Breast cancer
Breast cancer (BC) has exceeded lung cancer to become the most commonly diagnosed cancer worldwide, with 2.3 million cases in 2020 alone (Ref. Reference Sung1). At present, 70–80% of early-stage, non-metastatic cases are curable (Ref. Reference Harbeck2). However, secondary/metastatic BC is considered incurable with the currently available treatments. Unfortunately, in 2020 there were over 650 000 BC-related deaths worldwide, contributing to approximately 7% of cancer deaths that year (Ref. Reference Sung1). Therefore, there is an unmet clinical need to understand what causes certain cancers to resist treatment and what drives metastasis.
BC is a heterogeneous disease showing molecular and histological diversity between patients, resulting in variability in disease outcome and response to treatment. Biomarker expression has been used successfully to stratify breast tumours into molecular subgroups, guide treatment options and to develop targeted treatments such as endocrine therapies. The current molecular biomarkers with clinical significance include the oestrogen receptor (ERα), progesterone receptor (PR) and human epidermal growth factor receptor 2 (HER2) (Ref. Reference Harbeck2). Additionally, BCs that are ERα/PR negative and lack HER2 amplification are grouped as triple negative breast cancers (TNBCs), which lack available targeted treatment options (Ref. Reference Curigliano3), although some advances are being made in subsets of TNBC through the use of immune checkpoint inhibitors (ICIs) (Refs Reference Cortes4, Reference Emens5, Reference Schmid6, Reference Schmid7) and/or antibody-drug conjugates (Ref. Reference Bianchini8).
However, there are still limitations with current BC treatments, where patients may relapse even with subtype-specific treatment regimens. Therefore, further stratification and the identification of more effective and actionable prognostic and predictive biomarkers are required to improve patient management.
This review aims to examine the known molecular consequences of the species of bacteria Fusobacterium nucleatum (F. nucleatum) within the tumour microenvironment (TME), potentially identifying actionable pathways modulated by the bacterium that may have relevance in the BC setting.
The microbiome and cancer
The human body is host to a large population of microbes, estimated at 10–100 trillion cells (Ref. Reference Turnbaugh9), the majority of which exist within the gastrointestinal (GI) tract. Due to the development of next-generation sequencing techniques, organs which were previously believed to be sterile have been revealed to host microbial populations (Ref. Reference Nejman10). Furthermore, the human microbiome is shaped via co-evolution with the host, resulting in large compositional variations between age, sex, diet and geographical location. Therefore, the microbiome may contribute to the diversity observed in disease outcomes and treatment response between patients.
The imbalance in the relationship between the host and the microbiota (dysbiosis) is characterised by a reduction in the diversity of microbes present, and a shift towards a population in which pathogenic bacteria dominate. With the microbiome recently included as a hallmark of cancer (Ref. Reference Hanahan11) growing evidence suggests that both cancer-protective and tumour-promoting species exist, and can influence susceptibility, development, therapeutic response and metastasis (Ref. Reference Helmink12) of certain cancers. Therefore, particular members of the microbiome could be, and have already been, identified as biomarkers with clinical importance, including the human papilloma virus (HPV), hepatitis B and C and the bacterium Helicobacter pylori (Ref. Reference Cullin13).
However, more microbial species have been identified in recent years within tumour tissue as a result of the development of high-depth next-generation sequencing of bacterial 16S ribosomal RNA and more complete databases of sequenced organisms (Refs Reference Cullin13, Reference Wood and Salzberg14, Reference Gevers15, Reference Petersen16, Reference Wooley and Ye17, Reference Goodman and Gardner18). Critically, these approaches have been expanded to also characterise low-biomass intra-tumoural microbiomes, including introducing stringent pipelines which account for background noise and contamination (Ref. Reference Nejman10), and mining shotgun sequencing data generated on tumour tissue biopsies (Ref. Reference Poore19).
A number of these newly detected intra-tumoural microbes have been shown to modulate or contribute to cancer (Ref. Reference Sepich-Poore20). Conversely, some species have been exploited for cancer treatments such as probiotic treatments given alongside conventional therapy regimes or bacteria-assisted tumour-targeting therapies (Refs Reference Sedighi21, Reference Flores Bueso, Lehouritis and Tangney22).
Importantly, in a study by Nejman et al. (Ref. Reference Nejman10) which characterised the link between the microbiome and different types of solid tumours using next-generation sequencing, breast tumours were shown to have a rich and more diverse microbiome compared to the other tumour types tested, including melanoma and lung, but not including the GI tract. Furthermore, they noted variation within the dominant bacterial taxa between the ERα+, PR+ and HER2+ subtypes of BC (Ref. Reference Hanahan11). Other studies have confirmed that there is an altered microbiome in breast tumours compared with healthy tissue (Refs Reference Hieken23, Reference Esposito24, Reference Klann25, Reference Meng26, Reference Smith27, Reference Tzeng28, Reference Urbaniak29, Reference Urbaniak30), the findings of which have been reviewed previously (Refs Reference Parida and Sharma31, Reference O'Connor32). The potential to utilise the bacterial signature of breast biopsy tissue to infer malignancy status has also recently been reported (Ref. Reference Hogan33).
