1 Introduction
Lean thinking applied to product development, often called lean product development (LPD), has received positive and steadily increasing attention with the objective of revolutionizing product development (León & Farris Reference León and Farris2011). Originally witnessed at Toyota and labelled the Toyota Product Development System (Morgan & Liker Reference Morgan and Liker2006), LPD has evolved, from adapting the more mature theories and principles of lean manufacturing to the realm of engineering, to then become considered on its own. The theory includes inner principles and lean thinking concepts applied to product development such as waste (Oehmen & Rebentisch Reference Oehmen and Rebentisch2010), value/knowledge focus (Browning Reference Browning2000; Ward & Sobek Reference Ward and Sobek2014) and flow (Browning Reference Browning2000; Oppenheim Reference Oppenheim2004; Reinertsen Reference Reinertsen2007; Beauregard, Bhuiyan & Thomson Reference Beauregard, Bhuiyan and Thomson2014). Lean product development was described with its enablers, which has allowed for the construction of several models and frameworks suitable for lean product and process development practical implementation, theory-building and continuous research (Kennedy Reference Kennedy2003; Morgan & Liker Reference Morgan and Liker2006; Hoppmann et al. Reference Hoppmann, Rebentisch, Dombrowski and Zahn2011; León & Farris Reference León and Farris2011; Khan et al. Reference Khan, Al-Ashaab, Shehab, Haque, Ewers, Sorli and Sopelana2013; Ward & Sobek Reference Ward and Sobek2014). For instance, set-based design (SBD) (also set-based engineering or set-based concurrent engineering (SBCE)) has emerged as a key enabler of LPD. Indeed, SBD is a field of active research, with the purpose of either leveraging its principles based on the claimed efficiency of the design methodology (e.g., Raudberget (Reference Raudberget2015)) or integrating it into a framework for implementing lean product and process development as a whole (e.g., Khan (Reference Khan2012)). Nevertheless, studies on LPD in the industry show that its maturity of implementation in industry is low, no more than an introductory level and it has not been possible to find a company that coherently combines the LPD enablers into a whole to improve the company product development process in a lean way (McManus, Haggerty & Murman Reference McManus, Haggerty and Murman2005; Rebentisch Reference Rebentisch2008; Beauregard et al. Reference Beauregard, Bhuiyan and Thomson2014; Al-Ashaab et al. Reference Al-Ashaab, Golob, Urrutia, Gourdin, Petritsch, Summers and El-Nounu2016). With a focus placed on SBD, which is the design strategy in LPD, the objective of this current review is to find answers to the following questions:
(i) What is the state of the art of SBD literature?
(ii) What are the most important contributions to the SBD focusing on the development of a model and methodology?
(iii) What are the key aspects to consider when developing such a model and methodology?
The article consists of a study of SBCE research from 1987, the year in which publications on the Japanese product development practices began to appear, to 2017, which represents the end of the data collection. The review is drawn from an evidence-based systematic review procedure (Nissen Reference Nissen1996; NHS 2001; Tranfield, Denyer & Smart Reference Tranfield, Denyer and Smart2003), which is applied herein by using methods similar to (León & Farris Reference León and Farris2011) and (Baines et al. Reference Baines, Lightfoot, Williams and Greenough2006) in their reviews of LPD body of knowledge.
The remainder of the article is organized as follows. First, SBD theory and principles are discussed. Then the analytical framework and strategy used to review the literature are presented. The results of the review are discussed in Section 4 by means of quantitative analysis. Using the proposed framework, the selected contributions are then summarized and correlated in Section 5. New paths of research are discussed before the article is concluded in Section 6.
2 Set-based design
According to Clark & Fujimoto (Reference Clark and Fujimoto1991), the earliest decisions in product development have the largest impact on the overall quality of the product (effectiveness) and the overall cost of the project (efficiency). Many approaches to engineering design are focused on reducing cycle time following the famous motto ‘do it right the first time’. In terms of design strategy, this has often been translated into a need to propose the right solution as fast as possible. As observed by Sobek & Ward (Reference Sobek and Ward1996), when dealing with the development of complex products, many companies force the engineering teams to propose a feasible concept quickly so that it can then be optimized through numerous iteration loops. This pattern is understood as point-based because it focuses on one solution at a time and progressively refines it until all the stakeholders are satisfied with the outcomes. Figure 1 illustrates this view. A point in the design space represents a single solution from a given discipline while interacting with the others, whereas in Figure 2 the set of solutions is reflected by an area in the design space.

Figure 1. A point-based approach to the design of a complex product. Adapted from Ward et al. (Reference Ward, Liker, Cristiano and Sobek1995).
Another design strategy, the set-based approach, has been the subject of a number of publications over the past 20 years. It is one of the pillars of lean thinking applied to product development, again observed particularly in the automotive industry through companies such as Toyota, Honda, or Denso. Here, engineers may reason and communicate about an acceptable range of parametric values instead of the single best value at a time. Set-based design allows windows of possibilities to align gradually and therefore the best of all worlds to be projected. It is rather a convergence process than an evolution (Sobek & Ward Reference Sobek and Ward1996). Participants bring sets of possibilities to the table and juxtapose them to find an intersection of feasibility rather than successively criticizing and modifying a single option (Liker et al. Reference Liker, Sobek Ii, Ward and Cristiano1996). Figure 2 illustrates the approach.
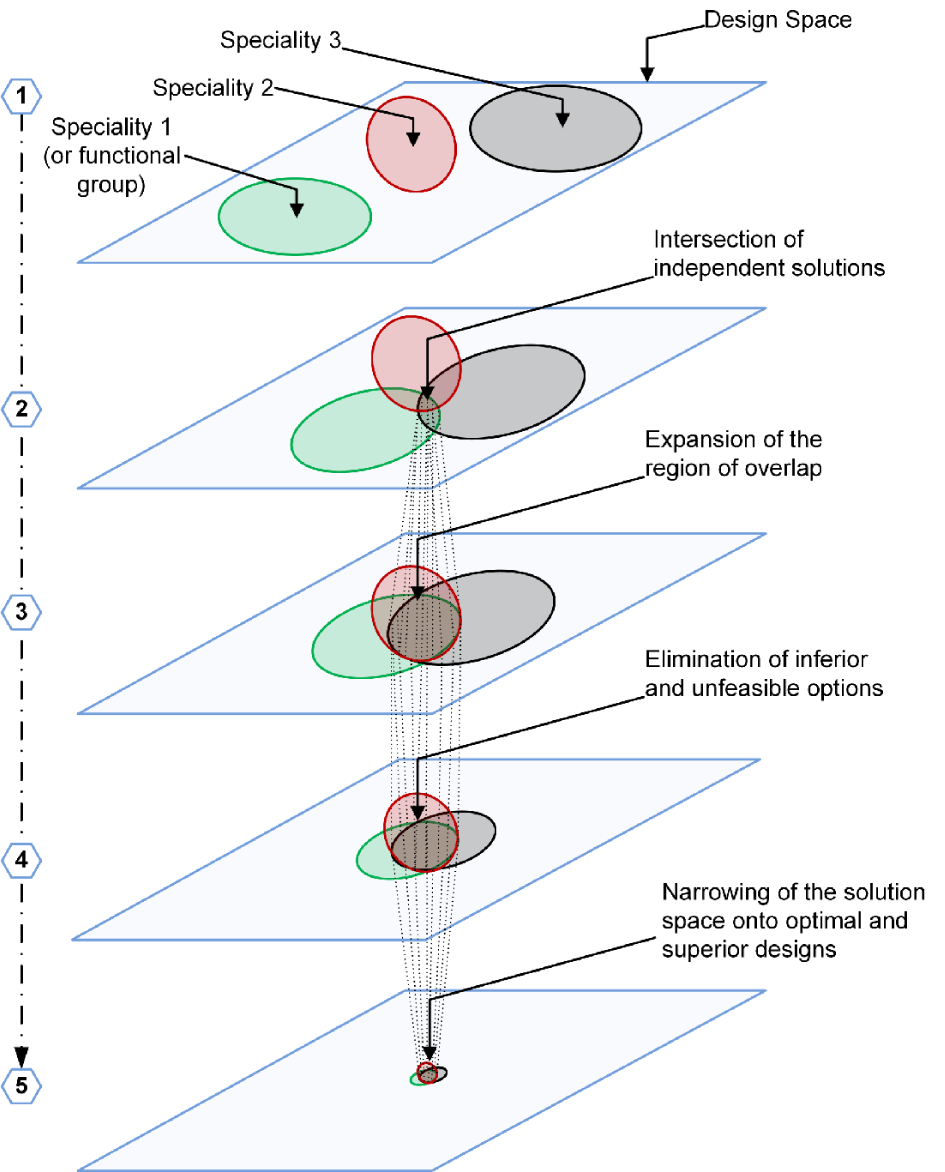
Figure 2. A set-based approach to the design of a complex product. Adapted from Bernstein (Reference Bernstein1998).
