Eradication of aquatic plant species is more difficult than eradicating terrestrial weeds (Genovesi Reference Genovesi2011). The constraints for working in an aquatic environment include physical access, political and public resistance against the use of aquatic pesticides (Hedge et al. Reference Hedge, Kriwoken and Patten2003), herbicide resistance (Puri et al. Reference Puri, MacDonald, Altpeter and Haller2007), difficulties in obtaining treatment efficacy with mechanical or chemical treatment (Patten and O’Casey Reference Patten and O’Casey2010), undesirable non-target impacts, and frequent endangered-species issues (Kerr et al. Reference Kerr, Hogle, Ort and Thornton2016; Strong and Ayres Reference Strong and Ayres2013, Reference Strong and Ayres2016).
Box 1 Management Implications
Large infestations of invasive plant species can cause major ecological perturbation. This is especially true in fragile habitats such as estuaries. With difficult-to-control species, like Spartina alterniflora (smooth cordgrass), herbicides are often the only effective management tool after the invasion has become widespread. The use of herbicides in sensitive habitats, however, is often prevented or delayed by the political climate and regulatory restrictions. This research documents how the widespread estuarine use of the herbicide imazapyr by land managers for over a decade extirpated over 3,000 ha of a recalcitrant invasive species, S. alterniflora. This resulted in almost immediate usage of restored habitat by shorebirds, with only a small percentage of the affected tidelands permanently succeeded to salt marsh species. Extirpation of S. alterniflora from all of the infested area was achieved with concerted effort by land managers. Achieving eradication, however, when the remaining plants are difficult to control and cryptic, will take long-term commitment of major resources.
A large-scale successful effort of aquatic weed control approaching eradication has been achieved with Spartina alterniflora Loisel (smooth cordgrass) in Willapa Bay, WA. Two other very successful eradication efforts are with caulerpa [Caulerpa taxifolia (M. Vahl)] in a small lagoon in California (Anderson Reference Anderson2005) and the forty-year effort to eradicate hydrilla [Hydrilla verticillata (L.F.) Royle] in 20 out of 29 separate waterways in California (Kratville Reference Kratville2013).
Several species of the grass genus Spartina are noted for their invasiveness on intertidal mudflats and saltmarshes due to their propensity for rapid expansion and ecosystem engineering (Ainouche et al. Reference Ainouche, Fortune, Salmon, Parisod, Grandbastien, Fukunaga, Ricou and Misset2009; Strong and Ayres Reference Strong and Ayres2013). The ecological consequences of Spartina invasions have been well studied (Grosholz et al. Reference Grosholz, Levin, Tyler and Neira2009). One species, S. alterniflora, occupied ~3,600 solid ha, covering the ~27,000 ha of intertidal and supratidal habitat of Willapa Bay, WA (WSDA 2017). Because of the serious threat it posed to the multimillion-dollar shellfish industry and to migratory shorebirds along the Pacific Flyway, eradication was legislatively mandated in 1994 (Hedge et al. Reference Hedge, Kriwoken and Patten2003). The effort to eradicate S. alterniflora was conducted by federal, state, and county resource agencies and the shellfish industry, utilizing the herbicide glyphosate and mechanical tilling and crushing (Hedge et al. Reference Hedge, Kriwoken and Patten2003; Patten and O’Casey Reference Patten and O’Casey2010). S. alterniflora expanded unabated despite the millions of dollars per year spent on its control. Progress was first achieved when imazapyr started to be used in 2004 (Patten 2003). Within five years, 3,500 solid ha in 2004 was reduced to 100 solid ha. In subsequent years, there was an ~49% annual decline in solid ha of S. alterniflora. At the end of the 2016 treatment season, 1,388 individual plants were found and treated (<0.4 ha–1) by resource agencies (Washington State Department of Agriculture, personal communication). Over the past three decades, over $30 million has been spent on the control effort in Willapa Bay (Strong and Ayres Reference Strong and Ayres2013). Peak annual expenditures for the control effort were >$2.4 million, decreasing $0.8 million during the past 3 years. This manuscript tracks (1) the success of the control (and extrapolates the duration that will likely be needed to achieve eradication), (2) post-control succession of affected tidelands to native marsh species, and (3) the impact that S. alterniflora removal has had on shorebird usage of affected tidelands.
