcohol can be excluded as a cause of fat accumulation in the liver, and liver fat exceeds 5 % by weight, this condition is known as non-alcoholic fatty liver disease (NAFLD). Fatty liver disease (hepatic steatosis) was originally believed to be clinically benign, but has emerged more recently as a critical determinant of cardiometabolic risk, and prerequisite for the development of non-alcoholic steatohepatitis (NASH), a more serious inflammatory condition which can progress to end-stage liver diseases( Reference Loomba and Sanyal 46 ). NAFLD has been associated with all of the components of classic MetS. It has been shown to be responsive to diet and lifestyle modification( Reference Kotronen and Yki-Järvinen 47 ), but its expression in ADT-related cardiometabolic risk is unknown. While the overflow of stored fat from SAT (‘fat-sink’ theory) provides one possible origin of fat for VAT and ectopic sites such as the liver, the extent to which fatty acids from the expanded SAT depot in ADT may promote fat deposition in the liver and NAFLD is equally unknown. It is possible that the extent of fat accumulation in the liver could contribute to the variability in the severity of ADT-related cardiometabolic risk expression between different individuals (Fig. 3).
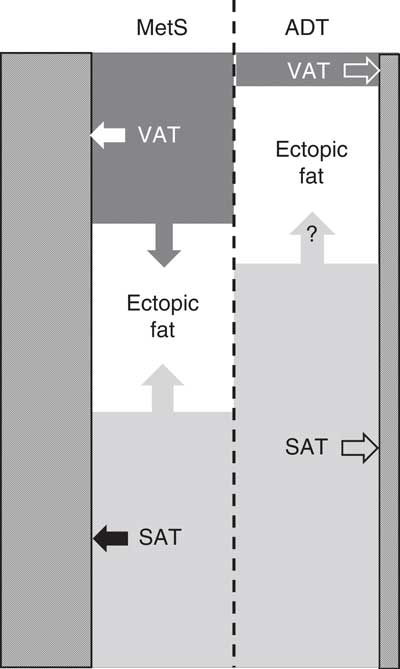
Fig. 3 Relative proportions of stored body fat in visceral adipose tissue (VAT) and subcutaneous adipose tissue (SAT), and potential partitioning (arrows) of stored body fat between VAT, SAT, ectopic sites (for example, liver) and skeletal muscle (///) in classic metabolic syndrome (MetS) and androgen deprivation therapy (ADT).
Does the metabolic syndrome have clinical utility in the identification and management of androgen deprivation therapy-related cardiometabolic risk?
CVD risk is known to increase with the number of components of the MetS( Reference Rutter, Meigs and Sullivan 26 ), but it has been argued that classic MetS has no diagnostic or prognostic value, since its individual components are equally or more effective in predicting CVD risk than combinations of these components( Reference Preiss and Sattar 48 ). Individual cardiometabolic risk factors are also treated clinically in isolation of one another by drug therapies. Clear differences between ADT and classic MetS suggest that the latter may have even less clinical utility in the identification and management of ADT-related cardiometabolic risk. Nevertheless, cardiometabolic risk factors represent sensitive and specific biomarkers of ectopic fat deposition( Reference Kelishadi, Cook and Adibi 49 ), which is an important aetiological factor for the development of CVD, diabetes and chronic liver disease. Also, if cardiometabolic risk can be identified at an early, subclinical stage, this will increase its amenability and responsiveness to diet and lifestyle modification than at a later stage, when long-term drug therapies must be invoked.
By comparison with the lifestyle interventions of weight loss and increased physical activity that are advocated after prostate cancer treatment in cancer survivors, we have relatively little evidence to support the clinical efficacy of dietary interventions.