Set-based design space awareness and thorough exploration extend the classical vision by enabling a broad discovery of the design space. This avoids narrowing too quickly on discrete areas of the space and thereby losing the potential for innovation and discovery. However, the additional costs incurred by supporting multiple alternatives long into the development process could be detrimental. To help companies better strategize their set-based exploration efforts, Ward & Sobek (Reference Ward and Sobek2014) propose to divide their development projects into four categories: tailoring; strategic breakthrough; limited innovation & reintegration; research. The approach is combined with probabilistic calculations to determine the optimal number of alternatives/prototypes to pursue given the probability of failure of a system or subsystem design (product of the probabilities for the individual concepts). The expected cost of failure is also considered (cost to the whole system). The calculation essentially focuses on reducing the risk of a development project failure by indicating areas that require broader evaluations (cheap, early in the development process). This usually yields a higher probability of success compared to the probability of failure that results from unknowingly pushing a potentially weak option far in the product development process (PDP). This is confirmed by Camburn et al. (Reference Camburn, Arlitt, Perez, Anderson and Kun2017) and Camburn et al. (Reference Camburn, Dunlap, Gurjar, Hamon, Green, Jensen, Crawford, Otto and Wood2015) through empirical research. By aggressively eliminating weak options early in the PDP and thereby confidently concentrating on robust areas that can guarantee flexibility, discovery and innovation, set-based approaches to design make strategic prototype planning/execution a key factor of success in development programmes.
Sobek, Ward & Liker (Reference Sobek, Ward and Liker1999) described the three principles of SBCE, which according to Ghosh & Seering (Reference Ghosh and Seering2014) have remained the same as that used for SBCE discussions and implementations across the research and industrial applications.
(1) Map the design space:
(i) Define feasible regions
(ii) Explore trade-offs by designing multiple alternatives
(iii) Communicate sets of possibilities
(2) Integrate by intersection:
(i) Look for intersections of feasible sets
(ii) Impose minimum constraint
(iii) Seek conceptual robustness
(3) Establish feasibility before commitment:
(i) Narrow sets gradually while increasing detail
(ii) Stay within sets once committed
(iii) Control by managing uncertainty at process gates
Several methods like the morphological chart (Cross Reference Cross1989), the method of controlled convergence (Pugh Reference Pugh1991), the Design–Build–Test cycle (Wheelwright & Clark Reference Wheelwright and Clark1992), the fuzzy inference-based concept convergence process (Augustine et al. Reference Augustine, Yadav, Jain and Rathore2010) and the configurable component (CC) based platform (i.e., product platform strategy) (Wahl & Johannesson Reference Wahl and Johannesson2010) share similarities with SBCE (design strategy), in the sense that they all use the exploration of multiple alternatives to converge within the design space or the design bandwidth.Footnote 1 The main specificity of SBCE is twofold: (1) in SBCE, speciality groups can independently analyse their design options (sets of design alternatives) and then intersect at integration events, which then eliminates the iterative path that is problematic in the point-based approach (Sobek & Ward Reference Sobek and Ward1996; Bernstein Reference Bernstein1998); (2) SBCE delays decisions to learn by experimentation (extensive prototyping) and additionally front-load the design/development (reuse/recycling of existing knowledge) before narrowing the design space by elimination of unfeasible designs (Morgan & Liker Reference Morgan and Liker2006; Ward & Sobek Reference Ward and Sobek2014). Prototyping, learning and the reuse of existing well-structured information and knowledge are therefore important aspects of SBCE.
3 Review strategy and analytical framework
The methodology followed to perform the review is an evidence-based procedure described by Tranfield et al. (Reference Tranfield, Denyer and Smart2003). SBD theoretical grounds were presented in the previous section, which led to the extraction of important aspects of the approach such as the exploration of a design space, the extensive use of prototypes and the very specific concurrent engineering practices that involve the ability to communicate about sets. ‘Design space’, ‘prototyping’ and ‘concurrent engineering’ are selected keywords for the procedural search following this preliminary synthesis. From a practical product development standpoint, handling multiple design alternatives during the development process usually calls for configurability of the product (or the platform) as a prerequisite (Van Veen Reference Van Veen1991; Hegge Reference Hegge1995; Wortmann & Erens Reference Wortmann and Erens1995; Erens Reference Erens1996; Sabin & Weigel Reference Sabin and Weigel1998; Männistö et al. Reference Männistö, Peltonen, Soininen and Sulonen2001; Claesson Reference Claesson2006; Claesson & Johannesson Reference Claesson and Johannesson2006). This aspect is considered herein as a key element from a practical product development implementation perspective. As a result, the combination of keywords selected to query the research databases goes as follows:
(i) Keyword 1 (KW1): set-based + concurrent engineering
(ii) Keyword 2 (KW2): set-based + prototyp*
(iii) Keyword 3 (KW2): SBCE
(iv) Keyword 4 (KW3): set-based + design space
(v) Keyword 5 (KW4): set-based + configur*
As this work pertains to engineering sciences and the related cognitive and social sciences, the research databases selected for the data extraction are Compendex and Inspec (EBSCO) for engineering research publications and Web of Science (Thomson Reuters) to cover the broader landscape of sciences related to design as a cognitive and social science (for the dynamics of social organization). Databases like Proquest (ABI Inform), which contain dissertations and other institutional publications, are not included to focus on peer-reviewed work. This is done assuming that backtracking through the peer-reviewed work will allow us to consider the related comprehensive institutional publications. Similarly, backtracking allows us to explore influences and also new areas of the subject, which are not otherwise nominally related to the topic as queried. This mitigates the limitation of solely relying on keyword searches.
Table 1 displays the result of the data extraction. The extraction resulted in a total of 1733 publications. After duplicate records were removed, the remaining 921 records were collated to form the pool of publications to distribute by the categories 0 to 5 described below.
Table 1. Data extraction

The research area and the content of the 921 publications were examined to perform a preliminary assessment with the purpose of assigning a category to the publication as inspired by León & Farris (Reference León and Farris2011) and Nissen (Reference Nissen1996). The categories that emerged from the preliminary assessment are listed below by adhering to the distinctions (Gericke, Eckert & Stacey Reference Gericke, Eckert and Stacey2017) that must be made among a method, process, tool, guideline and a methodology:
(i) Category 0 (767): The publication is not relevant to the review because of the content and the classification of the research area, e.g., content related to set theory and pure level set methods, classifications such as graph theory, set theory, signal processing, mathematics, imaging science and photographic technology.
(ii) Category 1 (29): The publication develops on methods that facilitate SBCE, e.g., fuzzy sets, interval sets and computational design synthesis.
(iii) Category 2 (46): The publication explores the combination of SBCE principles with a complementary design method in order to accelerate or improve the design/development process, e.g., multidisciplinary design optimization combined with SBCE principles.
(iv) Category 3 (19): The publication emphasizes lean concepts with more or less considerations for SBCE.