Materials and Methods
Study Area
Willapa Bay, WA (46.5°N, 124°W) is a large, shallow, bar-built estuary that covers an area of 347 km2 at mean higher high water (MHHW) and 191 km2 at mean lower low water (MLLW) (Figure 1). The tidal range between MHHW and MLLW is 2.4 to 3.4 m. Willapa Bay is a major stopover along the Pacific Flyway and is designated a Site of International Importance (Western Hemisphere Shorebird Reserve Network 2017). It is utilized by 10% of the world’s population of short-billed dowitcher (Limnodromus griseus caurinus), red knot (Calidris canutus roselaari), and dunlin (Calidris alpina pacifica) and hosts between 200,000 and 300,000 individuals of 43 shorebird species during annual migrations. Willapa Bay is also home to a robust (>$60 milllion yr–1) shellfish industry that supplies ~17% of the nation’s oysters (Patten Reference Patten2014). Monitoring studies from 2004 to 2016 were conducted throughout the bay but focused mainly at numerous locations within the Willapa National Wildlife Refuge.

Figure 1 Map of Willapa Bay, WA, showing locations of major study areas and infestation of Spartina alterniflora (TS, Tarlatt Slough; PP, Porter Point; PR, Palix River).
Invasive Plant and its Control
S. alterniflora is a perennial, deep-rooted saltmarsh cordgrass native to the east coast of North America. It first became established in Willapa Bay in the early 1900s and gradually increased in coverage up to the mid-1980s. By 2004, >27,000 ha of the tidal mudflats of the estuary had been colonized by large meadows of S. alterniflora (WSDA 2017). S. alterniflora spreads clonally from underground rhizomes and disperses long distances by floating seeds. Clonal patches grow together to form dense, tall (2 m) meadows that entrap sediments, raising the elevation of the tidelands and transforming mudflats into emergent monotypic marshes (Grosholz et al. Reference Grosholz, Levin, Tyler and Neira2009; Strong and Ayres Reference Strong and Ayres2013). Control of large expansive meadows was achieved with the use of imazapyr applied at 1.68 kg ha–1 by boom (aerial or large amphibious tracked vehicles). Follow-up control was done using a tank mix normally consisting of 2% glyphosate, 0.75% mix of imazapyr, and 0.75% crop oil. It was applied with a high-volume hand gun, backpack sprayer, or small hand sprayer. Finding and treating cryptic S. alterniflora plants in the high salt marsh required slow, methodical searching on foot by multiple searchers spaced 4 to 20 m apart at least twice a year along all ~230 km of shoreline.
Probability of Successful Control.
The success of the program’s final phase is based on the ability of resource agencies to find and treat the remaining plants and prevent viable seed production. Finding new small-to-medium size S. alterniflora plants tightly nested with sedges and other masking saltmarsh species is challenging. A series of surveys was conducted between 2008 and 2010 to assess the efficacy of the resource agencies to search, find, and treat individual S. alterniflora plants within the salt marsh. A typical search pattern that would be employed during a spray operation was used to survey. The number of treated plants (showing discoloration caused by herbicide) and untreated plants (dark green) for each site during each survey time was recorded. Large plants (>1-m diam) were ignored for this survey. The density of S. alterniflora plants in these locations ranged from 10 to 1,500 plants ha–1. In 2008, five locations in Willapa Bay were surveyed on August 21 and then resurveyed on October 7. Survey sites ranged in size from 230 to 1,300 m2. In another 2008 survey, 35 sites within one large salt marsh (464 m2 plots) were surveyed in mid-October. In October 2009, 15 sites along a 10-km stretch of shoreline were surveyed. Each site was ~0.5 km in length. Also in 2009, six locations throughout the bay were surveyed from late September to mid-October. The areas surveyed ranged from 5 to 208 ha. In addition to the number of treated and untreated plants at each site, all untreated plants were located using global positioning systems (GPS). A follow-up survey was done in 2010 to determine if those 2009 untreated plants were found and treated by agencies in the following year. To assess if agency-treated plants died, 147 treated plants were flagged in the high salt marsh in October 2008. The proportion of those plants that died was recorded prior to the start of the new control season.