Breast cancer-associated bacteria have been found predominantly to reside intracellularly, both within breast tumour epithelial cells and immune cells (Refs Reference Nejman10, Reference Fu34). However, the microbiome of distant organs such as those of the GI tract can also affect carcinogenesis and progression of BC by influencing factors such as diet, obesity, levels of free circulating oestrogens and immune modulation (Refs Reference Helmink12, Reference Bodai and Nakata35, Reference Fernandez36). Moreover, the microbiome of both distant organs and the site of the tumour has been linked to local and systemic impacts on cancer chemotherapy efficacy and toxicity (Refs Reference Helmink12, Reference Bawaneh37). Studies have also shown that modulating the gut microbiome before and during chemotherapy treatment could improve efficacy and reduce the incidence of adverse events (Refs Reference Aarnoutse38, Reference Chen39), and more specifically, the gut microbiome was used as a predictive biomarker for doxorubicin responsiveness in a 4T1 murine TNBC model (Ref. Reference Bawaneh37).
Furthermore, some bacterial species have been shown to alter the TME, which is important in tumour formation, progression, metastasis and drug resistance (Refs Reference Coussens and Werb40, Reference Whiteside41). Bacterial colonisation of the tumour has been shown to activate the intertwined processes of tumour-promoting inflammation and evasion of tumour destruction by the immune system (Fig. 1) (Refs Reference Hanahan11, Reference Ismail, Hampton and Keenan42). Investigations into how the intra-tumoral bacteria may influence the breast TME are only beginning. However, remodelling of the TME in BC by bacteria has already been shown using the 4T1 syngeneic model inoculated with Escherichia coli K-12, where increased type IV collagen deposition, increased matrix metalloproteinase 9 (MMP9) expression and altered distribution of tumour-associated macrophages were observed (Ref. Reference Esposito24). Additionally, intraductal injection of mouse teats with Bacteroides fragilis resulted in increased local inflammation, tissue fibrosis and higher T-cell infiltration than in control mice (Ref. Reference Parida43).
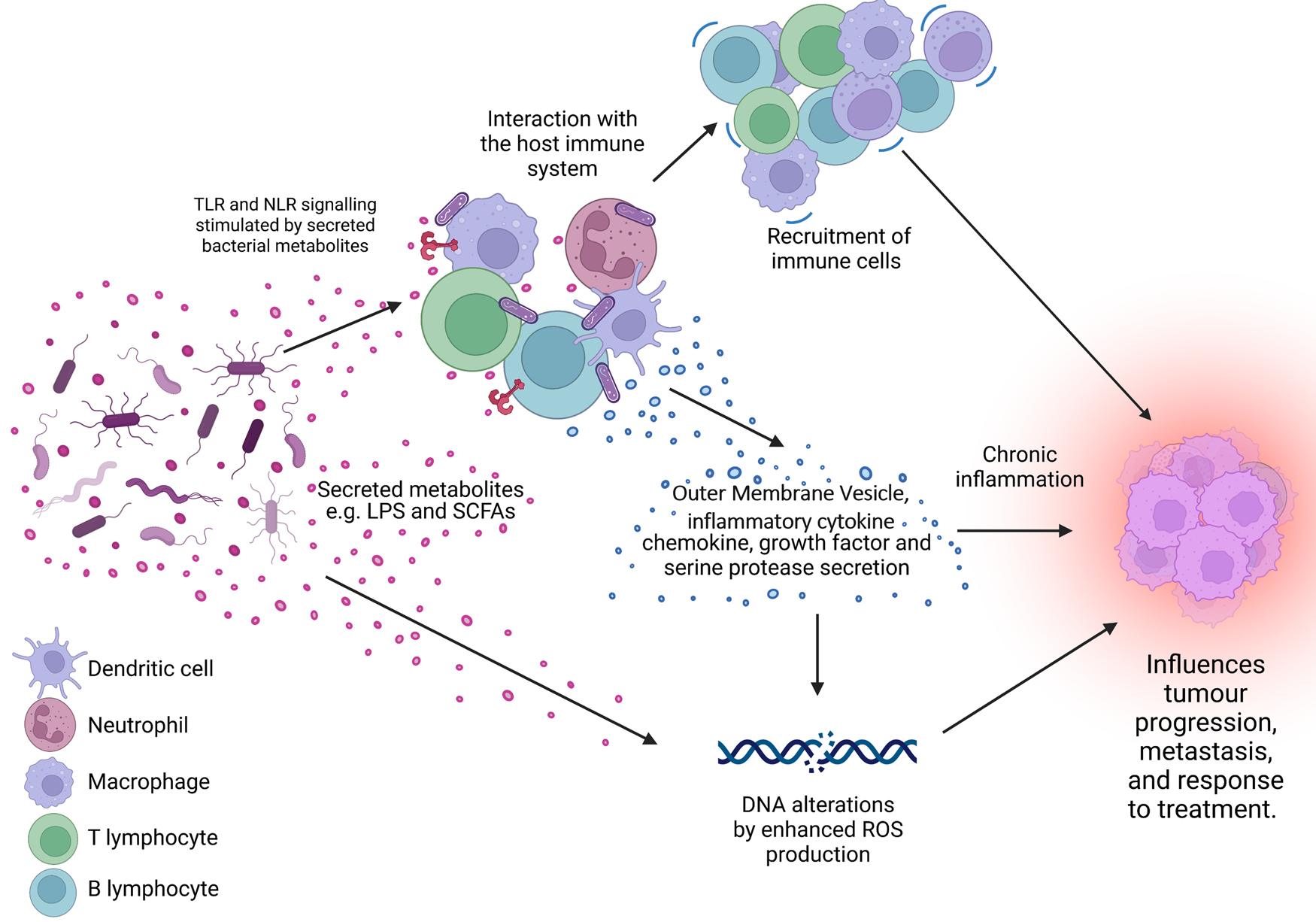
Figure 1. The microbiome is a key regulator of the tumour microenvironment (TME). Secreted factors and ‘immunomodulatory’ factors produced by bacteria can activate damage sensors on immune cells, for example, outer membrane vesicles which contain proinflammatory molecules such as lipopolysaccharide (LPS) on Gram-negative bacteria which stimulates Toll-like receptor (TLR)-4 signalling in immune cells. This activation results in the expression of a range of chemokines and cytokines, which further influence the recruitment and behaviour of immune cells within the TME and can lead to a state of chronic inflammation. Cells present in the TME can also produce growth factors and serine proteases which induce tumour progression. Furthermore, bacteria secrete metabolites such as short chain fatty acids (SCFAs) which can interact with the TME to reshape it, and/or cause genomic instability within the cells. LPS, lipopolysaccharide; SCFA, short-chain fatty acid; ROS, reactive oxygen species; TLR, Toll-like receptor; NLR, Nod-like receptor. Figure created with BioRender.
Fusobacterium nucleatum: an overview
F. nucleatum is a Gram-negative, anaerobic, adhesive bacterium and is commonly found within the oral mucosa where it aids in biofilm formation, supporting a normal oral microenvironment (Ref. Reference Lamont, Koo and Hajishengallis44). However, F. nucleatum has also been associated with adverse pregnancy outcomes (Refs Reference Vander Haar45, Reference Parhi46), appendicitis (Ref. Reference Swidsinski47) and importantly, many tumour types (Refs Reference Nejman10, Reference Castellarin48, Reference Kostic49). For example, F. nucleatum has been reported to be a potential biomarker for populations of colorectal cancer (CRC) (Refs Reference Flanagan50, Reference Guo51, Reference Chen52, Reference Zhang53).
