INTRODUCTION
Obsessive compulsive disorder (OCD) has an estimated lifetime prevalence of 1% to 3% (Crino et al., Reference Crino, Slade and Andrews2005) and is characterized by recurrent, intrusive, and bothersome obsessions and compulsions to counteract these thoughts. OCD is among the leading causes of functional disability worldwide (Murray & Lopez, Reference Murray and Lopez1997) with significant deficits in quality of life, which in severe cases are comparable to those observed in schizophrenia (Bystritsky et al., Reference Bystritsky, Liberman, Hwang, Wallace, Vapnik, Maindment and Saxena2001).
An abnormality involving cortical-striatal circuitry has been a critical component of neurobiological models of OCD (Aouizerate et al., Reference Aouizerate, Guehl, Cuny, Rougier, Bioulac, Tignol and Burbaud2004; Szeszko et al., Reference Szeszko, MacMillan, McMeniman, Chen, Baribault, Head, Lim, Ivey, Rose, Banerjee, Bhandari, Moore and Rosenberg2004). Moreover, defects in multiple brain regions involved in this neural loop have also been considered integral to OCD phenomenology, cognitive dysfunction (Kwon et al., Reference Kwon, Kim, Lee, Lee, Kim, Lyoo, Cho and Lee2003; for review see Menzies et al., Reference Menzies, Chamberlain, Laird, Thelen, Sahakian and Bullmore2008), and effective serotonergic and/or dopaminergic pharmacotherapy including the basal ganglia, thalamus, and regions of the prefrontal cortex (Rapoport, Reference Rapoport1991). Consistent with disruption of this neural circuitry, significant neurocognitive deficits including impaired attention, learning strategies, memory, set-shifting, and response inhibition have been observed in OCD (Chamberlain et al., Reference Chamberlain, Blackwell, Fineberg, Robbins and Sahakian2005; Kuelz et al., Reference Kuelz, Hohagen and Voderholzer2004). Moreover, recent data suggest that different symptom profiles in patients with OCD have been linked differentially with the motor-activating striato-thalamo-orbitalfrontal system versus the motor-inhibitory orbitofrontal-striatal loop (Cavedini et al., Reference Cavedini, Gorini and Bellodi2006).
Despite a heterogeneous diagnostic phenotype, a number of well-designed neurocognitive studies have demonstrated consistent and replicable cognitive impairment in domains related to striato-thalamo-orbitofrontal systems, when subjects were matched for symptom severity, co-morbidities, and demographic features (Aouizerate et al., Reference Aouizerate, Guehl, Cuny, Rougier, Bioulac, Tignol and Burbaud2004; Cavedini et al., Reference Cavedini, Gorini and Bellodi2006; Greisberg & McKay, Reference Greisberg and McKay2003). Neuroimaging studies have reported abnormalities consistent with the cognitive impairments noted; however, a recent meta-analysis suggested that structural and functional brain changes may be more widespread in OCD than originally believed (Menzies et al., Reference Menzies, Chamberlain, Laird, Thelen, Sahakian and Bullmore2008). The goal of the current study is thus to characterize the degree of global cognitive impairment and the relative strengths and weaknesses of OCD patients using profile analysis across 6 neurocognitive domains versus healthy volunteers.
METHODS AND MATERIALS
Subjects
Included 26 adult outpatients with OCD and 38 healthy volunteers matched for age, sex, and handedness. Diagnoses were made through a diagnostic consensus process in which Structured Clinical Interview for Axis I DSM-IV Disorders (First et al., Reference First, Spitzer, Williams and Gibbon1998) (SCID) interviews were augmented by medical records and presented in a weekly conference comprised of senior researchers and clinical staff. Healthy volunteers were free of Axis I disorders, as determined from the SCID-Non-Patient version and had no Axis I first-degree family history. Procedures were approved by the North Shore–Long Island Jewish Health System IRB and written informed consent was obtained from all participants.