Agency control success was based on three years of treatment records obtained from the Washington Department of Natural Resources. Daily records on the amount of chemical sprayed and the number of plants treated for three discrete areas for 2014 to 2016 were summed by year. Each area was surveyed and treated twice a year by the same crew. The areas surveyed and treated ranged from ~330 ha to ~750 ha of shoreline in the areas of the native salt marsh where recalcitrant S. alterniflora remained (~0.1 to 0.25 plants ha–1). Plants were small, 1 to 3 shoots, <0.5-m tall, <2 to 3 years old, and occurring mostly from regrowth from previously treated plants, not seedlings. The percent decline for each area and year was calculated based on plant numbers found and amount of chemical used per area each year.
Seed Viability
Resource agencies were asked to collect and save mature seed heads from small clones or plants when they found isolated clones that had not been treated. Samples were collected from late August to mid-October in 2007 (n=3), 2008 (n=58), 2010 (n=9), 2013 (n=11), 2014 (n=66), and 2016 (n=11). Samples were also collected in 2012 and 2015 but had rotted during the collection process and were not used to assess viability. Seed viability was assessed by placing samples in mesh bags in aerated fresh estuarine water (Davis et al. Reference Davis, Taylor, Civille and Strong2004). Water was changed every two weeks. The number of seeds per sample ranged from 16 to 20,000, with a median of 122 seeds per sample. Percent germination was determined after 5 months.
Post-Control Salt Marsh Succession.
The total area of Willapa Bay that was formerly intertidal mudflats, then colonized by S. alterniflora, and then permanently lost to salt marsh succession, was measured using Google Earth’s July 2014 satellite color photos. Tideflats previously occupied by S. alterniflora in Willapa Bay were identified. These areas were distinguished by their green-brown color, distribution patterns, and ground truthing. When the distinctions between new and old marsh grass were not clear on Google Earth, aerial photographs from before and during the early S. alterniflora invasion were used to locate and mark the former wetland boundary. These photos were either 1994 Washington Department of Natural Resources aerial infrared photographs or 1992 or 1996 aerial photographs. The areas were traced to determine the exact surface area that was formerly S. alterniflora, and that is now occupied by salt marsh species. An example tracing of four S. alterniflora clones that succeeded to salt marsh is shown in Figure 2. Areas smaller than ~4 m2 or those that were too difficult to easily distinguish were not included. To verify the accuracy of the area of the tracings and that the newly formed salt marsh areas were not macroalgae, 24 locations in all major sections of the bay were visually ground truthed. The surface area obtained by tracing the area on foot (mean size of each traced area was 798 m2) with GPS was compared to that obtained from the satellite photos. The linear relationship between the areas recorded from GPS tracing on foot and that from satellite tracing was R2=0.998. The total area of Willapa Bay that was formerly intertidal mudflats, then colonized by S. alterniflora and succeeded to saltmarsh, was calculated by adding up all the areas traced.
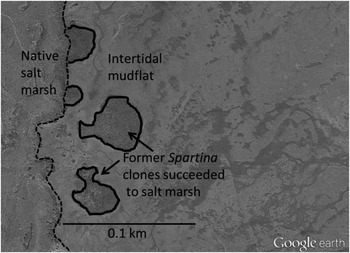
Figure 2 The four areas outlined above (0.234 ha) were former intertidal mudflats along the Long Beach Peninsula in Willapa Bay, WA, that were colonized by Spartina alterniflora, and then succeeded to salt marsh species after S. alterniflora was controlled by herbicides. Satellite photo from July 30, 2014 Google Earth.
In 2004 we initiated a study to evaluate the succession of salt marsh species that became established following the control of the ~300 ha Porter Point S. alterniflora meadow (Figure 1). At its peak the meadow covered ~2.5 km of shoreline and extended ~2 km out into the bay. The area was broadcast treated with imazapyr from 2003 to 2005, with follow-up spot treatment to remove individual plants in subsequent years. Eight sets of two permanent parallel transects were laid out 33-m apart extending outward for 600 m (south to north) starting at the native marsh (~2.9-m elevation above mean low tide) and extending approximately halfway out to the farthest portion of the former S. alterniflora meadow into the lower tidal zone. The transects were located along a 1,400 m section in the middle of the meadow. The change in transect elevation was gradual, averaging 0.1-m drop every 100 m along the transect. Plant density (percent cover by species) was assessed using quarter-meter quadrats randomly dropped every 33 m along the transect lines by visual approximation. Data were collected yearly from 2004 to 2012 and in 2015. Frequency of occurrence within a quadrat was calculated for each species. Results for annual frequencies by species are presented for pooled quadrats every 66 m. Results for pooled percent cover every 99 m for the major plant species are provided for 2009, 2012, and 2015.
