Book contents
- Multiparameter Flow Cytometry in the Diagnosis of Hematologic Malignancies
- Multiparameter Flow Cytometry in the Diagnosis of Hematologic Malignancies
- Copyright page
- Contents
- Contributors
- Preface
- Abbreviations
- 1 Flow Cytometry in Clinical Haematopathology: Basic Principles and Data Analysis of Multiparameter Data Sets
- 2 Antigens
- 3 Flow Cytometry of Normal Blood, Bone Marrow and Lymphatic Tissue
- 4 Reactive Conditions and Other Diseases Where Flow Cytometric Findings May Mimic Haematological Malignancies
- 5 Examples of Immunophenotypic Features in Various Categories of Acute Leukaemia
- 6 Acute Lymphoid Leukaemias (ALL) and Minimal Residual Disease in ALL
- 7 Immunophenotyping of Mature B-Cell Lymphomas
- 8 Plasma Cell Myeloma and Related Disorders
- 9 Mature T-Cell Neoplasms and Natural Killer-Cell Malignancies
- 10 Flow Cytometric Diagnosis of Hodgkin's Lymphoma in Lymph Nodes
- 11 Minimal Residual Disease in Acute Myeloid Leukaemia
- 12 Ambiguous Lineage and Mixed Phenotype Acute Leukaemia
- 13 Flow Cytometry in Myelodysplastic Syndromes
- 14 Future Applications of Flow Cytometry and Related Techniques
- Index
- References
2 - Antigens
Published online by Cambridge University Press: 01 February 2018
- Multiparameter Flow Cytometry in the Diagnosis of Hematologic Malignancies
- Multiparameter Flow Cytometry in the Diagnosis of Hematologic Malignancies
- Copyright page
- Contents
- Contributors
- Preface
- Abbreviations
- 1 Flow Cytometry in Clinical Haematopathology: Basic Principles and Data Analysis of Multiparameter Data Sets
- 2 Antigens
- 3 Flow Cytometry of Normal Blood, Bone Marrow and Lymphatic Tissue
- 4 Reactive Conditions and Other Diseases Where Flow Cytometric Findings May Mimic Haematological Malignancies
- 5 Examples of Immunophenotypic Features in Various Categories of Acute Leukaemia
- 6 Acute Lymphoid Leukaemias (ALL) and Minimal Residual Disease in ALL
- 7 Immunophenotyping of Mature B-Cell Lymphomas
- 8 Plasma Cell Myeloma and Related Disorders
- 9 Mature T-Cell Neoplasms and Natural Killer-Cell Malignancies
- 10 Flow Cytometric Diagnosis of Hodgkin's Lymphoma in Lymph Nodes
- 11 Minimal Residual Disease in Acute Myeloid Leukaemia
- 12 Ambiguous Lineage and Mixed Phenotype Acute Leukaemia
- 13 Flow Cytometry in Myelodysplastic Syndromes
- 14 Future Applications of Flow Cytometry and Related Techniques
- Index
- References
Summary
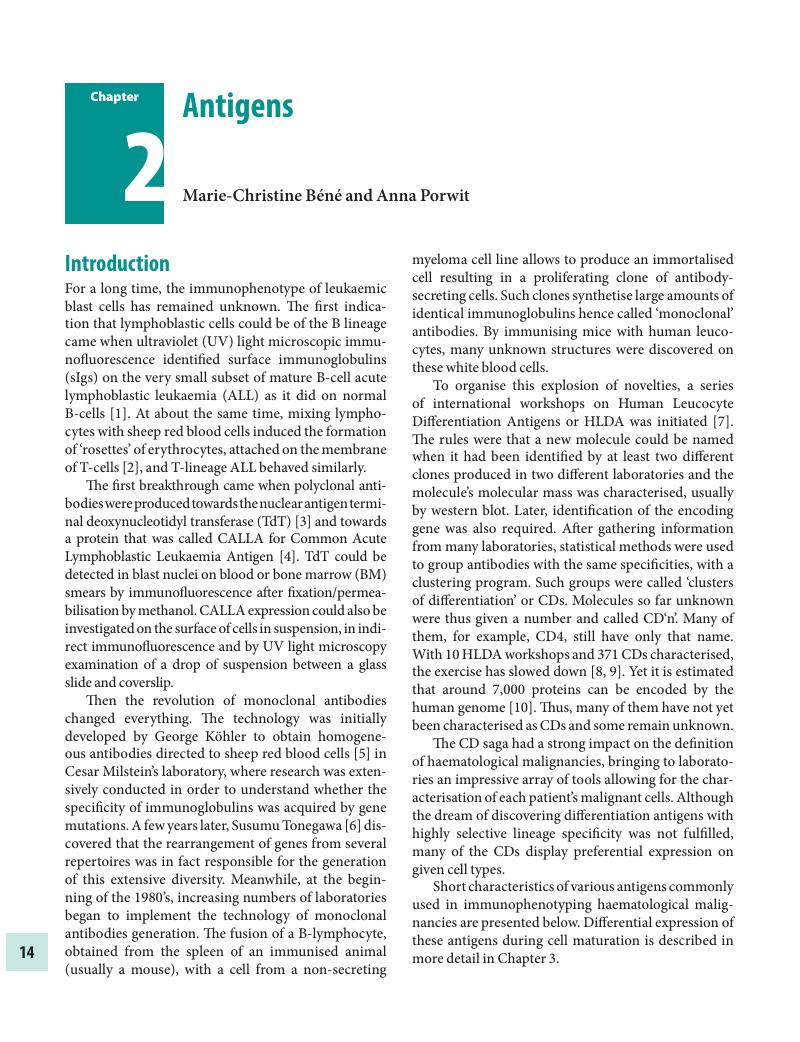
- Type
- Chapter
- Information
- Publisher: Cambridge University PressPrint publication year: 2018