Clinical Assessments
Included the Yale-Brown Obsessive Compulsive Scale (YBOCS) (Goodman et al., Reference Goodman, Price, Rasmussen, Mazure, Fleischmann, Hill, Heninger and Charney1989), the 17-item Hamilton Depression Rating Scale (HDRS) (Hamilton, Reference Hamilton1960) and the Hamilton Anxiety Rating Scale (HARS) (Hamilton, Reference Hamilton1959). Patient medication status was heterogeneous: seven subjects were medication-free; six were on selective serotonin reuptake inhibitor (SSRI) monotherapy; and remaining subjects were taking multiple medications. Concomitant medications included other antidepressants (tricyclics, bupropion) (n = 5); atypical antipsychotics (n = 6); mood stabilizers (n = 3); and modafanil (n = 3). No subject had undergone psychosurgical procedures.
Neurocognitive Assessment
We computed six neurocognitive domains, based on prior empirical work by our group (Bilder et al., Reference Bilder, Goldman, Robinson, Reiter, Bell, Bates, Pappadopulos, Willson, Alvir, Woerner, Geisler, Kane and Lieberman2000; Rhinewine et al., Reference Rhinewine, Lencz, Thaden, Cervellione, Burdick, Henderson, Bhaskar, Keehlisen, Kane, Kohn, Fisch, Bilder and Kumra2005), by computing an average of z-scores from each individual test comprising the respective domain. Scores were computed so that higher values were indicative of better performance. Domains (with coefficient alpha in parentheses) included: (1) Motor (.70) comprising right- and left-handed Finger Tapping and Grooved Pegboard performance; (2) Verbal Memory (.78) comprising California Verbal Learning Test-Trials 1–5 Total and Delayed Free recall; Wechsler Memory Scale-Revised (WMS-R)-Logical Memory I and II; and Wechsler Adult Intelligence Scale–Revised (WAIS-R)-Digit Span; (3) Visual Memory (.85) comprising WMS-R-Visual Reproduction I and II; (4) Reasoning and Problem Solving (.73) comprising Wisconsin Card Sorting Test-Categories completed and Perseverative Responses, Ruff Figural Fluency–Total Designs; and WAIS-R-Block Design; (5) Speed of Processing (.74) comprising WAIS-R-Digit Symbol, Trailmaking Test, A and B, Time to Completion; and Cancellation Test-Total time; and (6) Language (.78) comprising Controlled Oral Word Association Test; Animal Naming; WAIS-R-Vocabulary; and WAIS-R-Information. In addition, a global domain was computed as the average of the 6 neurocognitive domains.
Statistics
Raw scores were transformed to z-scores based on data from the healthy volunteers who were assigned a mean of 0 and a standard deviation of 1. Patients were compared to healthy volunteers on neurocognitive domains using multivariate analysis of covariance (MANCOVA) with age as a covariate (multivariate ANOVA assumptions were met). Effect sizes for group differences were computed using Cohen's d.
Spearman's correlations were used to investigate the relationship between the neurocognitive domains and YBOCS, HDRS, and HARS total scores.
We used profile analysis to contrast each individual neurocognitive domain with a mean global domain score, which was computed by averaging the z-scores of all other domains excluding the one to which it was being compared. This approach specifically addresses the question: Given a subject's performance on all other neurocognitive domains (represented as a global z-score), to what degree does an individual's performance deviate from expectation on any specific neurocognitive domain? All domain z-scores were transformed to standardized residuals using the approach described by Chapman and Chapman (Reference Chapman and Chapman1989). Specifically, residual scores were computed using the healthy volunteers' regression equations with global z-score as the independent variable and the individual domain score as the dependent variable. We then applied these equations to the patient group to obtain standardized residual scores for each domain. The use of standardized residuals is superior to simply using z-scores for profile analysis, as it considers the statistical interdependence of the measures.
Post hoc analyses included: (1) MANCOVA for the individual neurocognitive measures in each of the domains in an effort to delineate patterns of performance within each domain and (2) ANCOVA to test for effects on neurocognition related to medication status. In both of these analyses we covaried for age.