Search strategy and selection criteria for review
A systematic search of published literature was conducted to retrieve studies that reported on nutrition interventions (according to WHO definition) or detailed dietary intake after the diagnosis of prostate cancer, as well as reported cardiometabolic risk factors and/or sarcopenia. No restriction was placed on study design or duration of follow-up. Only studies reported in English were included from the following electronic databases: the Cochrane Library, PubMed, MEDLINE, EMBASE and CINAHL. The Index/MeSH (Medical Subject Heading) ‘prostate cancer’ was combined with terms associated with nutrition interventions and cardiometabolic risk factors (metabolic syndrome, body fat distribution, body mass index, abdominal obesity, morbid obesity, waist circumference, skinfold thickness, waist-hip ratio, hypertension, dyslipidaemia, insulin resistance, hyperglycaemia, blood pressure, cholesterol, diabetes, dysglycaemia, sarcopenia). Additional search strings were constructed using key word terms. All searches were conducted between February and March 2016 and retrieved 1593 published articles since 1 January 2000.
Future strategies for the dietary treatment of androgen deprivation therapy-induced cardiometabolic risk
Despite similarities between cardiometabolic risk in classic MetS and that induced by ADT, the rapid onset, and distinct and highly variable expression of characteristics in the latter, necessitates a unique and personalised dietary approach. This will require application of the evidence-based effects of diet on ADT-related cardiometabolic risk. However, diet and lifestyle strategies to treat metabolic complications of ADT have predominantly focused on the role of exercise in preference to diet. When the role of diet has been addressed, the quality and detail of information on the interventions have ranged from general healthy eating advice, to more prescriptive guidelines based on the dietary modification of cardiovascular risk, using for example the Adult Treatment Panel III (ATP-III) dietary guidelines( Reference Conti 50 ). The outcome of dietary interventions reported to date has often been poorly described and multi-modal, making it difficult to compare and assimilate results for the design of future dietary interventions (Table 2). In the absence of such data, it would seem prudent to adopt an approach based on the existing evidence for the efficacy of diet on cardiometabolic risk factors in classic MetS. This approach could then be coupled with the concept of personalised nutrition, which is based on the premise that greater improvements in cardiometabolic health can be achieved by the tailoring of dietary modifications to the cardiometabolic risk profile of an individual or, more realistically, group of individuals. In this respect, the co-existence of classic obesity or metabolic obesity, with or without sarcopenia, represent key phenotypic differences for the formulation of an individualised dietary prescription to manage and reduce ADT-related cardiometabolic risk. This approach would provide a more consistent, and evidence-based strategy than many current practices.
Table 2 Dietary intervention studies of androgen deprivation therapy (ADT)-related cardiometabolic risk
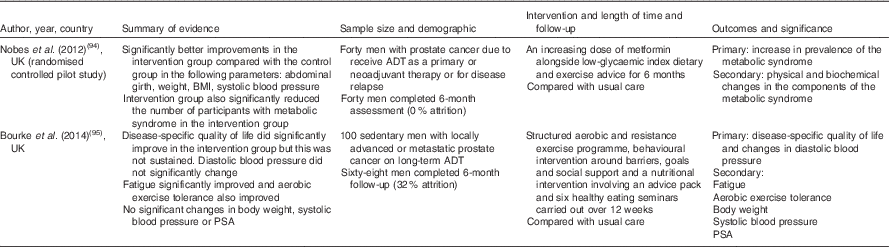
PSA, prostate-specific antigen.
Current evidence for the nutritional management of cardiometabolic risk factors
It is important to emphasise that the nutritional management of cardiometabolic risk does not strictly include the treatment of raised serum LDL-cholesterol. While the latter is an established risk factor for CVD that is associated with increased body weight, obesity and ADT, and is often classified as a cardiometabolic risk factor, its metabolic origins are distinct from the classic cardiometabolic risk factors described above. With the exception of the shared benefits of weight loss, the nutritional management of elevated serum LDL-cholesterol is also distinct from the management of cardiometabolic risk, and delivered via application of the well-documented Portfolio Diet( Reference Jenkins, Kendall and Faulkner 51 , Reference Jenkins, Jones and Lamarche 52 ), which includes weight loss, if appropriate, the replacement of SFA, plant sterols, soya protein, viscous fibres and nuts alongside regular clinic visits. This combined dietary approach has been shown to lower LDL-cholesterol more effectively than a low-saturated fat diet alone( Reference Jenkins, Jones and Lamarche 52 ).