(v) Category 4 (36): The publication reports on the practical application of SBCE principles and tools.
(vi) Category 5 (24): The publication emphasizes the development of SBCE theories, models and methodologies for SBCE practical implementation. This category consists of the 24 publications that are considered the most important contributions with regard to the second and third objectives of the review. The category contains the contributions to classify according to the new framework.
Indeed, a new classification framework is proposed in this review with the goal of mapping the topology of the most relevant SBD literature from two perspectives. The first is the coverage of the engineering design process when it comes to proposing/developing models and methodologies for the practical implementation of SBD, i.e., SBCE. The second is the research paradigms/methods chosen by the SBD research.
SBD belongs to the lean philosophy and as such can be assessed through the fundamental continuous improvement cycle, i.e., PDSA, Plan–Do–Study–Act (Deming Reference Deming2000), as well as the fundamental value creation cycle, i.e., LAMDA, Look–Ask–Model–Discuss–Act (Ward & Sobek Reference Ward and Sobek2014). An additional layer may also involve mapping each step of these cycles to the generic creative design process (Formulation–Synthesis–Analysis–Evaluation–Documentation–Reformulation) (Howard, Culley & Dekoninck Reference Howard, Culley and Dekoninck2008) as perceived from the SBCE perspective, i.e., Explore (formulate, map the design space); Generate (synthesize/analyse alternative designs); Learn (evaluate, document, communicate); Refine (overlap design options, eliminate weak options, reformulate). The practical implementation cycle steps aligned with the SBCE design process steps altogether result in four dimensions/areas for analysing the literature. These dimensions/areas can be decomposed into segments representing the granular concepts, approaches, techniques and catalysts for which the use and implementation in the literature might be explored with the purpose of proposing/developing models and methodologies intended to support the transition to SBCE. The proposed classification framework is shown in Figure 3 in the form of a continuous improvement cycle. Each segment is described further in Sections 4 and 5 by correlating it to the publications.

Figure 3. Set-based design process classification framework.
Another way to categorize the literature is to identify the research paradigm and the methods used in the research publications. The aim is to objectively perceive the purpose of the research, the type of enquiry, inference and construction used by the researchers, as well as the typical outputs and theory-building versus practical implications that can be expected from the research (Hoepfl Reference Hoepfl1997; Hart Reference Hart1998). The research methods in particular reveal the type of enquiry and, therefore, the type of answers a research work can provide. From an epistemological standpoint, researchers fundamentally take stances that can be explicit or rather implicit. Figure 4 shows the proposed SBCE literature research paradigm classification framework.
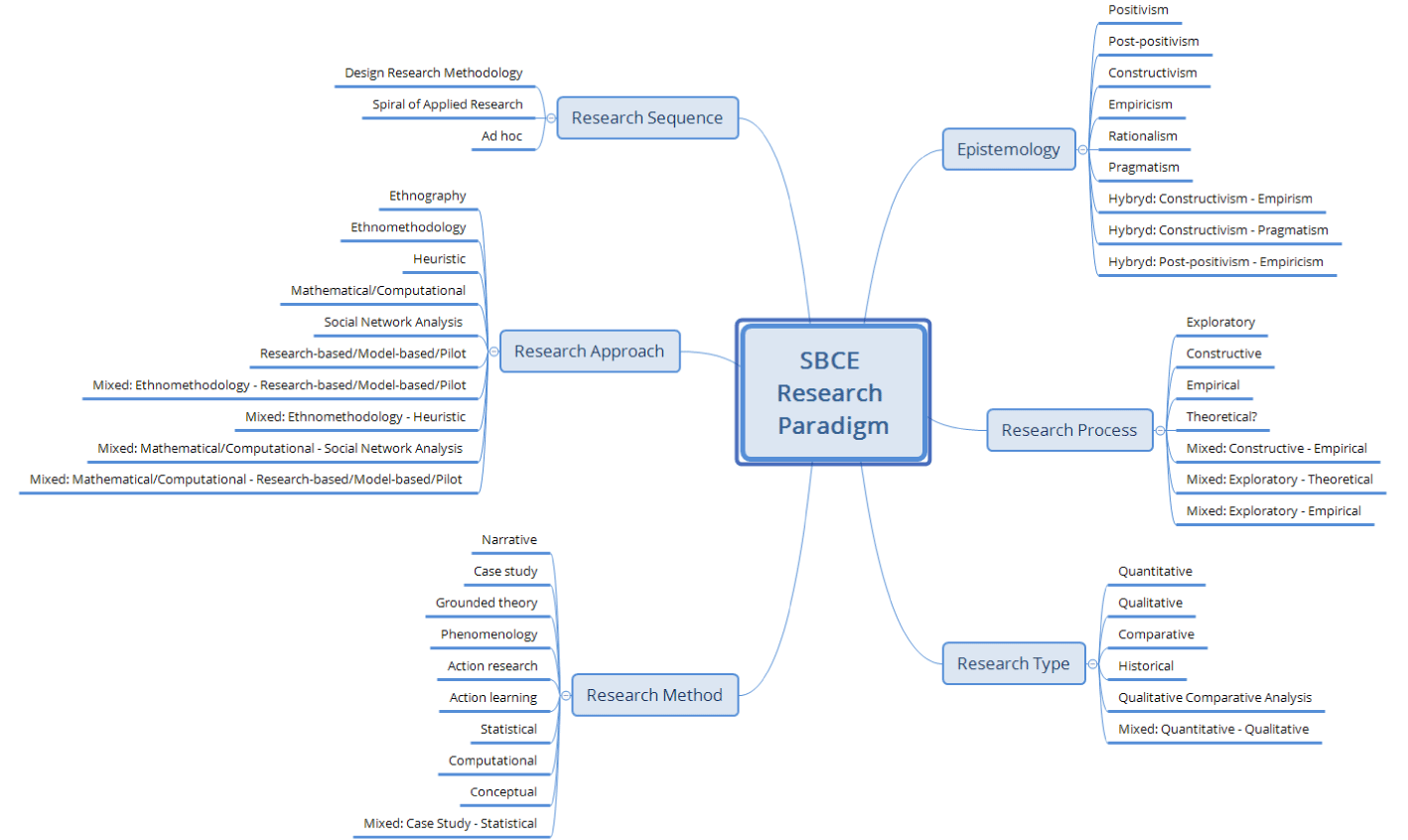
Figure 4. Research paradigm classification framework.
4 Progression, maturity and impact of SBCE research
The majority of publications (i.e., 46 out of 154) (30%) consists of research papers that combine SBCE principles with complementary design methods to improve the design/development process. The publications in this category 2 frequently focus on the design synthesis and selection process by portraying how the other method could adequately fit the SBCE concept generation, exploration and selection process. This denotes a growing interest in SBCE from diverse field areas. It also suggests a pervasive influence of SBCE, especially on methods and techniques for early stages of the development process. Examples, to name a few, include the combination of SBCE principles and techniques with the following:
(i) Multi-objective problem/multidisciplinary optimization (Moshaiov & Avigad Reference Moshaiov and Avigad2007; Avigad & Moshaiov Reference Avigad and Moshaiov2009, Reference Avigad and Moshaiov2010; Hannapel, Vlahopoulos & Singer Reference Hannapel, Vlahopoulos and Singer2012; Hannapel & Vlahopoulos Reference Hannapel and Vlahopoulos2014; Sasaki & Ishikawa Reference Sasaki and Ishikawa2015), Constraint Satisfaction Problem (CSP) (Ishikawa & Nahm Reference Ishikawa and Nahm2005; Nahm & Ishikawa Reference Nahm and Ishikawa2005; Nahm, Ishikawa & Yang Reference Nahm, Ishikawa and Yang2007; Qureshi et al. Reference Qureshi, Dantan, Bruyere and Bigot2010; Canbaz, Yannou & Yvars Reference Canbaz, Yannou and Yvars2011; Inoue et al. Reference Inoue, Lindow, Stark, Tanaka, Nahm and Ishikawa2012, Reference Inoue, Nahm, Tanaka and Ishikawa2013a; Wang et al. Reference Wang, Yannou, Alizon and Yvars2013; Yannou et al. Reference Yannou, Yvars, Hoyle and Chen2013; Kizer & Mavris Reference Kizer and Mavris2014)
(ii) Model-based systems engineering (ElMaraghy & Jack Reference ElMaraghy and Jack1993; Toche et al. Reference Toche, Pellerin, Fortin, Huet, Rivest, Bouras and Louhichi2012; Cho, Lee & Bae Reference Cho, Lee and Bae2014; Becker & Wits Reference Becker and Wits2015; Essamlali, Sekhari & Bouras Reference Essamlali, Sekhari and Bouras2016)
(iii) Game theory (Pourabdollahian, Taisch & Kerga Reference Pourabdollahian, Taisch and Kerga2012; Kerga et al. Reference Kerga, Rossi, Taisch and Terzi2014a,Reference Kerga, Rossi, Terzi, Taisch, Bessega and Rossob)
(iv) Bayesian networks (Shahan & Seepersad Reference Shahan and Seepersad2009, Reference Shahan and Seepersad2012; Matthews et al. Reference Matthews, Klatt, Seepersad, Haberman and Shahan2014)
Figure 5 displays the distribution of the 154 relevant publications by categories.