Shorebird Census
The change in shorebird foraging density was monitored in three large solid S. alterniflora meadows during and after the control effort. The Porter Point Meadow was ~300 ha; the Tarlatt Slough meadow was ~70 ha; the Palix River meadow was ~175 ha (See Figure 1 for locations). All sites were historic prime foraging areas for shorebirds along the Pacific Flyway during migration prior to becoming S. alterniflora meadows (Buchanan and Evenson Reference Buchanan and Evenson1997). Large-scale control using herbicides was initiated in 2003 at Porter Point, 2004 at Tarlatt Slough, and 2005 at Palix River. All three sites were relatively free of live S. alterniflora and residual dead stubble by summer of 2006. Shorebird usage during the spring migration window was monitored from 2004 to 2011 at Porter Point, 2004 and 2007 to 2011 at Tarlatt Slough, and from 2005 to 2010 at the Palix River. Monitoring was done within 100-m by 100-m permanent plots. Plots were marked with 3-m sections of polyvinyl chloride pipe in each corner. Plots were randomly established within each meadow. A single observer monitored the plots from ~300-m distance using a spotting scope with a 20-60 X lens (Leupold, Beaverton, OR). Porter Point had three monitoring plots and Tarlatt Slough and Palix River had six monitoring plots each. All birds already present or landing in the subplot during a 10-min interval were counted. Plots were monitored in random order within a 2-h period of the optimal observing time (just prior to tidal coverage or tidal withdraw; Buchanan and Evenson Reference Buchanan and Evenson1997). Observations were made five to seven times during each spring migration on days when weather conditions allowed. Observations were made by species but data are presented collectively as all shorebirds. Most birds (98%) were small Calidris sandpipers, including dunlins (C. alpina pacifica) and western sandpipers (C. mauri).
Results and Discussion
Probability of Successful Control
The success of finding and treating S. alterniflora plants within the salt marsh ranged by year and survey (Table 1). The lowest percentage of total S. alterniflora plants found and treated in the salt marsh was 41%, the highest 84%. The surveys done in October indicated almost twice the success rate of finding S. alterniflora as surveys done in the summer (mean of 83% vs. 44%). For 2008 and 2009, the average end of the season detection following two passes through an area was 68%. The probability of finding a missed plant the second year of a search almost doubled (77% from 41%). According to agency records for 2014 to 2016, the annual decline in Spartina for a discrete area was 37% based on the amount of chemical used and 34% based on the number of plants treated. This compares similarly to the 35% average annual decline in S. alterniflora bay-wide since 2010 (WSDA 2017). The disconnect between end of the season percent detection (68%) and end of the season percent decline (35%) would suggest either a major influx of new seedlings or an average kill rate of only 52%. Agencies reported that seedlings only made up a very small proportion of their treatment ground; therefore a low kill rate is likely the problem. In our experiment, flagging each treated plant to record the follow-up death rate, we only recorded 53% of the plants completely killed from imazapyr. This is much lower than the average of 89% reported S. alterniflora control with imazapyr under experimental conditions (Patten 2003). Agencies report that the S. alterniflora plants they are finding are most often regrowth found in areas that have been previously treated, suggesting a failure to deliver a lethal dose of imazapyr to the entire underground rhizome. This regrowth from unaffected subterranean tissue has been problematic in the ability to obtain complete control of another aggressive invasive perennial grass, cogongrass [Imperata cylindrica (L.) Beauv.], with a single application of imazapyr (Dozer et al. Reference Dozier, Gaffney, McDonald, Johnson and Shilling1998; Willard et al. 1996). For S. alterniflora control, the label only allows one herbicide treatment per year. An alternative scenario for the occurrence of recalcitrant S. alterniflora is that populations of S. alterniflora in Willapa Bay could have developed resistance to imazapyr due to its continuous use since 2004. Imazapyr-resistant Mexican grass [Ixophorus unisetus (J. Presl) Schltdl)] and goosegrass [Eleusine indica (L. Gaertn.] have been reported in Latin America after just five years of consecutive use (Valverde et al. Reference Valverde, Chaves, González and Garita1993). The fact that agencies have been using a combination of imazapyr and glyphosate to treat S. alterniflora during most of their control efforts would suggest that imazapyr-resistant S. alterniflora, while possible, is unlikely. However, sites with persistent regrowth problems have never been assessed for imazapyr resistance. Agencies are unwilling to risk any seeds being produced and remove any seed heads they find, thus preventing access to divergent genetic material to assess herbicide resistance.