In general, diets that restrict the intake of food energy to achieve weight loss have proven efficacy in improving cardiometabolic risk factors in overweight and obese individuals, primarily by reducing total body fat( Reference Muzio, Mondazzi and Harris 53 , Reference Laddu, Dow and Hingle 54 ). Because body composition is difficult to assess without high-resolution imaging techniques, anthropometric measures of BMI, waist circumference and waist:hip ratio are often used as proxies. Prescriptive targets for weight reduction include a sufficient weight loss to achieve a BMI of 25 kg/m2 for the improvement of hypertension( Reference Appel, Brands and Daniels 55 ), and a BMI of between 21 and 23 kg/m2 for reducing prostate cancer aggression( Reference Arab, Su and Steck 56 ) and an energy restriction of 600 kcal (2500 kJ) per d for the management of obesity( 57 ). Energy restriction can be achieved by the manipulation of portion size and/or energy density of food, and by altering behaviour in ways that suppress appetite, hunger and promote satiety, as for example energy loading early in the day( Reference Garaulet, Gómez-Abellán and Alburquerque-Béjar 58 ), maintaining an adequate quantity and quality of sleep( Reference Knutson 59 ), by fasting( Reference Harrington, Martin and Ravussin 60 ) and through meal replacement( Reference Abete, Astrup and Martínez 61 ). Humans have adapted to a pattern of dietary intake in developed societies that provides ready and regular access to high-energy-dense foods, which over time will inevitably contribute to weight gain and obesity. This pattern of food intake is in complete discord with our innate physiology that is designed to withstand prolonged periods of fasting in an environment where food is frequently unavailable( Reference Mattson, Allison and Fontana 62 ). The unavoidable imposition of time-restricted feeding or intermittent fasting in an environment where food is not readily available, but weight gain and obesity are rare, provides persuasive evidence for the potential of fasting to improve cardiometabolic health. While there is, as yet, no convincing evidence to show that time-restricted feeding or intermittent fasting is more effective than constant energy restriction in promoting weight loss, there are data to suggest the former may confer additional benefits in terms of reducing cardiometabolic risk factors, above and beyond its impact on weight loss( Reference Varady 63 , Reference Harvie, Pegington and Mattson 64 ). In a randomised trial, the timing of food intake was shown to make an impact upon cardiometabolic risk factors in young women, in whom insulin resistance and fasting insulin were significantly better in those who undertook an intermittent energy-restricted dietary pattern, compared with those who continually restricted dietary intake( Reference Harvie, Pegington and Mattson 64 ). Moreover, various fasting practices have been associated with increased dietary compliance, presumably as a favoured option for those unwilling to restrict food intake on a daily basis( Reference Klempel, Kroeger and Bhutani 65 ).
For individuals who express increased cardiometabolic risk, but who are neither overweight nor obese, a condition often referred to as ‘metabolic obesity’, restricting food energy would be inappropriate and unethical. There is convincing evidence to suggest that the origin of cardiometabolic risk in metabolic and classic obesity arises from increased VAT and excess ectopic fat in key metabolic tissues, such as the liver, pancreas, heart and skeletal muscle( Reference Smith 66 ). There are also reports that these depots of VAT and ectopic fat are reducible by energy-restricted weight loss and by manipulating the quality of dietary macronutrients. The latter is addressed in a review by Abete et al. ( Reference Abete, Astrup and Martínez 61 ) that highlights the importance of personalising diets with a specific macronutrient ratio (30 % protein, 40 % low-glycaemic index (GI) carbohydrates, high amounts of MUFA and n-3 fatty acids) as well as fibre, isoflavones, Ca and antioxidants).