RESULTS
OCD patients were 34.6% women with a mean age of 37.3 ± 9.9 years and an average education of 14.5 ± 1.8 years. Patients were 57.7% right-handed and 88.5% were self-identified as white. Healthy volunteers were 36.8% women with a mean age of 35.0 ± 7.8 years and an average education of 14.6 ± 1.5 years. Healthy volunteers were 76% right-handed and 84.2% were white. Patients did not differ significantly from healthy volunteers in distributions of age, education, sex, handedness or race. OCD patient subtypes were as follows: Symmetry/ordering (n = 2); Hoarding (n = 2); Contamination/cleaning (n = 13); Aggressive/checking (n = 7); and Sexual/religious (n = 2). Clinical ratings in the patient group were consistent with moderate to severe overall illness severity, with approximately equivalent ratings on obsessive and compulsive severity indices. Levels of state anxiety at the time of testing, as per the HARS, were in the mild range and current depressive symptoms as per the HDRS were rated as mild to moderate (Table 1).
Table 1. Neurocognitive domain performance of OCD patients compared to healthy volunteers

1 Z-score relative to healthy control mean of 0.0 with a standard deviation of 1.0.
* p-value ≤ .05;
** p-value ≤ .01.
YBOCS: Yale Brown Obsessive Compulsive Scale; HDRS: Hamilton Depression Rating Scale; HARS: Hamilton Anxiety Rating Scale.
MANCOVA of the six neurocognitive domains and the global z-score revealed a significant main effect of group (F = 5.88; df = 1,63; p < .001) and age (F = 3.42; df = 1,63; p = .006). Patients demonstrated a significant global deficit approximately ½ standard deviation below healthy volunteers on the global z-score (F = 14.13; df = 1,63; p < .001). Post-hoc analyses revealed significant group differences in visual memory, motor functioning and processing speed (Table 1). In contrast, the groups did not differ significantly in verbal memory, reasoning/problem solving, or language (Table 1).
There were no significant correlations between any of the neurocognitive domains and YBOCS or HDRS scores; however, performance in the Motor domain was significantly correlated with HARS total score (Spearman's ρ = .60; p = .002), such that higher anxiety ratings were associated with better performance on motor tasks.
Profile analysis indicated that OCD patients had significant deficits in motor functioning (t = 2.53; df = 25; p = .018) and processing speed (t = 2.14; df = 25; p = .037) compared to their overall neurocognitive performance. In contrast, relative strengths were observed in verbal memory (t = 2.68; df = 25; p = 0.013), language (t = 2.21; df = 25; p = .037) and reasoning/problem solving (t = 2.10; df = 25; p = 0.047) compared to overall functioning (Fig. 1).
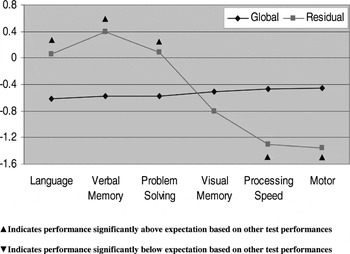
Fig. 1. Neurocognitive profile of OCD patients using standardized residuals.
We also conducted post-hoc, exploratory analyses (MANCOVA) across individual neurocognitive tests in an effort to parse out more specific impairments within each domain of function (Supp. Table 1), as previous literature has demonstrated impairments on specific aspects of neurocognitive tests, whereas other domain-related measures seem to be less impacted by OCD. Within the domains of motor functioning and processing speed, we found that gross motor speed (Finger Tapping Test) was relatively intact, whereas fine motor skills were significantly impaired (Grooved Pegboard). In addition, both components of the Trailmaking Test (A & B) were impaired, as was Letter Cancellation; however, WAIS-R Digit Symbol was not significantly impaired. Verbal Memory and Visual Memory domains both displayed an interesting pattern of impairment on immediate recall of information with relatively intact delayed recall, suggestive of deficits in learning that do not seem to translate into impaired memory performance. No significant impairment was noted on any of the measures in the Reasoning and Problem Solving domain or in the Language domain.