Studies have shown that F. nucleatum presence in tumour tissue is associated with poor overall survival (OS) in oesophageal squamous cell carcinomas (ESCC), early-stage HPV-negative tongue cancer (Ref. Reference Desai54), as well as increased metastasis in CRC patients (Refs Reference Chen52, Reference Mima55, Reference Yamamura56, Reference Kunzmann57, Reference Galeano Nino58). However, in oral squamous cell carcinoma (OSCC), F. nucleatum presence is associated with a lower recurrence rate, reduced metastases and longer OS (Ref. Reference Neuzillet59). This highlights the complexity of host–pathogen relationships, and therefore the need for individual, context-specific studies.
Methods to detect and quantify specific microbes have advanced, and the development of RNA in situ hybridisation (Refs Reference Bullman60, Reference Borgognone61, Reference Zhang62), next-generation sequencing (Refs Reference Nejman10, Reference Kostic49) and qPCR on tumour tissue (Refs Reference Castellarin48, Reference Salvucci63) has enabled detection of F. nucleatum in both high- and low-biomass tumour tissues.
F. nucleatum was identified in approximately 30% of breast tumours by Nejman et al. (Ref. Reference Nejman10), and within other BC cohorts (Refs Reference Hieken23, Reference Urbaniak29, Reference Parhi64, Reference Hoskinson65, Reference Banerjee66). Additionally, while the abundance of F. nucleatum relative to cancer cells is low, it is shown to increase in abundance in higher stage breast tumours (Ref. Reference Tzeng28). However, the clinical significance has not yet been fully elucidated for F. nucleatum in the breast. Given the findings that F. nucleatum is associated with both favourable outcomes in OSCC, and adverse outcomes in CRC and ESCC, it will be important in the future to determine the significance of F. nucleatum in the breast on survival outcomes.
Parhi et al. (Ref. Reference Parhi64) showed that F. nucleatum promoted mammary tumour growth and, critically, metastatic progression when inoculated into mice. They suggested that this effect may be mediated by suppression of T-cell infiltration into the TME and/or increased expression of MMP9 (Ref. Reference Parhi64).
The oncogenic mechanisms of F. nucleatum in cancer
An important feature of F. nucleatum is its ability to bind to a variety of host and neighbouring bacterial cells via a range of virulence factors including the Fap2 protein that binds to the sugar D-galactose-β-N-acetyl-D-galactosamine (Gal-GalNAc) (Refs Reference Sung1, Reference Harbeck2, Reference Curigliano3)(Refs Reference Parhi64, Reference Abed67) which is overexpressed in CRC and BC (Refs Reference Parhi64, Reference Abed67). Specifically, F. nucleatum binds to tumour cells, influencing downstream oncogenic and pro-metastatic signalling (Refs Reference Casasanta68, Reference Chen69, Reference Chen70, Reference Chen71, Reference Hashemi Goradel72, Reference Rubinstein73, Reference Yang74). A summary of known oncogenic F. nucleatum interactions in CRC through F. nucleatum virulence factors is summarised in Figure 2 (Refs Reference Rubinstein73, Reference Rubinstein75, Reference Xu76, Reference Lu, Yeh and Ohashi77, Reference Ellis and Kuehn78, Reference Despins79). This review expands on the influence of F. nucleatum on the TME, and how these findings may guide the research into the relationship between BC and F. nucleatum.