Dietary fat
While much of the emphasis of replacing SFA with PUFA and/or MUFA is rightfully focused on reducing serum LDL, the exchange of dietary SFA for PUFA also produces favourable effects in lowering VAT and liver fat, and on vascular function( Reference Rosqvist, Iggman and Kullberg 67 ). In contrast to the established effects of weight loss and physical exercise on increasing insulin sensitivity, there is no definitive evidence to show that isoenergetic changes in diet produce the same effect. While this applies specifically to the replacement of SFA with unsaturated fatty acids( Reference Jebb, Lovegrove and Griffin 68 ), a reduction in VAT following the replacement of SFA with unsaturated fatty acids would be expected to reduce insulin resistance in this tissue. Similarly, although the increased intake of long-chain n-3 fatty acids in supplemental doses (1–3 g/d) from purified marine sources has no impact on insulin sensitivity, these fatty acids exert favourable effects on cardiometabolic risk by reducing serum TAG in the postprandial and post-absorptive phases, improving vascular function, and lowering biomarkers of low-grade inflammation and blood pressure( Reference Jump, Depner and Tripathy 69 ). The cell membranes of hepatocytes taken from patients with NAFLD have also been shown to be relatively deficient in long-chain n-3 PUFA and, conversely, rich in SFA( Reference Araya, Rodrigo and Videla 70 ). Interestingly, supplements of long-chain n-3 PUFA are effective in reducing liver fat in NAFLD( Reference Scorletti, Bhatia and McCormick 71 ) which may explain, in part, how these fatty acids produce beneficial effects on cardiometabolic risk factors.
Dietary carbohydrate
Three ways in which dietary carbohydrates can affect cardiometabolic risk factors are via their glycaemic properties, content of free sugars, and indigestible dietary fibre. GI describes the rate and extent at which carbohydrate-rich foods raise serum glucose, and concomitantly insulin, relative to a pure glucose control. High-GI foods have been linked to obesity and increased cardiometabolic risk( Reference Cocate, Pereira and Marins 72 ). Although there have been various attempts to list GI on food products, GI is determined by numerous physiochemical factors that complicate its measurement and interpretation, and have confounded its practical application in human dietetics and nutrition. Free sugars, formally known as non-milk extrinsic sugars, are sugars added to sweeten foods and include sucrose, a disaccharide of the monosaccharides glucose and fructose, and fructose. Free sugars have been shown to make a significant contribution to energy intake in populations, and are thus implicated in the development of obesity( Reference Te Morenga, Mallard and Mann 73 ), though other more recent data indicate that dietary fat over a mean range of intakes makes a greater contribution to body weight( Reference Anderson, Celis-Morales and Mackay 74 ). High intakes of free sugars in excess of 20 % total energy have been shown to increase VAT and ectopic fat in the liver, and produce marked effects on cardiometabolic risk factors( Reference Stanhope, Schwarz and Keim 75 ). Sugar-sweetened beverages have been identified as one of the main perpetrators for the delivery of such high intake of free sugars( 76 ), and thus potentially adverse metabolic effects, most notably in children and adolescents. However, the mean intake of free sugars in adult populations is well below this level of intake, and shows no significant association with the risk of CVD mortality until free sugars reach about 25 % of total energy intake( Reference Yang, Zhang and Gregg 77 ). Soluble and insoluble dietary fibres from vegetable and cereal sources have been shown to exert favourable and pronounced effects on cardiometabolic risk factors( Reference Riccioni, Sblendorio and Gemello 78 ). While the underlying mechanisms for these beneficial effects of dietary fibre are not entirely clear, they may involve influences on the absorption of nutrients and gut microbiota that are independent of changes in body weight, and promotion of weight loss via the displacement of absorbable energy from dietary fat and carbohydrates.