Secondary analyses to assess the effects of medication status revealed that deficits in the motor domain may be explained, at least in part, by medication. Univariate ANCOVA, controlling for age, demonstrated a significant main effect of medication (F = 4.38; df = 1,25; p = 0.048), such that patients taking at least one psychotropic medication (n = 19) were more impaired (z-score = −1.12 ±0.94) than patients who were not taking any psychotropic medications (n = 7) (z-score= −0.29 ±0.62); however this did not seem to be related to medication class. Specifically, patients taking SSRI monotherapy (n = 6) performed comparably (z-score= −1.25) to patients taking atypical antipsychotics (n = 5) (z-score= −1.14). Notably, deficits in the Processing speed domain did not appear to be related to medication status (F = .28; df = 1,25; p = .61) nor did performance in any other neurocognitive domain.
DISCUSSION
Using a comprehensive neurocognitive battery we report significant cognitive impairment in adult patients with OCD. We identified a global deficit of approximately ½ standard deviation and specific deficits in motor and processing speed domains. Moreover, compared to their overall performance, OCD patients demonstrated relative strengths in language, verbal memory, and problem solving. These data are consistent with previous reports of significant deficits on speeded measures in patients with OCD (Chamberlain et al., Reference Chamberlain, Blackwell, Fineberg, Robbins and Sahakian2005).
Of note, our cohort of OCD patients did not display the extent of executive dysfunction and verbal memory impairment that has recently been reported (Chamberlain et al., Reference Chamberlain, Fineberg, Menzies, Blackwell, Bullmore, Robbins and Sahakian2007). These discrepancies may be related to a lack of sensitivity of some of the cognitive measures to the deficits in OCD or to the blurring of multiple cognitive constructs when using a domain approach. Specifically, OCD patients did indeed demonstrate deficits on the immediate recall of both verbal and visual information that did not carry over as impaired delayed recall performance. This is consistent with the notion that dysfunctional strategies for learning may be a neurocognitive feature of OCD that is not always captured by pure memory tests (i.e., delayed recall measures). It is also possible that the use of a profile analysis in this study, which included standardized residuals controlling for the global deficit, resulted in less severe executive functioning impairment by virtue of the fact that a proportion of variance on all individual measures and domains is explained directly by global (or general) cognitive ability. Finally, the lack of impairment on measures of executive function may also be attributable to a higher functional capacity in our cohort of OCD patients relative to those previously studied; however, our cohort did fall within the moderate to severely-ill range on psychopathology ratings.
Our results are at least partially consistent with data derived from neuroimaging studies of OCD. A recent meta-analysis of the imaging findings in OCD patients provided an excellent summary of previous work related to frontal-striatal systems and highlighted the need to consider the possibility that these are not the only brain regions associated with the symptoms or the cognitive impairment related to OCD (Menzies et al., Reference Menzies, Chamberlain, Laird, Thelen, Sahakian and Bullmore2008). Many studies have utilized an ROI approach, inherently limiting the obtainable results to specific regions targeted by the design and further reducing the capacity to identify structural and functional abnormalities within neural systems, as opposed to discrete brain regions. Recent studies using whole brain approaches, including voxel-based morphometry (VBM), or positron emission tomography (PET), combined with multivariate statistical methodology, have proven to be more sensitive to network abnormalities (Harrison et al., Reference Harrison, Yücel, Shaw, Brewer, Nathan, Strother, Olver, Egan, Velakoulis, McGorry and Pantelis2006) and have revealed novel areas of interest, including posterior cingulate, precuneus, and cerebellum (Soriano-Mas et al., Reference Soriano-Mas, Pujol, Alonso, Cardoner, Menchon, Harrison, Deus, Vallejo and Gaser2007). In the future, these approaches may allow for greater explanatory power with regard to cognitive deficits detected in OCD, because they are undoubtedly related to abnormalities in relatively large-scale networks in the brain. This may be particularly true when using standard neuropsychological measures, which were originally devised to assess broader and more complex aspects of cognition (i.e., working memory), as compared with some of the more recently developed experimental measures that are designed to assay more discrete aspects of neurocognition (i.e., alerting or orienting of attention). The inclusion of “purer” measures of cognitive function that are specifically targeted to the phenomenology of OCD will be an important step in future studies.