Table 1 The probability of finding and treating Spartina alterniflora, and annual decline in S. alterniflora in Willapa Bay, WA.

Seed Viability
Total mean germination for the 158 samples collected between 2007 and 2016 was 1.5% ± 0.57 SE, with 14.6% of the samples having viable seeds. Of the 26 samples with viable seeds, the average germination was 8.8% ± 1.1 SE. Percent germination varied by year from 0.3% to 40%. This variation might reflect the quality of samples collected rather than interannual variability. Because the samples were not collected at ideal times (mid-August to mid-November) or stored under ideal conditions (vehicle for weeks) and were often moldy when obtained, our germination values are likely to be very conservative. In 2016, for example, most of the samples were collected late in the season. Only a few were moldy and 91% of them had viable seeds, with a mean germination of 3.6% ± 1.5 SE. Overall, these results suggest that there is likely some selfing in these isolated clones and that the self-sterility of S. alterniflora as suggested by Strong and Ayres (Reference Strong and Ayres2013) is only partial. In addition, it would indicate that the weak Allee effect reported for these clones that results from pollen limitation (Davis et al. Reference Davis, Taylor, Civille and Strong2004) was not adequate to prevent viable seeds from being shed from small S. alterniflora plants separated from each other by several kilometers.
Feasibility of Eradication
Based on the number of plants treated in Willapa Bay in 2016 (1,338), an extirpation model using a 35% decline per year would require a continued effort out to 2035 before there were <0.5 plants bay-wide. Using the 64% decline per year value, which was the overall decline report for Willapa Bay between 2015 and 2016 (WSDA 2017), it would take until 2024 to achieve <0.5 plants bay-wide. This model presupposes that no mature clones that produce viable seeds were missed. Our results, however, indicate that small clones of S. alterniflora separated by several kilometers from similar plants are very capable of producing viable seed. For example, in 2010, one sample obtained from a 1-m diam clone produced ~5000 seeds that germinated, and in 2016 one isolated sample with 23 flowering culms produced 204 seeds that germinated. One or two escaped fecund clones with seeds could easily reset the extirpation timeline, and it has been difficult to find and eliminate all seed production in Willapa Bay. Finding remote isolate clones in small headwater sloughs well outside the normal search pattern has been challenging.
An additional variable confounding the extirpation model is landowner lawsuits to prevent chemical control of S. alterniflora on their property. One such lawsuit was successful. The court required the landowner to achieve eradication by mowing their S. alterniflora multiple times per year. This effort, while reducing the stand density, did not prevent S. alterniflora from seeding. This minor adversarial conflict in invasive species management will only result in slight delay in the extirpation rate. A much more complicated management scenario exists in San Francisco Bay salt marshes, where Spartina eradication and ecosystem restoration goals are in conflict with the Endangered Species Act (ESA). In these marshes, the endangered California Ridgeway’s rail (Rallus obsoletus obsoletus) obtains refuge in the tall vegetative cover provided by the vigorous growing hybrid of invasive S. alterniflora and the native California cordgrass (S. foliosa Trin.) (Casazza et al. Reference Casazza, Overton, Bui, Hull, Albertson, Bloom, Bobzien, McBroom, Latta, Olofson, Rohmer, Schwarzbach, Strong, Grijalva, Wood, Skalos and Takekawa2016). Control of the hybrid S. alterniflora × S. foliosa has been restricted at 11 sites containing 80% of the remaining invasive (Kerr et al. Reference Kerr, Hogle, Ort and Thornton2016). Every 2.5% reduction in the hybrid S. alterniflora population resulted in a 1% decline in California rail population growth rate (Casazza et al. Reference Casazza, Overton, Bui, Hull, Albertson, Bloom, Bobzien, McBroom, Latta, Olofson, Rohmer, Schwarzbach, Strong, Grijalva, Wood, Skalos and Takekawa2016). Adaptive management strategies have been designed and are being implemented to ameliorate the conflict between the control effort and ESA enforcement. This includes the use of herbicide for only seed suppression, along with revegetation, creation of refuge islands, management of rail predators, and rail breeding (Casazza et al. Reference Casazza, Overton, Bui, Hull, Albertson, Bloom, Bobzien, McBroom, Latta, Olofson, Rohmer, Schwarzbach, Strong, Grijalva, Wood, Skalos and Takekawa2016; Kerr et al. Reference Kerr, Hogle, Ort and Thornton2016).