Dietary protein
The impact of dietary protein on cardiometabolic risk may be mediated to a variable extent by its ability to counteract the adverse metabolic effects of sarcopenia, primarily by reducing anabolic resistance in skeletal muscle( Reference Nowson and O’Connell 79 ). Since it is clear that the expression of cardiometabolic risk is enhanced in the presence of sarcopenia, a strategic priority must be to ensure an adequate intake of total protein, and then to consider the quality and time of ingestion of protein in relation to its anabolic effects on skeletal muscle( Reference Mamerow, Mettler and English 80 ).
Salt and alcohol
The adverse consequences of salt and alcohol are largely centred on their effects in raising blood pressure. Accepted recommendations for the prevention and treatment of hypertension are to lower salt intake to achieve 1·5 g Na/d and moderation of alcohol intake (two units/d for men; one unit/d for women)( Reference Appel, Brands and Daniels 55 ).
Dietary patterns
There is emerging evidence that the most effective dietary strategies for reducing risk and mortality from multifactorial CVD are based on the modification of multiple dietary components within a dietary pattern. Examples of such dietary patterns include variants of the Dietary Approaches to Stop Hypertension (DASH) diet( Reference Moore, Conlin and Ard 81 , Reference Blumenthal, Babyak and Hinderliter 82 ) (rich in fruits and vegetables (eight to ten servings/d), rich in low-fat dairy products (two to three servings/d), and a reduction in saturated fat and cholesterol and K intake (120 mmol/d)), and variants of the Mediterranean diet, as tested in the PREDIMED (Prevención con Dieta Mediterránea) study( Reference Estruch, Ros and Salas-Salvadó 83 ). While these interventions were not specifically designed to lower cardiometabolic risk, it is reasonable to assume that since cardiometabolic risk has a multifactorial aetiology, it will be more responsive to multiple dietary changes within an altered dietary pattern. Focusing on whole foods and dietary patterns rather than single nutrients also reflects the way in which free-living humans consume nutrients and measure their dietary intake. This approach has been embraced recently by the new dietary guidelines in the Netherlands, which are based entirely on manipulating the intake of whole foods( Reference Kromhout, Spaaij and de Goede 84 ).
The Mediterranean diet has become renowned as a dietary pattern associated with the prevention of CVD, which has been successfully tested in the PREDIMED study. The key principles of this dietary pattern are a largely plant-based diet, featuring fruit, vegetables, whole grains and nuts with moderate amounts of oily fish, olive oil and nuts, and smaller quantities of trans-fatty acids and SFA, red meat and processed and refined foods. The PREDIMED study intervened in men at increased risk of CVD with two variants of the Mediterranean diet supplemented with either extra-virgin olive oil (four tablespoons/d) or mixed nuts (≥3 servings/week) against a control group who were advised to lower their intake of dietary fat. After 4·8 years, the oil- and nut-supplemented diets reduced the risk of cardiovascular events by 30 and 28 %, respectively, relative to the control.
A personalised, phenotypic dietary approach for the treatment of androgen deprivation therapy-related cardiometabolic risk
As previously described (Table 1), patients on ADT express a variable combination of drug-induced cardiometabolic risk factors. These can be subdivided into four risk categories or phenotypes, each of which will require different dietary priorities (Table 3).
Table 3 Cardiometabolic risk factors and dietary priorities in different physical phenotypes resulting from androgen deprivation therapy
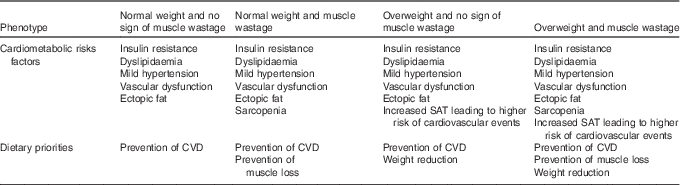
SAT, subcutaneous adipose tissue.