Psychomotor deficits may reflect an epiphenomenon of the psychopathological symptoms of OCD (i.e., excessive monitoring and checking of response), which undoubtedly influence the rate at which cognitive functions are carried out (Veale et al., Reference Veale, Sahakian, Owen and Marks1996). In contrast, neurocognitive slowness may be a direct result of dysfunctional neural circuitry in the cortical-striatal loop (Purcell et al., Reference Purcell, Maruff, Kyrios and Pantelis1998), as it has been noted to be specific to tasks that tap frontal-subcortical systems (Galderisi et al., Reference Galderisi, Mucci, Catapano, D'Mato and Maj1995) and it correlates with neurophysiologic abnormalities in these brain regions (Kim et al., Reference Kim, Kang, Youn, Kang, Kim and Kwon2003). Unfortunately, because of sample size limitations, we were unable to adequately assess the relationships between clinically-rated features of illness, such as obsessional slowness, and neurocognitive dysfunction, which might begin to address this question.
Our results suggest that motor and cognitive speed are critical to the impairments in OCD and may have implications for future study design. Although analyses revealed that medicated OCD patients, regardless of medication class, performed significantly worse than unmedicated patients on tasks of motor skills, medication status did not seem to significantly influence performance in any other neurocognitive domain. We cannot exclude the possibility that medications may have contributed to motor impairment; however, recent studies have suggested that treatment with SSRIs does not significantly impact motor performance in healthy subjects (Wingen et al., Reference Wingen, Bothmer, Langer and Ramaekers2005) and treatment with atypical antipsychotics may in fact improve performance on tasks of motor and processing speed in schizophrenia patients (Keefe et al., Reference Keefe, Seidman, Christensen, Hamer, Sharma, Sitskoorn, Lewine, Yurgelun-Todd, Gur, Tohen, Tollefson, Sanger and Lieberman2004), making this an unlikely explanation for our results.
There was no significant relationship between neurocognition and either OCD symptom severity or depression, as measured by the YBOCS and the HDRS; however, total HARS score was positively correlated with motor performance, which is likely best explained by an inverted-U phenomenon in which elevated levels of state anxiety may enhance performance on certain types of neurocognitive tests. Moreover, our study was limited by the sample size and possibly by the lack of representation of a severely ill group of OCD patients, such as patients who might require psychosurgery to control their symptoms. We are unable to demonstrate that the cognitive dysfunction noted in our sample is related to psychopathology that is specific to OCD, as general features of OCD such as anxiety are common to other psychiatric diseases, as are abnormalities in frontal-striatal functions. Additional neurocognitive and neuroimaging studies will be necessary to determine how specific deficits are related to the underlying pathophysiology of the disorder and to what extent they might contribute to impairment in other neurocognitive domains and relate to specific symptom domains.
ACKNOWLEDGMENTS
This work was supported in part by grants from the National Institute of Mental Health to Dr. Szeszko (MH01990) and the NSLIJ Research Institute General Clinical Research Center (M01 RR018535).
APPENDIX
Table 1. Neurocognitive performance on individual measures: OCD patients compared to healthy volunteers

1 Z-score relative to healthy control mean of 0.0 with a standard deviation of 1.0. Corresponding T-scores (mean of 50 SD of 10) and Scaled scores (Mean of 10 and SD of 3) are provided for ease of interpretation across standardized scales.
* p-value ≤ .05;
** p-value ≤ .01.