Eradication, not extirpation, is the mandated goal for Washington’s Spartina control program (Hedge et al. Reference Hedge, Kriwoken and Patten2003). Declaring eradication is a tradeoff between the costs to continue to search versus the risk for reestablishment (Regan et al. Reference Regan, McCarthy, Baxter, Dane Panetta and Possingham2006). A continued search with no detection must be maintained for three years before eradication can be declared in Willapa Bay. These search efforts, with or without detecting S. alterniflora, are expensive. The mean annual cost of the entire effort to manage Willapa S. alterniflora during the past five years has been ~$0.8 million y–1 (WSDA 2017), which equates to ~$3,500 km–1 of shoreline per year. Funding levels are expected to decline markedly over the next decade. Such reduction in control cost did not prevent extirpation of S. alterniflora in a small South African estuary (Ridden et al. Reference Ridden, van Wyk and Adams2016) but may be a concern in a large estuary like Willapa Bay. It will necessitate going from two full bay-wide patterns per year to one or fewer per year.
As these efforts decline, the risk of reestablishment will increase. How big is that risk? Probability of detection, seed bank persistence, and colonization rate are critical for evaluating risk of reestablishment (Panetta Reference Panetta2015; Regan et al. Reference Regan, McCarthy, Baxter, Dane Panetta and Possingham2006, Reference Regan, Chadès and Possingham2011). While missing plants that go to seeds is a risk, that risk is minimized by the one-year seed bank persistence (Xiao et al. Reference Xiao, Zhang and Zhu2009). The colonization rate is also of low importance for S. alterniflora in Willapa Bay. Based on historical evidence, it only increases at 12% per year (Dennis et al. Reference Dennis, Civille and Strong2011). Assuming any new colonization is geographically contained, any outbreak could easily be controlled with herbicides. The greatest risk for reestablishment is the combined low overall probability of detection of ~0.65 and the low probability of obtaining complete kill of the treated plants. Finding and retreating individual plants that resprout in subsequent years has been made easier with cloud-based plant mapping software (WSDA 2017) that all agencies currently use. If agencies’ claim is true that most of the shoots treated in 2016 were not seedlings but from previously treated plants that resprouted after several years, then full use of these mapping features could greatly facilitate re-detection and expedite eradication. Even with GPS features, finding a few grass shoots among tall sedge in the salt marsh has still been difficult.
If the long-term kill rate of these isolated clones is as low as our data suggests (53%), then anything that would improve herbicide translocation throughout the entire rhizome could shorten the time to eradication. Delaying the treatment timing of detected S. alterniflora until the fall instead of ‘when found’ would be expected to increase control (Patten Reference Patten2002). The larger plant size in the fall would allow for greater leaf area available for herbicide absorption. For another perennial grass, I. cylindrica, where complete kill with a single herbicide application has been problematic, enhanced efficacy has been found by using lower rates of imazapyr or glyphosate and with fall applications (Dozier et al. Reference Dozier, Gaffney, McDonald, Johnson and Shilling1998). Overall, assuming that there is enough sustained funding to assure that the S. alterniflora plants in Willapa Bay that release large quantities of viable seeds into the estuary are substantially eliminated, then final eradication of S. alterniflora in Willapa Bay is feasible within the next two decades. This is in contrast to other Pacific Coast estuaries, like San Francisco, where ESA issues hamper control of invasive Spartina spp. (Casazza et al. Reference Casazza, Overton, Bui, Hull, Albertson, Bloom, Bobzien, McBroom, Latta, Olofson, Rohmer, Schwarzbach, Strong, Grijalva, Wood, Skalos and Takekawa2016; Kerr et al. Reference Kerr, Hogle, Ort and Thornton2016; Strong and Ayres Reference Strong and Ayres2016) or like Humboldt Bay, CA, where the unwillingness to use chemical control (H.T. Harvey & Associates Reference H.T.2013) precludes an effective eradication strategy.