Figure 5. Distribution of category 1–5 publications.
Category 1 is very similar to category 2 with the only difference that category 1 focuses on the method itself (e.g., fuzzy set-based approach, interval set propagation, method of imprecision (MoI), etc.) and the means by which it can facilitate SBCE (e.g., Otto & Antonsson (Reference Otto and Antonsson1995), Ward (Reference Ward1989), Finch & Ward (Reference Finch and Ward1997)). The authors in category 1 typically display complementary publications in category 2 where the novel approach that combines SBCE with the expert method is forwarded, but the two categories are not always easy to separate on this sole basis. Category 1 examples include these works (Hernandez-Luna & Wood Reference Hernandez-Luna and Wood1994; Antonsson & Otto Reference Antonsson and Otto1995; Ong & Nee Reference Ong and Nee1998; Ong, Sun & Nee Reference Ong, Sun and Nee2003; Shan & Wang Reference Shan and Wang2003; Wang & Shan Reference Wang and Shan2004; Panchal et al. Reference Panchal, Gero Fernández, Paredis, Allen and Mistree2007; Zhai, Khoo & Zhong Reference Zhai, Khoo and Zhong2009; Hernandez-Luna, Moreno-Grandas & Wood Reference Hernandez-Luna, Moreno-Grandas and Wood2010; McKenney et al. Reference McKenney, Gray, Madrid and Singer2011a; Canbaz, Yannou & Yvars Reference Canbaz, Yannou and Yvars2014; Qureshi et al. Reference Qureshi, Dantan, Bruyere and Bigot2014; Kawakami et al. Reference Kawakami, Kami, Ishikawa and Xiao2016). One can therefore argue that categories 1 and 2 could merge into one single category about discrete methods that facilitate SBCE.
Category 4 comes in the second position with 36 of the 154 publications (23%). The category consists of publications on the practical application of SBCE principles and tools. Examples include these works (Giachetti Reference Giachetti1997; Ford & Sobek Reference Ford and Sobek2005; Parrish et al. Reference Parrish, Wong, Tommelein and Stojadinovic2008a; Ishikawa & Inoue Reference Ishikawa and Inoue2009; Raudberget & Sunnersjö Reference Raudberget and Sunnersjö2010; Takai Reference Takai2010; Raudberget Reference Raudberget2010a,Reference Raudbergetb; McKenney, Kemink & Singer Reference McKenney, Kemink and Singer2011b; Maksimovic et al. Reference Maksimovic, Al-Ashaab, Sulowski and Shehab2012; Rocha, Affonso & De Oliveira Reference Rocha, Affonso and De Oliveira2012; Kerga, Khan & Arias Reference Kerga, Khan and Arias2012a; Kerga, Taisch & Terzi Reference Kerga, Taisch, Terzi, Rivest, Bouras and Louhichi2012b; Neeley et al. Reference Neeley, Lim, Zhu and Yang2013; Rocha, Affonso & De Oliveira Reference Rocha, Affonso and De Oliveira2013; Inoue et al. Reference Inoue, Nahm, Tanaka, Ishikawa, Stjepandić, Rock and Bil2013b; Kennedy, Sobek and Kennedy Reference Kennedy, Sobek and Kennedy2014; McKenney & Singer Reference McKenney and Singer2014; Araci, Al-Ashaab & Maksimovic Reference Araci, Al-Ashaab, Maksimovic, Massaro and Garlatti2015; Landahl, Raudberget & Johannesson Reference Landahl, Raudberget and Johannesson2015; Bertoni et al. Reference Bertoni, Levandowski, Isaksson and Larsson2016; de Souza & Borsato Reference de Souza and Borsato2016; Heikkinen et al. Reference Heikkinen, Stolt, Elgh and Andersson2016; Landahl et al. Reference Landahl, Levandowski, Johannesson, Isaksson, Borsato, Wognum, Peruzzini, Stjepandić and Verhagen2016; Maulana et al. Reference Maulana, Flisiak, Al-Ashaab, Araci, Lasisz, Beg and Rehman2016; Elhariri Essamlali, Sekhari & Bouras Reference Elhariri Essamlali, Sekhari and Bouras2017). These publications are distinguished from the ones in category 5 as they do not attempt to develop actual SBCE theories, models and methodologies. The publications in category 5 consist of the 24 most important publications with regard to the main objectives of the review. They will be examined in detail in Section 5 by using the proposed analytical framework. The following subsections discuss the state of the art of SBD by means of quantitative analysis.
4.1 Progression of SBCE research
Bibliometrics are used in this section in order to obtain insights into the topology, progression, maturity and relevance of SBCE research. The age profiles of the publications from all categories (1 to 5) are shown in Figure 6.
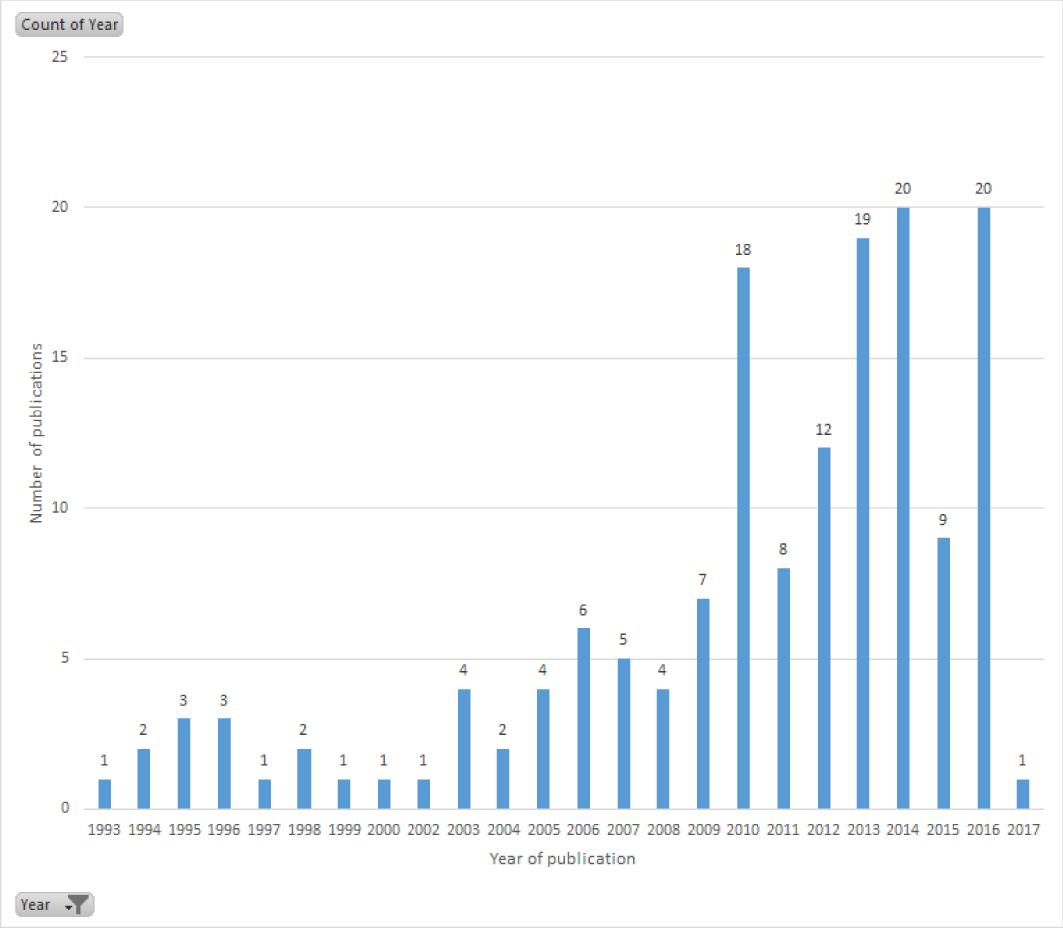
Figure 6. Age profile of the publications (category 1–5).