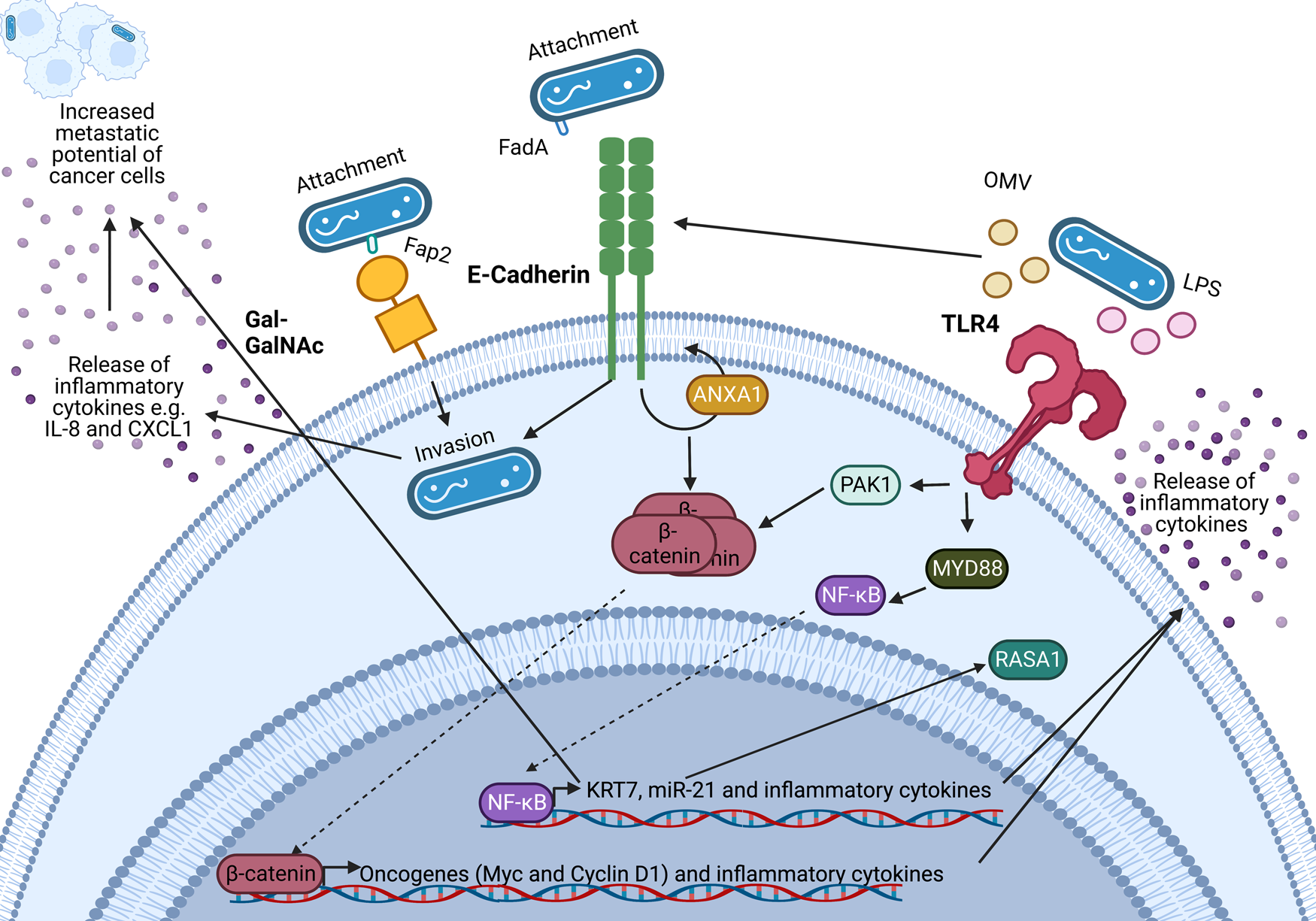
Figure 2. Known oncogenic pathways modulated by Fusobacterium nucleatum. F. nucleatum (shown in blue) binds to tumour cells via interaction of its Fap2 protein with D-galactose-β(1–3)-N-acetyl-D-galactosamine (Gal-GalNAc) or by FadA interacting with E-cadherin, which is enhanced by Annexin A1 (ANXA1), enabling attachment and invasion of tumour cells. F. nucleatum also secretes outer membrane vesicles (OMVs) and lipopolysaccharide (LPS) which interact with the Toll-like receptors (TLRs) to initiate downstream signalling pathways that mediate the release of inflammatory cytokines and transcription of miR-21 which is known to regulate the activity of the oncoprotein RASA1. The E-cadherin and TLR4 signalling induced by F. nucleatum binding stimulates β-catenin accumulation in the cytoplasm and its subsequent translocation to the nucleus where it upregulates transcription of oncogenes including c-MYC and Cyclin D1. Furthermore, F. nucleatum is able to aid metastasis through OMV-mediated degradation of E. cadherin, NF-κB mediated increased expression of keratin 7 (KRT7), and via induction of the inflammatory cytokines IL-8 and CXCL1. Figure created with BioRender.