Increased cardiometabolic risk can exist in the absence of a BMI in excess of 30 kg/m2 (metabolic ‘obesity’), but the concept of metabolic ‘healthy’ obesity has recently been challenged as a non-entity because of conflicting evidence for the presence of cardiometabolic risk in this condition( Reference Fan, Song and Chen 85 ). This provides supporting rationale for placing all patients on ADT on a cardioprotective diet, which whilst having no negative effects on health, will be effective in the management of insulin resistance, dyslipidaemia, mild hypertension and vascular dysfunction. Superimposed on this is the priority to promote weight loss in overweight or obese patients, and the need to consider the preservation of muscle mass, if the patients shows signs of sarcopenia. The guidelines described in Table 4 were derived from the compilation of various dietary patterns which have been shown to reduce CVD risk.
Table 4 Dietary guidelines for the treatment of androgen deprivation therapy-related cardiometabolic risk
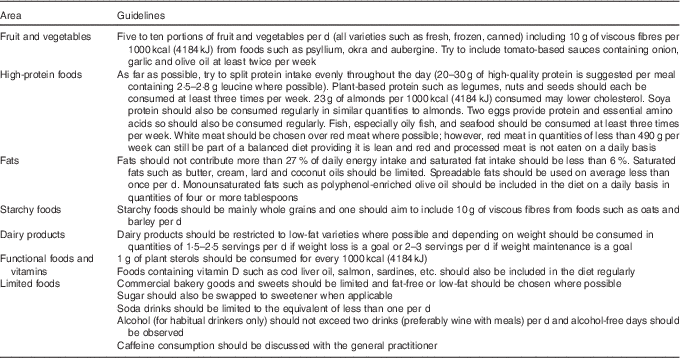
Management of weight and fat distribution
Energy restriction is required to promote weight loss in ADT patients who are overweight or obese (600 kcal (2500 kJ)/d reduction advised by the National Institute for Health and Care Excellence (NICE)). While weight reduction alone appears to be achievable with a daily energy restriction, there is emerging evidence to suggest that additional improvements in insulin resistance and lean mass retention can be achieved through intermittent energy restriction( Reference Varady 63 , Reference Harvie, Pegington and Mattson 64 ).
Sarcopenia
Limited studies have investigated skeletal muscle mass and metabolism in patients on ADT. It has been suggested that promyogenic drugs and myokines may be required to reduce the risk of sarcopenia and frailty in patients on ADT( Reference Basaria and Bhasin 86 ). There is, as yet, no convincing evidence to support the efficacy of lifestyle interventions (nutrition and exercise interventions) as a means for attenuating muscle loss in patients on ADT. It is therefore reasonable to suggest that patients on ADT who show signs of muscle weakness follow similar therapeutic strategies to those who are at risk of muscle wastage, but not on ADT, such as regular protein intake and consumption of specific amino acids.
Weight-loss programmes tend to promote a loss of both fat mass and fat-free mass. A study of 275 men and women in the USA concluded that men decreasing their body weight by 10 % should expect 35–40 % of the weight loss to be fat-free mass( Reference Dixon, Lambert and Grima 87 ). To prevent this loss of muscle in overweight and obese patients with sarcopenia, many studies have examined how weight-loss programmes can be adapted to preserve lean tissue. Increasing protein intake to above 0·9 g/kg per d was shown to be ineffective in preserving muscle mass in obese adults( Reference Backx, Tieland and Borgonjen-van den Berg 88 ). In contrast, a reduction in energy intake of 500 kcal (2100 kJ)/d with the consumption of whey-protein supplement (20 g protein/d) was shown to promote a significant loss of fat mass whilst preserving fat-free mass, as compared with a control group in obese adults( Reference Frestedt, Zenk and Kuskowski 89 ). The synthesis of myofibrillar protein was also shown to be stimulated to a significantly greater extent in a weight-reducing diet that was supplemented with isolated whey protein (1·3±0·1 g protein/kg per d) as compared with soya or carbohydrate( Reference Hector, Marcotte and Churchward-Venne 90 ). Moreover, obese patients following a 600 kcal (2500 kJ)/d energy reduction, resistance training programme and consuming a supplement containing whey protein, leucine and vitamin D containing 21 g protein (equating to an overall protein intake of 1·11±0·28 g protein/kg per d) preserved appendicular muscle mass in comparison with a control group who did not consume a protein supplement( Reference Verreijen, Verlaan and Engberink 91 ).