Post-Control Salt Marsh Succession
Based on areas detected on Google satellite photos, the total area of new salt marsh in Willapa Bay as a result of S. alterniflora-induced accretion was 208 ha. The bulk of this occurred in the south bottom of the bay (74 ha) and along a narrow band on the eastern edge of the Long Beach Peninsula. With the exception of Jenson Spit, there were very few hectares of new salt marsh around Long Island.
Spergularia canadensis (Pers.) G. Don (Canadian sandspurry), Cotula coronopifoli L. (brass buttons), and Salicornia pacifica L. (pickleweed) were the pioneer succession species (Figure 3). They occurred at high frequencies (up to 50%) out to ~300 m from the native marsh within the first three years after S. alterniflora was controlled. Spergularia canadensis extended its range out to ~500 m by 2007 to 2010 but gradually became uncommon at all distances by 2015. Cotula coronopifoli declined rapidly after 2008. Salicornia pacifica became the dominant species out to ~400 m and remained so until 2012. The frequency of Triglochin maritimum L. (seaside arrowgrass) gradually increased up to 2008 out to ~600 m and remained a frequency species up to 2015. Distichlis spicata (L.) Greene var. spicata (seashore saltgrass) frequencies out to ~200 m increased gradually, starting in 2008 and became the most frequent species in quadrats out to that distance by 2015. Carex lyngbyei Hornem (Lyngbye’s sedge) presence increased just along the native marsh fringe starting in 2010.

Figure 3 Frequency of occurrences of native marsh species over time by distance from native marsh line during succession following S. alterniflora control.
Total plant coverage increased with time out to 400 m (Figure 4). Between 2009 and 2015, assessment of succession by percent cover for every 100 m along the transect outwards indicated solid coverage of the tideflat by marsh plants by 2015 for the first 200 m. Overall plant coverage continued to increase over time in the 200 to 400-m zone but declined at 400 to 500 m. Coverage in the first 200 m shifted from being mainly S. pacifica is in 2009 and 2012 to mainly D. spicata in 2015. C. Lyngyei coverage in the first 100 m increased in 2015. T. maritimum increased over time to become the main cover in the 200 to 400-m zone by 2015. Succession ecology followed patterns previously reported for salt marshes in PNW coastal estuaries (Belleveau et al. Reference Belleveau, Takekawa, Woo, Turner, Barham, Takekawa, Ellings and Chin-Leo2015; Jefferson Reference Jefferson1973; Thom et al. Reference Thom, Zeigler and Borde2002). Early colonizing species, C. coronopifolia, T. maritimum, S. canadensis, and S. pacifica, were present during the first few years, followed by long-lived species after 6 to 11 years. Elevations for tidal marsh vegetation colonization were similar to those reported by Belleveau et al. (Reference Belleveau, Takekawa, Woo, Turner, Barham, Takekawa, Ellings and Chin-Leo2015) and Jefferson (Reference Jefferson1973).

Figure 4 Percent total cover of native marsh species over time by distance from native marsh line during succession following Spartina alterniflora control.