Fusobacterium nucleatum and inflammation within the tumour microenvironment
Inflammation is one of the hallmarks of cancer, with up to 20% of cancers being preceded by chronic inflammation at the site (Refs Reference Grivennikov80, Reference Grivennikov, Greten and Karin81). While F. nucleatum can bind to cancer cells and activate oncogenic signalling directly, as observed in CRC, there is also evidence that F. nucleatum is able to indirectly promote tumour progression by modulating the inflammatory microenvironment.
F. nucleatum infection is closely linked to NF-κB signalling by numerous studies in multiple cell types (Refs Reference Salvucci63, Reference Rubinstein73, Reference Yang74, Reference Bui82, Reference Chen83, Reference Hung84, Reference Kostic85, Reference Nomoto86), however this link has not yet been investigated in BC. NF-κB signalling can be activated by bacteria through immune receptors including the Toll-like receptors (TLRs) to upregulate many chemokines and cytokines (described in further detail below). For example, TLR2 and TLR4 are implicated in F. nucleatum-stimulated macrophage cytokine production (Ref. Reference Park87). Constitutive activation of NF-κB signalling has been linked to inflammation and cancer (Ref. Reference Taniguchi and Karin88) via regulation of genes involved in cell proliferation, differentiation and innate and adaptive immune responses (Ref. Reference Liu89).
A number of studies have identified an inflammatory signature associated with F. nucleatum presence within CRC (Refs Reference Abed67, Reference Despins79, Reference Kostic85, Reference Proenca90). Specifically, F. nucleatum presence within human colonic tumours has been associated with the upregulation of the pro-inflammatory cytokines IL-6, IL-8 and IL-1β, among others (Refs Reference Despins79, Reference Kostic85, Reference Proenca90). It is possible that with further investigation into the breast TME, comparisons could be made between the effect of F. nucleatum in these two cancers.
In BC, upregulation of serum IL-6 levels is associated with poor prognosis (Refs Reference Shibayama91, Reference Dethlefsen, Hojfeldt and Hojman92), where hormone-sensitive tumour cells have a greater response to IL-6 (Ref. Reference Fontanini93). IL-6 has been linked to epithelial-mesenchymal transition (EMT) in BC and enhances mesenchymal stem cell recruitment in the breast TME (Refs Reference Korkaya94, Reference Madden, Szpunar and Brown95). Therefore, it is interesting that IL-6 secretion is induced by F. nucleatum infection in B lymphocytes (Ref. Reference Toussi, Liu and Massari96) and macrophages (Ref. Reference Chen83). Similarly, in CRC, Wang et al. noted that F. nucleatum infected CRC cells displayed an EMT cancer stem cell-like behaviour as a result of IL-6/STAT3 signalling (Ref. Reference Wang97).
Additionally, multiple studies have identified upregulated IL-8 as a result of F. nucleatum infection in CRC cells (Refs Reference Casasanta68, Reference Despins79, Reference Kostic85, Reference Toussi, Liu and Massari96, Reference Tang98). IL-8 in BC is associated with positive lymph node status and higher-stage tumours (Refs Reference Kozlowski99, Reference Ma100).
In colonic cells, F. nucleatum-secreted outer membrane vesicles, and the FomA porin that is present on them, induced IL-8 expression in a TLR2- and TLR4-dependent manner (Refs Reference Toussi, Liu and Massari96, Reference Engevik101), as a result of NF-κB signalling (Ref. Reference Martin-Gallausiaux102). TLRs recognise microbial products, such as lipopolysaccharide from Gram-negative bacteria like F. nucleatum and stimulate secretion of inflammatory mediators and/or activate immune cells. Extracellular vesicles were further found to induce IL-8 secretion in colonic epithelial cells in a TLR4-dependent mechanism (Ref. Reference Engevik101), again involving NF-κB signalling. F. nucleatum induces IL-8 expression through pathways involving increased reactive oxygen species (Ref. Reference Kang103), β-catenin signalling (Refs Reference Rubinstein73, Reference Rubinstein75) and invasion via its FadA adhesin (Ref. Reference Abed67), as depicted in Figure 3.

Figure 3. Known pathways induced by F. nucleatum binding that result in increased interleukin-8 (IL-8) secretion. (a) F. nucleatum infection in Caco-2 colorectal cancer cells impaired autophagic flux, which enhanced the production of TNF-α, IL-1β and IL-8 via the increase in reactive oxygen species (ROS). (b) F. nucleatum binding via its FadA adhesin to the sugar D-galactose-β(1–3)-N-acetyl-D-galactosamine (Gal-GalNAc) on colorectal cancer cells enables invasion, which further stimulates the release of IL-8 and CXCL1. (c) Outer membrane vesicles and the porin FomA secreted by F. nucleatum stimulate Toll-like receptors (TLRs) 2 and 4 on colonic epithelial cells, inducing NF-κB signalling that results in increased IL-8 secretion. (d) F. nucleatum's FadA adhesin binds to E-cadherin, activating β-catenin signalling in CRC cells, resulting in increased expression of pro-inflammatory cytokines, including IL-8. Figure created with BioRender.