The profile discloses a fluctuating growth of SBCE publications from 1993 onward, with a proliferation starting around 2010. There are two potential reasons for the relative absence of SBCE related publications between 1987 and 1993. First, set-based concurrent engineering, as it refers to Toyota’s practice, was coined by Ward et al. (Reference Ward, Liker, Sobek and Cristiano1994) in their landmark paper presented at the proceedings of the 1994 ASME Design Technical Conferences in Minneapolis, USA. Second, even though early 90s’ research on engineering design calculations clearly involved set-based reasoning (see fuzzy parameters, labelled interval calculus and other methods like the MoI and constraint satisfaction) (Antonsson & Wood (Reference Antonsson and Wood1989), ElMaraghy & Jack (Reference ElMaraghy and Jack1993), Hernandez-Luna & Wood (Reference Hernandez-Luna and Wood1994), Hyvönen (Reference Hyvönen1992), Tommelein (Reference Tommelein1989), Ward (Reference Ward1989), Wood, Otto & Antonsson (Reference Wood, Otto and Antonsson1992)), they did not entail the holistic SBCE philosophy and principles, institutional knowledge capture and reuse advocated enabling a superior product development (PD) process (see previous descriptions of LPD and SBCE). Moreover, these early research efforts involving set-based methods were not always nominally identified as such, which is the reason why some may not be retrieved by keyword search. The complementary publications are discussed in this article as discovered by backtracking through the references. The proliferation of SBCE research in the 21st century, especially in the past 10 years, suggests a growing interest in the field, which might be generated by a context of compelling socioeconomic factors in the aftermath of the global financial crisis (2007–2008). These factors have demanded responsive, flexible, agile and LPD processes for companies to stay competitive in rapidly changing markets. There might be other reasons for the proliferation, which could not be established on the sole basis of evidence from this review.
Table 2. Total Cites, Impact Factor and Eigenfactor Score
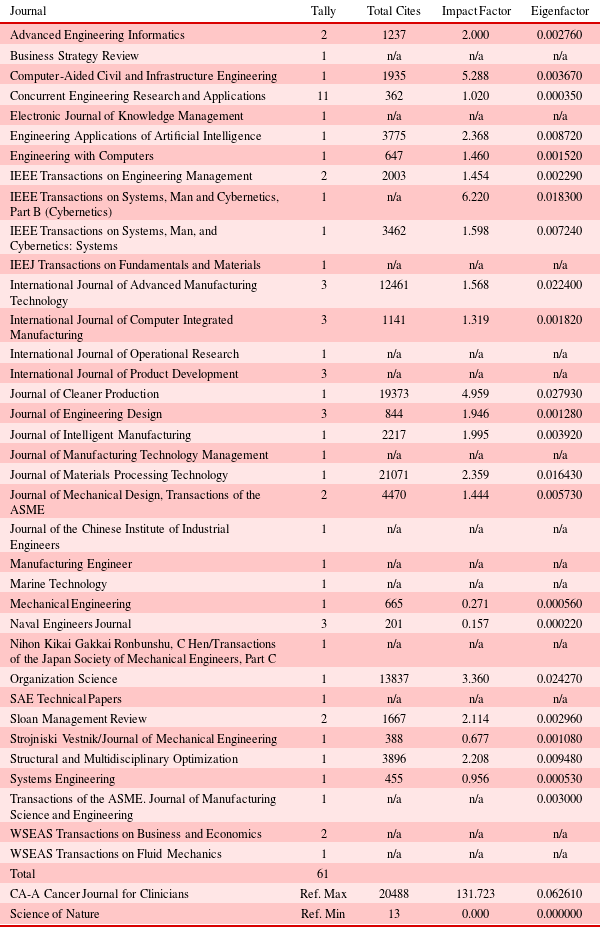
4.2 Maturity and impact of SBCE research
Following the restriction to peer-reviewed publications, the pool of 154 relevant publications is split between journal articles (40%) and proceeding papers (60%). The authors concur herein with León & Farris (Reference León and Farris2011) that the relative number of publications in scientific journals remains an objective indicator of fundamental and theoretical development into a field. Consequently, it can be argued that the relatively small number of journal publications on SBCE indicates a low theoretical development of SBCE. Nevertheless, a wide variety of journals (36) have published SBCE related papers. This variety is the result of SBCE being explored from diverse perspectives, i.e., knowledge domains.
The age profile chart shows evidence of a growing popularity and interest in SBCE, but it provides less information on the maturity and importance of the field of research. Assessment variables that can support such analysis include the ratio between peer-reviewed and non-peer-reviewed publications, the count of publications in journals and the ranking of the journal by indexes such as the Total Cites, Impact Factor (Journal Citation Reports published by Thomson Reuters) and the Eigenfactor
score(eigenfactor.org). Table 2 lists the Total Cites, Impact factor and Eigenfactor score of the journals that published the SBCE content extracted for the review. These criteria were not used to include or exclude a publication. They are discussed as a means to comparatively assess the relevance, importance and visibility of SBCE research based on the metric.
Six articles (Terwiesch, Loch & Meyer Reference Terwiesch, Loch and Meyer2002; Ong et al. Reference Ong, Sun and Nee2003; Nahm & Ishikawa Reference Nahm and Ishikawa2006a,Reference Nahm and Ishikawab; Wasim et al. Reference Wasim, Shehab, Abdalla, Al-Ashaab, Sulowski and Alam2013; de Souza & Borsato Reference de Souza and Borsato2016) of the overall pool are published in journals with high Total Cites, (i.e., Organization Science, Journal of Material Processing Technology, Journal of Cleaner Production and the International Journal of Advanced Manufacturing Technology). In addition, a noticeable number of publications come from journals with relatively high Total Cites, which potentially indicates an increasing relevance of the research on SBCE and LPD. This, however, cannot be confirmed on the sole basis of the Total Cites as many other fields are published by the same journal and the Total Cites applies to all publications by the journal.