There is evidence to suggest that despite the similar effects of daily and intermittent energy restriction on weight loss, a higher percentage of lean mass may be preserved when undertaking intermittent energy restriction in the form of ‘feeding’ and ‘fasting’ days( Reference Varady 63 ).
The timing of daily protein intake may also be important in this respect. The 24 h mixed muscle protein fractional synthesis rate was shown to be significantly higher when protein was evenly distributed throughout the day, as compared with skewing intake towards the evening meal( Reference Mamerow, Mettler and English 80 ). A list of putative dietary factors with potential to prevent sarcopenia that results from advancing age includes protein, vitamin D, antioxidants, long-chain PUFA and dietary patterns( Reference Robinson, Cooper and Aihie Sayer 92 ).
Psychological and clinical considerations
As with any long-term diet and lifestyle modification, the ability for an individual to sustain change relies partly on the conscious and subconscious psychological strategies employed throughout the intervention. Michie et al. ( Reference Michie, Ashford and Sniehotta 93 ) have generated a framework of psychological techniques, used within the realms of diet and exercise regimens, and various behaviour change techniques to help individuals modify their behaviours. The latter includes, but is not limited to, goal setting, action planning, barrier identification and problem solving. Lifestyle interventions should not disregard the importance of considering behaviour change techniques which can greatly improve compliance and make an impact on outcomes. Abete et al. ( Reference Abete, Astrup and Martínez 61 ) also highlight the relevance of investigating the intrinsic and extrinsic determining factors of dietary intake to aid compliance in promoting weight loss.
Dietitians and nutrition professionals often receive referrals for patients with cancer who require nutrition support for weight loss as a result of various cancer treatments. Men who are struggling with weight gain and increased cardiovascular risk may be overlooked because weight gain during cancer is perhaps not expected and not monitored in these patients. The holistic clinical care of a patient’s health can also be diminished when individual elements of their condition are managed by different individuals. There is a need to highlight these issues in the service currently provided to patients with prostate cancer, and to generate guidelines that include referral criteria for various healthcare professionals who can advise on lifestyle changes that could reduce risk of CVD.
Summary and conclusions
ADT is highly effective in reducing mortality from prostate cancer, but certain forms of this therapy, most notably GnRH agonists, induce cardiometabolic abnormalities that can lead to premature cardiac events. While ADT-induced cardiometabolic risk is similar to that found in classic MetS, it is distinct in having a single underlying cause of testosterone deficiency, a more rapid onset, and being characterised by an accumulation of SAT, and sarcopenia and sarcopenic obesity.
Cardiometabolic risk can be reduced through energy restriction to promote weight loss, and by manipulating the composition of macronutrients, chiefly fat and carbohydrate, to target specific metabolic risk factors. Existing evidence for the beneficial role of diet in the prevention of ADT-related cardiometabolic risk is scant. Future treatment strategies should consider adopting an evidence-based approach with a personalised strategy that tailors dietary modification to the risk phenotype of the patient. Nutritional assessment should include consideration of the level to which a patient adheres to the Mediterranean diet, the quantity, quality and timing of protein intake, overall energy content and GI, while ensuring that World Cancer Research Fund guidelines are being met. We await the outcome of adequately powered RCT to establish if these dietary modifications will be successful in ameliorating ADT-related cardiometabolic risk.
Acknowledgements
Financial support was received from Movember and Prostate Cancer UK.
B. A. G., L. T., K. P. and S. F. planned, designed and performed literature searches for this review. B. A. G. and L. T. were the principal writers, and K. P. and S. F. provided peer review and editorial comments.
There are no conflicts of interest.