Fusobacterium nucleatum and the tumour immune microenvironment
The studies highlighted in Table 1 provide abundant evidence that F. nucleatum is capable of altering the composition and actions of the immune cell population of the TME. It is possible that F. nucleatum promotes an immunosuppressive TME, enabling tumour cell escape from immune surveillance. While research into how the presence of F. nucleatum alters the immune response to other cancers is more advanced, little is known at this time with respect to the impact of F. nucleatum on the TME in BC. Given the importance of the immune response to BC and its impact on survival, drug efficacy and metastatic potential (Ref. Reference Badr, Berditchevski and Shaaban104), the presence of F. nucleatum and its known ability to alter the tumour immune microenvironment is an important area of future research.
Table 1. The effect of F. nucleatum on immune cells from different studies
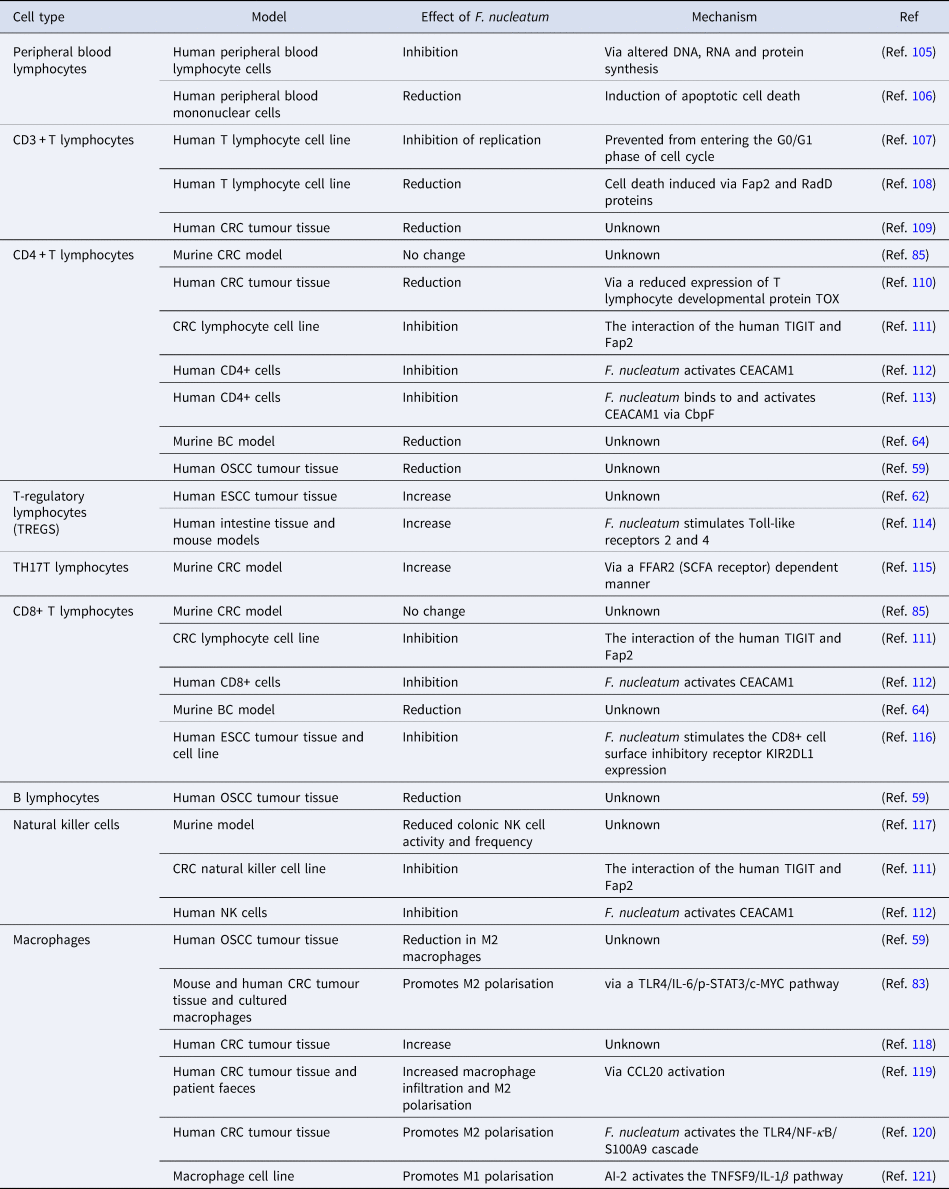
AI-2; autoinducer-2, BC; breast cancer, CbpF; chlorine-binding protein; CCL20, chemokine (C-C motif) ligand 20; CD, cluster of differentiation; CEACAM1, CEA cell adhesion molecule 1; c-MYC, cellular-MYC; CRC, colorectal cancer; DNA, deoxyribonucleic acid; ESCC, oesophageal squamous cell carcinoma; FFAR2, free fatty acid receptor 2; IL-1β, interleukin 1β; IL-6, interleukin-6; KIR2DL1, killer cell immunoglobulin-like receptor 2DL1; NF-κB, nuclear factor kappa B; NK, natural killer cell; OSCC, oral squamous cell carcinoma; p-STAT3, phospho-signal transducer and activator of transcription 3; RNA, ribonucleic acid; SCFA, short-chain fatty acid; S100A9, S100 calcium-binding protein A9; TIGIT, T-cell immunoreceptor with Ig and ITIM domains; TLR4, Toll-like receptor 4; TNFSF9, tumour necrosis factor ligand superfamily member 9; TOX, thymocyte selection-associated high mobility group box protein.