The average Impact Factor of the journals including the publications in the pool of relevant research is relatively low, which is quite representative of the field of design and product development research. However, Concurrent Engineering Research and Applications, which is the journal with most (11) of the 61 journal publications, is ranked 12th in the Computer, Database and Information Technology category. Similarly, Sloan Management Review is ranked 11th in the Management, Business, Decision Science and Finance category, while IEEE Engineering Management Review is in the top 100 of the same category (81st). Similarly, the International Journal of Advanced Manufacturing Technology is ranked 20th in the Mechanical, Production, Design, Automobile, Aeronautical and Industrial Engineering category. These results denote an extended breadth of SBCE research as it appears in several influential journals across different domains. It should be noted that the Impact Factor is an indicator of how many citations an article receives on average when published in a journal (Fersht Reference Fersht2009), which is in correlation with either the number of readers of the journal or the quality of the content or both. It is not possible to distinguish the impact of SBCE publications as compared to other publications of the same journal when it comes to the journal Impact Factor. However, it is possible to infer a potential visibility, importance and interest being given to SBCE research. The Eigenfactor Score similarly attempts to rate the influence of scientific journals by counting the number of citations a journal receives in a year and then weighing them based on the importance of the journal with the incoming citation (Bergstrom Reference Bergstrom2007). As seen in Table 2, the results are very similar to Total Cites, which is in line with the predicted strong correlation between Eigenfactor Scores and the total number of citations received by a journal (Fersht Reference Fersht2009). Therefore, conclusions similar to Total Cites apply.
Table 3. Category 5 SBCE publications

5 Results from content analysis
This section focuses on the 24 publications in category 5, which are those related to the development of SBCE theories, models and methodologies aimed at practical implementation. The publications are listed in Table 3. The table is sorted by year of publication.
The first part of the synthesis examines the relationships between these contributions by using the proposed classification framework. The last part discusses the contributions in more detail by following a succession of epochs centred on the formulation of the Second Toyota Paradox by Ward et al. (Reference Ward, Liker, Cristiano and Sobek1995).
5.1 Classification by research paradigms/methods
The chart in Figure 7 displays the distribution of research paradigms and methods related to the publications in the category of interest.
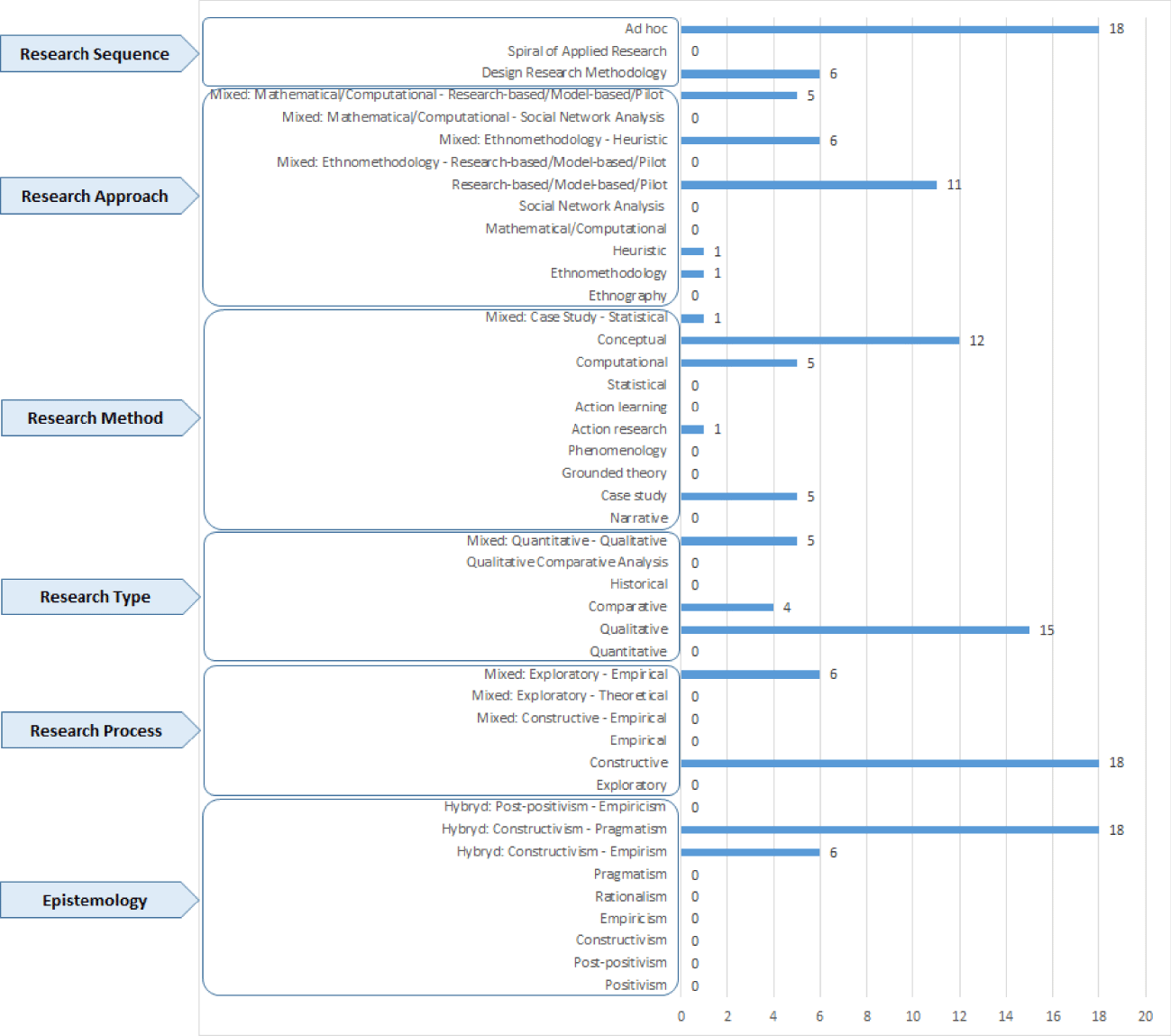
Figure 7. Distribution of the publications by research paradigms/methods.
The majority of publications in category 5 suggests a constructivist–pragmatist stance (75%), which denotes a field in active construction with common ground assumptions, principles and philosophy, shared and accepted within the community and believed to be further theorized through reasoning, experience and research. Knowledge in the SBCE and LPD community is viewed as a social construction (the community of SBCE and LPD researchers), and the foundational theories and principles are consistently rooted to the same landmark research effort, which in turn accounts for the rather constructivist–empiricist stance (25%) taken while studying Toyota and the Japanese automakers.
SBCE research is marked by enquiries into the interdisciplinary interactions within the design and product development process in context-specific settings. This is done in order to discover and understand the enablers and catalysts of the practice of SBCE. SBCE research is therefore predominantly qualitative and inductive, proceeding through natural enquiries. Data are expressed in the form of words rather than numbers, but quantitative analysis is often used to support experimental and computational methods, determine causality or to simply make sense of the data in order to generalize findings and extrapolate to similar situations. Qualitative coding (Strauss & Corbin Reference Strauss and Corbin1990) is frequently used to segment the data into categories that facilitate in-depth understanding, comparison and the development of the SBCE theory. Comparative research is applicable to the foundational research work that formulated the SBCE concepts through the benchmarking, study and comparison of the world automakers. These researchers mainly performed case studies, which allowed them to understand the ‘How’ and ‘Why’ from the natural settings of actual SBCE practitioners. Indeed, the formulation of the Second Toyota Paradox by Ward et al. (Reference Ward, Liker, Cristiano and Sobek1995) is a key milestone in SBCE research as it actually epitomized and coined the approach. Based on this evolution, there are necessarily themes and ideas that are not explicitly associated with these authors’ designation but which might disclose characteristics of the same and, therefore, would actually improve its understanding and implementation. The remaining portion of the publications is theoretical, conceptual and computational by nature, proceeding through research-based, model-based and pilot research to either generate a theory of SBCE or confirm/improve one constructed so far. There is one action research performed in civil engineering (construction industry), which also accounts for the one purely ethnomethodology-based approach reported in the chart. The benchmarking and comparative studies performed by the foundational research work is, by contrast, regarded as mixing ethnomethodology and heuristic. From a research sequence standpoint, the large part of the focused publications (75%) follows ad hoc sequences with respect to the scientific method. The remaining part displays a preference for the Design Research Methodology (DRM) by Blessing & Chakrabarti (Reference Blessing and Chakrabarti2009). This could reflect a recent influence of the DRM on the study of SBD at the atomic student project level rather than at a potentially larger integrated research programme level (count zero for the Spiral of Applied Research).