Shorebird Census and Long-Term Impact of S. alterniflora Control
Shorebird usage at all three locations increased markedly following S. alterniflora control (Figure 5). Shorebird usage was essentially zero when there was dense S. alterniflora at the initial observations and peaked at ~800 birds ha–1 for 10-min observations. At Porter Point, shorebird density stabilized starting in 2007. At this site, the monitoring plots were eventually succeeded by native marsh, which achieved 80% coverage by 2009. Post-succession, shorebirds at this site were observed mostly utilizing bare ground farther out in the mudflats. Shorebird density at the Tarlatt Slough site stabilized between 2008 and 2010 and peaked in 2011. Most of the monitoring plots at Tarlatt Slough remained free of post-S. alterniflora successional marsh species. Shorebird visitation continued to increase up to our last observation period at Palix River. The site remained free from succession by marsh plants.

Figure 5 Shorebird usage of three large Spartina alterniflora meadows over time post-control effort.
Long-distance shorebird migration is energetically demanding. Without a network of refueling sites, the continued success of migrating shorebirds is compromised (Newton Reference Newton2006). Willapa Bay is one of the major foraging stops along the Pacific Flyway (Buchanan and Evenson Reference Buchanan and Evenson1997). By 2003, large S. alterniflora meadows had eliminated most shorebird foraging in the upper-intertidal areas of Willapa Bay and threatened to compromise the refueling network (Buchanan Reference Buchanan2003; Patten and O’Casey Reference Patten and O’Casey2007). Shorebird visitation was reduced by as much as 67% (Jaques Reference Jaques2002). Between 2004 and 2008, the wide-scale use of the herbicide imazapyr to remove over 3,600 ha of solid S. alterniflora meadow in Willapa Bay resulted in one of the largest successful restorations of prime shorebird foraging habitat in North America. Shorebird utilization of affected sites during peak migration periods went from essentially nonexistent to >800 ha–1 per 10-min observation period within only a few years from the initial treatment. Because of the dimension of restoration success, this effort essentially prevented the loss of Willapa Bay for shorebirds, preserving it to become a site of international importance (Western Hemisphere Shorebird Reserve Network 2017).
Besides the direct effect of the S. alterniflora canopy on shorebird usage of the mudflats, the increased tidal elevation that results from accelerated accretion in S. alterniflora meadows may result in colonization by salt marsh species before the infested tide flats can return to their pre-invasion elevation (Belleveau et al. Reference Belleveau, Takekawa, Woo, Turner, Barham, Takekawa, Ellings and Chin-Leo2015; Hacker and Dethier Reference Hacker and Dethier2006; Reeder and Hacker Reference Reeder and Hacker2004). Shorebirds, like dunlins, which require bare mudflat for foraging (Goss-Custard and Moser Reference Goss-Custard and Moser1988; Page et al. Reference Page, Stenzel and Kjelmyr1999), would continue to be compromised even after the Spartina was removed. Fortunately, total bay-wide succession of S. alterniflora meadows to salt marsh meadow was limited to 208 ha (6% of total former S. alterniflora). While 74 ha of the salt marsh habitat in the South Bay could be considered permanent loss of prime shorebird habitat, it was limited to <1% of the area available for foraging in that area. This loss of foraging area is likely to be ecologically inconsequential to maintaining populations of migratory shorebird species.
Summary
This case study exemplifies how a long-term eradication effort using a very effective aquatic herbicide in a challenging tidal environment resulted in rapid decline of a recalcitrant invasive species. Previous attempts at controlling this aggressive, rapidly spreading invasive using mechanical and less-effective herbicides in this and other estuaries have not been at all successful (Hedge et al. Reference Hedge, Kriwoken and Patten2003; Patten and O’Casey Reference Patten and O’Casey2010). This study found that (1) detecting, treating, and killing isolated cryptic S. alterniflora plants in the high salt marsh is challenging and can have an annual success rate as low as 35%; (2) isolated S. alterniflora plants can be reproductively successful and eliminating any plants from going to seed is critical for long-term success; (3) long-term commitment of resources will be required to achieve eradication; (4) only a small percentage (6%) of accreted tidelands following a S. alterniflora infestation were permanently succeeded to salt marsh due to elevation change, and (5) almost immediate usage of restored habitat by shorebirds occurred following S. alterniflora removal.
Acknowledgements
The authors thank the federal (Willapa National Wildlife Refuge), state (Departments of Agriculture, Natural Resources, and Fish and Wildlife), and county (Pacific County Weed Control) agencies and the shellfish industry for their steadfast efforts to control Spartina alterniflora and cooperation in this project.