Fusobacterium nucleatum and tumour response to treatment
Treatment of BC is multi-faceted, using a combination of surgery, radiotherapy and/or systemic therapy guided by the cancer molecular subtype (Ref. Reference Harbeck2). However, drug resistance (intrinsic and acquired) often develops. F. nucleatum may influence treatment response in CRC, ESCC, OSCC and rectal adenocarcinoma. Given the presence of F. nucleatum in approximately 20% of BCs (Ref. Reference Nejman10), the importance of F. nucleatum as a biomarker which may aid in predicting response of BC subtypes to their treatments warrants further investigation. Additionally, F. nucleatum itself presents a potential therapeutic target, with antibiotic treatment successfully restricting growth and metastasis of mammary tumours in a mouse model, where the mice were inoculated with F. nucleatum (Ref. Reference Parhi64).
Fusobacterium nucleatum and chemotherapy resistance
As chemoresistance in BC is not yet fully understood, understanding mechanisms underlying drug resistance is vital to improve therapeutic approaches and clinical outcomes. Importantly, F. nucleatum has been reported to contribute to chemoresistance within CRC, ESCC and OSCC (Refs Reference Liu122, Reference Rui123, Reference Yu124, Reference Zhang125).
In CRC cell lines, F. nucleatum was shown to promote chemoresistance to oxaliplatin and 5-fluorouracil (5-FU) by upregulating autophagy (Ref. Reference Yu124) in a TLR4- and MYD88-dependent signalling pathway, and by preventing apoptosis via upregulation of ANO1 (Ref. Reference Lu126) or BIRC3 (Ref. Reference Zhang125). Additionally, F. nucleatum promotes chemoresistance to 5-FU as well as cisplatin and docetaxel in ESCC (Refs Reference Wang116, Reference Liu122, Reference Liang127) via upregulation of autophagy and preventing apoptosis. It is important to note that 5-FU is often used in BC treatment as a part of the FEC regime (5-FU, epirubicin and cyclophosphamide), in combination with docetaxel. Additionally, cisplatin is used in the neo-adjuvant setting for TNBC treatment (Ref. Reference Silver128). Furthermore, F. nucleatum induced autophagy is linked to CRC metastasis (Ref. Reference Chen70). These studies correlate with the observed poor patient response to neoadjuvant chemotherapy in ESCC tumours with high abundance of F. nucleatum (Refs Reference Yamamura129, Reference Wang130). Similarly, F. nucleatum was also shown to be enriched in OSCCs which were unresponsive to chemotherapy (Ref. Reference Rui123).
Fusobacterium nucleatum and radiotherapy resistance
Serna et al. (Ref. Reference Serna131) showed that chemotherapy and radiotherapy treatment was able to shift rectal adenocarcinoma tumours from F. nucleatum-positive to F. nucleatum-negative, which then showed improved relapse-free survival. However, any persistent F. nucleatum positivity correlated with a higher risk of relapse development.
Additionally, Dong et al. (Ref. Reference Dong132) demonstrated that oral administration of F. nucleatum in CRC mice impaired the efficiency of radiotherapy, promoted colonic inflammation, increased the volume and number of tumours present and further increased metastases.
With radiotherapy being a major adjuvant therapy for eradication of BCs, F. nucleatum within the tumour tissue may be an important biomarker that predicts treatment response to radiotherapy.
Fusobacterium nucleatum and immunotherapy
Immune checkpoint therapy inhibits the interaction between a T-cell inhibitory receptor and its canonical ligand(s), allowing T lymphocytes to elicit antitumour responses (Ref. Reference Topalian, Drake and Pardoll133). For example, programmed cell death protein 1 (PD-1) when bound to its ligand PD-L1 inhibits T-cell activation (Ref. Reference Ahmadzadeh134). While BC is considered to be less sensitive to immunotherapy than other cancers (Refs Reference Cardoso135, Reference Solinas136, Reference Parkes137), PD-L1 is still expressed on a small subset of BC tumour cells (Refs Reference Polonia138, Reference Humphries139), and is associated with TNBC and HER2 overexpressing BCs (Refs Reference Humphries139, Reference Bertucci and Goncalves140). Furthermore, treatment with ICIs such as atezolizumab has been approved for metastatic TNBC, and pembrolizumab improved clinical outcome for metastatic TNBC and high-risk early-stage TNBC (Refs Reference Heimes and Schmidt141, Reference Adams142, Reference Kwapisz143, Reference Schmid144, Reference Zacharakis145). Recently, the FDA has granted accelerated approval to pembrolizumab in combination with chemotherapy for high-risk early-stage TNBC and for metastatic TNBC whose tumours express PD-L1. Therefore, the impact that F. nucleatum has on altering response to immunotherapy across BC subgroups should be further investigated, as well as its potential as a biomarker able to identify patients which will benefit from it.