5.2 Classification by design process/methods
The chart in Figure 8 shows the distribution of the publications in category 5 by segments of the proposed classification framework.
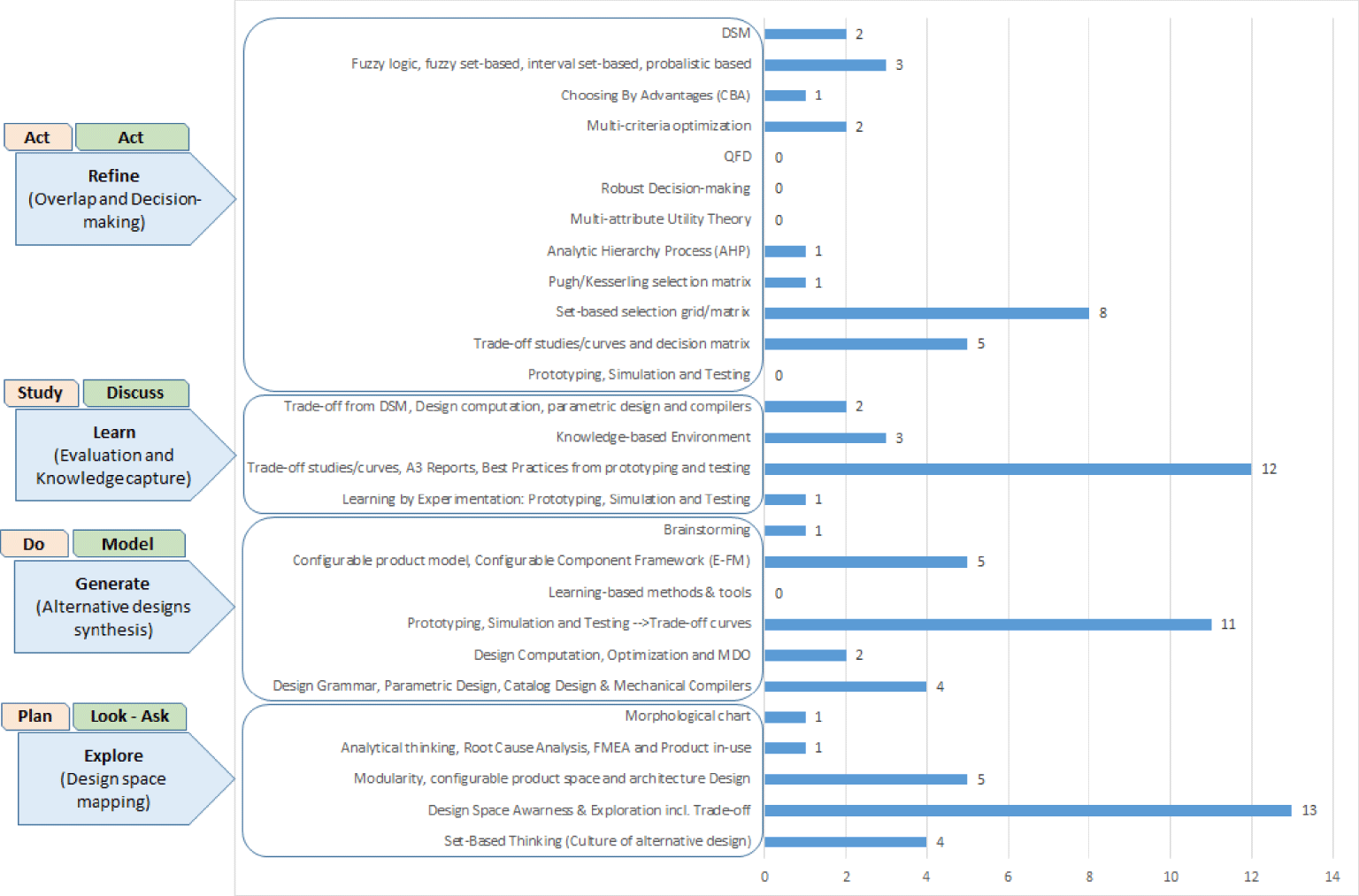
Figure 8. Distribution of the publications by design process/methods.
The initial phase of the SBCE process is sometimes achieved by merely considering multiple alternatives long into the design process and without resorting to any specific technique. This SBCE culture of alternative design exploration is a common practice in engineering design (Hubka & Eder Reference Hubka and Eder1987; Cross Reference Cross1989; Pugh Reference Pugh1991; Wheelwright & Clark Reference Wheelwright and Clark1992; Baxter Reference Baxter1995; Ullman Reference Ullman2009; Ulrich & Eppinger Reference Ulrich and Eppinger2012; Pahl & Beitz Reference Pahl and Beitz2013), and it does not necessarily distinguish SBCE from the other PD paradigms. In practice, it is the time and effort spent handling, exploring and documenting multiple alternatives long into the development process that makes SBCE different. Four of the 24 publications in category 5 simply discuss this vision and practice during the PD process (Liker et al. Reference Liker, Sobek Ii, Ward and Cristiano1996; Al-Ashaab et al. Reference Al-Ashaab, Golob, Attia, Khan, Parsons, Andino, Perez, Guzman, Onecha and Kesavamoorthy2013, Reference Al-Ashaab, Golob, Urrutia, Gourdin, Petritsch, Summers and El-Nounu2016; Ghosh & Seering Reference Ghosh and Seering2014).
Design space awareness and exploration extend the vision by enabling the broad discovery of the design space to avoid narrowing too quickly on discrete areas of the space and thereby losing the potential for innovation and discovery. Engineering design books and textbooks also advocate the practice using a variety of knowledge-based tools and techniques, but it appears that there is a focus on trade-off studies within the SBCE literature, particularly trade-off curves (Maksimovic et al. Reference Maksimovic, Al-Ashaab, Sulowski and Shehab2012; Araci et al. Reference Araci, Al-Ashaab and Maksimovic2016). This can be explained by a desire to explore and formalize Toyota’s practice of SBCE as described by the foundational literature on SBCE (Ward et al. Reference Ward, Liker, Sobek and Cristiano1994; Sobek & Ward Reference Sobek and Ward1996; Sobek Reference Sobek1996b). The majority $({\geqslant}60\%)$ of the publications in category 5 advocate exploring the design space, generating solutions and learning either by using computational tools (design grammars, parametric design, catalogue design and mechanical compilers) (Nahm & Ishikawa Reference Nahm and Ishikawa2006a,Reference Nahm and Ishikawab; Parrish et al. Reference Parrish, Wong, Tommelein and Stojadinovic2008b; Avigad & Moshaiov Reference Avigad and Moshaiov2010; Inoue et al. Reference Inoue, Nahm, Okawa and Ishikawa2010; Shahan & Seepersad Reference Shahan and Seepersad2010) or developing trade-off curves through prototyping, simulation and testing during one or more of the three phases (Ward et al. Reference Ward, Liker, Sobek and Cristiano1994, Reference Ward, Liker, Cristiano and Sobek1995; Liker et al. Reference Liker, Sobek Ii, Ward and Cristiano1996; Sobek Reference Sobek1996a,Reference Sobekb; Sobek et al. Reference Sobek, Ward and Liker1999; Khan et al. Reference Khan, Al-Ashaab, Doultsinou, Shehab, Ewers, Sulowski, Frey, Fukuda and Rock2011; Raudberget Reference Raudberget2011; Al-Ashaab et al. Reference Al-Ashaab, Golob, Attia, Khan, Parsons, Andino, Perez, Guzman, Onecha and Kesavamoorthy2013; Levandowski et al. Reference Levandowski, Forslund, Söderberg and Johannesson2013; Michaelis et al. Reference Michaelis, Levandowski and Johannesson2013; Levandowski et al. Reference Levandowski, Michaelis and Johannesson2014; Al-Ashaab et al. Reference Al-Ashaab, Golob, Urrutia, Gourdin, Petritsch, Summers and El-Nounu2016; Araci et al. Reference Araci, Al-Ashaab and Maksimovic2016).