In both patients and mice with CRC, Gao et al. found that F. nucleatum presence was correlated with improved response to PD-1/PD-L1 blockade treatment (Ref. Reference Gao146). In the murine model of CRC, treatment with F. nucleatum enhanced anti-PD-L1 treatment response, and further improved survival (Ref. Reference Gao146). Moreover, when F. nucleatum treatment was combined with anti-PD-L1 treatment, there was a significant increase in the amount of CD8+ T lymphocytes in the TME. Cancers with higher populations of CD8+ T lymphocytes are expected to have the greatest response to immunotherapy (Ref. Reference Trujillo147). Therefore, it is possible to hypothesise that the alterations induced by F. nucleatum in CRC may result in a TME which responds more effectively to immunotherapy. However, a higher abundance of F. nucleatum in the patient's airways has been associated with a worse response of lung cancer to PD-1 blockade treatment (Ref. Reference Chu148).
Conclusions and future directions
F. nucleatum has been identified as a bacterial species which colonises the breast and recent findings indicate that it may contribute to BC progression and metastatic development (Ref. Reference Parhi64). However, the underlying pathogenic mechanisms are poorly understood, with few studies investigating the potential role of F. nucleatum in BC patient cohorts. Typically, F. nucleatum has been identified in approximately 20–30% of BC tumours (Refs Reference Nejman10, Reference Urbaniak29, Reference Parhi64), but correlation with clinical characteristics such as tumour stage or BC subgroup requires further investigation.
The literature from research into other cancer types, including CRC, indicates that F. nucleatum is able to modulate the local TME, promoting an inflammatory state and further interacting with and influencing infiltrating immune cells. The question of whether the presence of F. nucleatum in the TME of breast carcinomas will show the same trends in inflammation and immunomodulation requires further investigation. In particular, advanced in vitro models such as organoids could be beneficial to recapitulate how the hypoxic environment of the tumour influences the survival and growth of the anaerobic F. nucleatum. Additionally, in vivo models should be considered for further investigating the relationship between F. nucleatum in breast tumours with the tumour immune microenvironment (Ref. Reference Parhi64).
Multiple protocols have been suggested in order to quantify the presence of F. nucleatum in cancer patients, for example, a faecal F. nucleatum-based assay for CRC (Ref. Reference Huang, Peng and Xie149), and qPCR of F. nucleatum DNA in tumour tissue (Refs Reference Flanagan50, Reference Datorre150, Reference de Carvalho151, Reference Tunsjo152, Reference Yamamura153). However, current literature highlights the difficulties in detecting microbial DNA from human host tissues, which is exacerbated in low microbial biomass tumour tissues such as is seen in the breast (Refs Reference Bodai and Nakata35, Reference de Goffau154, Reference Walker155, Reference Walker, Tangney and Claesson156). Before F. nucleatum can be used as a biomarker for any cancer type, a sensitive, yet cost-effective assay must be developed to detect and quantify F. nucleatum in patients. Salivary F. nucleatum DNA has been identified as a non-invasive biomarker for CRC and gastric cancer diagnosis (Refs Reference Zhang53, Reference Chen157). Further research is required to determine if these findings could also apply to other F. nucleatum-linked cancers, including breast.
Targeting F. nucleatum in the tumour could potentially introduce an exciting novel treatment option. Parhi et al. (Ref. Reference Parhi64) showed that antibiotic treatment of a BC mouse model inoculated with F. nucleatum eliminated F. nucleatum from the tumour and further suppressed F. nucleatum-induced tumour growth. It is therefore tempting to consider antibiotics adjunct to current BC treatments to target tumour-promoting bacteria. However, given the role of the patient's microbiome in influencing drug efficacy (Refs Reference Helmink12, Reference Bodai and Nakata35, Reference Bawaneh37, Reference Aarnoutse38, Reference Gopalakrishnan158, Reference Routy159, Reference Lehouritis160), broad microbe-targeting treatments may not be beneficial. Interestingly, a F. nucleatum-specific bacteriophage, FNU1, has been recently suggested as a means to eradicate the oncobacterium from the tumour (Ref. Reference Kabwe161). Strong evidence supports the influence of the gut microbiome in response to cancer therapy, most notably ICIs (Ref. Reference Li162). Given the increasing use of ICIs in BC, especially for TNBC (Refs Reference Heimes and Schmidt141, Reference Adams142, Reference Kwapisz143, Reference Tarantino163), the potential interaction between F. nucleatum within the breast and ICI therapy (Ref. Reference Gao146) is an especially interesting area of future research.
In conclusion, by better understanding the consequences of the presence of this bacterium, it will provide valuable insights into the role of the microbiota in BC progression and how it influences treatment efficacy in patients.