From a product platform design standpoint, modularity, architecture design and the configurable product space (bandwidth) are also advocated within the SBCE literature, as valid means to explore the design space and generate viable alternative designs (Levandowski et al. Reference Levandowski, Forslund, Söderberg and Johannesson2013, Reference Levandowski, Michaelis and Johannesson2014, Reference Levandowski, Müller and Isaksson2016; Michaelis et al. Reference Michaelis, Levandowski and Johannesson2013; Raudberget et al. Reference Raudberget, Michaelis and Johannesson2014). The CC framework and Enhanced Function Means (E-FM) modelling by Johannesson & Claesson (Reference Johannesson and Claesson2005) are used there as the backbone to enable the approach during the product platform design.
The refinement phase of the SBCE process consists of overlapping alternative options from the disciplines and functional domains and making decisions to eliminate unfeasible designs. Overlapping the range options from many different views is generally perceived as complex and difficult to achieve, and researchers typically put forward trade-off studies, curves, generic decision matrices and set-based selection grids and matrices for communicating and eliminating alternatives (Sobek et al. Reference Sobek, Ward and Liker1999). The matrices use technical and other qualitative aspects/criteria of a design from different domains’ perspectives in order to weigh and assess the feasibility and performance of the design.
The next section will analyse the contributions on the development of SBCE theories, models and methodologies for SBCE included in category 5 in more detail. The discussion is organized in a succession of epochs presented to render the evolution of themes, ideas and experiences towards the formulation and refinement of the SBCE philosophy, principles, models and methodologies.
5.3 Before the Toyota Paradox
As previously mentioned, set-based reasoning and methods existed before the publication of the Second Toyota Paradox. These early 90s’ research efforts in engineering design calculations involved set-based reasoning by using fuzzy parameters, labelled interval calculus and other methods like the MoI and constraint satisfaction (Antonsson & Wood Reference Antonsson and Wood1989; Tommelein Reference Tommelein1989; Ward Reference Ward1989; Hyvönen Reference Hyvönen1992; Wood et al. Reference Wood, Otto and Antonsson1992; ElMaraghy & Jack Reference ElMaraghy and Jack1993; Hernandez-Luna & Wood Reference Hernandez-Luna and Wood1994). However, they did not entail the holistic SBCE philosophy, principles and the institutional knowledge capture and reuse advocated, enabling a superior PD process as encapsulated in the Second Toyota Paradox (Ward et al. Reference Ward, Liker, Cristiano and Sobek1995). In addition, these early research efforts involving set-based methods were not always nominally identified as such, which is the reason why some may not be retrieved by keyword searches with the term ‘set-based’. This area of publication as discussed here is discovered through reference backtracking with the purpose of understanding what set-based thinking was about before the Second Toyota Paradox and the origination of the term ‘set-based concurrent engineering’. For instance, Ward initially developed a theory of quantitative inference for artefact sets applied to design compilers, in particular, mechanical design compilers. The software construct used a schematic and high-level labelled interval specification language to allow a mechanical designer to search for an optimal design through a component database. The search then returned appropriate catalogue numbers of the granular components and progressively narrowed them until an overall cost function was fulfilled. The theory and the resulting software implementation represented and manipulated sets of artefacts at operating conditions rather than a single artefact under a single operating condition. Moreover, it performed searches by progressively narrowing volumes of the artefact space rather than searching point to point in that space. The theory and its implementation really correspond to SBD as it was later formulated by the author. Another example of set-based reasoning (applied to a design process before the Second Toyota Paradox) is the work done by Tommelein in structural engineering. Tommelein (Reference Tommelein1989) used a set-based methodology to generate adequate construction site layouts by reasoning about spatial constraints that need to be satisfied between rectangular sets in a layout. The approach was implemented in the SightPlan software, which is based on the Blackboard (BB1) architecture for combining geometry under constraints and displaying emerging solutions without committing to a specific one (Tommelein Reference Tommelein1989). From a similar perspective, Antonsson and Wood stressed uncertainty and the fuzzy nature of specifications and requirements during the design by formulating the design problem in terms of fuzzy parameters and constraints. In their method, they generate a range of possible design variable values (fuzzy sets of design) using the fuzzy set method of imprecision. This method resolves the design variable values by mapping the design preference onto the performance space while also quantifying imprecision (Antonsson & Wood Reference Antonsson and Wood1989; Wood et al. Reference Wood, Otto and Antonsson1992; Hernandez-Luna & Wood Reference Hernandez-Luna and Wood1994). Other methods preceding the Second Toyota Paradox include the use of Boolean equations (ElMaraghy & Jack Reference ElMaraghy and Jack1993) or interval arithmetic (Hyvönen Reference Hyvönen1992) to resolve design problems and potentially communicate between disciplines (e.g., design–manufacturing) via a range of parameter values rather than a single value at a time.
5.4 The Second Toyota Paradox
Set-based concurrent engineering, as it refers to Toyota’s practice, was coined by Ward et al. (Reference Ward, Liker, Sobek and Cristiano1994) in their landmark paper presented at the proceedings of the 1994 ASME Design Technical Conferences in Minneapolis, USA. The paper presents a new paradigm for concurrent or simultaneous design. The new paradigm is based on apparent paradoxes in Toyota Motor Company’s new product development strategies. Evidence from various interviews with the U.S. and Japanese automakers and automotive parts suppliers is presented, including two in-depth case studies. The paper concludes with a discussion of the potential benefits of this new paradigm. The paradigm was then extended in an article published the following year in the MIT Sloan Management Review (Ward et al. Reference Ward, Liker, Cristiano and Sobek1995). According to the authors, the First Toyota Paradox is Toyota’s lean production system as it involves unusual practices compared to traditional mass production. The article introduces the reader to the Second Toyota Paradox, which is basically the SBCE paradigm. The paradox is expressed by means of delaying decisions, communicating about the range of possibilities and pursuing apparently excessive numbers of prototypes. These elements taken together form the way by which Toyota is believed to design better cars faster and cheaper than its competitors. The authors explain that in their practice of SBCE, Toyota’s engineers ‘intuitively’ distinguish between cases requiring broad design space exploration as opposed to cases where a narrow, more constrained search is suitable; they later described how to make this determination formally based on the type of development project (Ward & Sobek Reference Ward and Sobek2014). The SBCE characteristics, techniques and advantages are explained, emphasizing a process that relies on handling and narrowing sets of possible solutions through design space exploration, prototyping, learning and commitment to robustness, all this by using effective management practices and the chief engineer concept. Many detailed examples of SBCE interactions among Toyota’s functional disciplines are discussed and also Toyota’s SBCE interactions with its suppliers. Examples of the joint practice of SBCE with key partner Nippon Denso are provided. The authors conclude with the prediction that ‘companies adopting concurrent engineering through cross-functional teams and a structured development process that focus on designing the right product in the concept stage will inevitably move in the direction of set-based concurrent engineering’ (Ward et al. Reference Ward, Liker, Cristiano and Sobek1995).
Sobek (Reference Sobek1996a) further advocates the SBCE process in a paper published in the Mechanical Engineer. The author argues that engineers can find better designs quicker by designing and developing sets of alternatives and then continually communicating about the available options rather than pursuing a single option at a time. SBCE is presented as the application of SBD to parallel development (in reference to CE, i.e., concurrent engineering), with five typical activities: global exploration, space expansion, parallel narrowing, conceptual robustness and pre-design. Many characteristics and advantages of SBD are also explained including the chief engineer role, prototyping and testing, individual and organizational learning, communication about sets of design and design spaces and commitment after establishing feasibility and global optima rather than independently optimized components.
In a search of evidence of SBCE, Liker et al. investigated 92 Japanese and 119 US automotive parts suppliers, with the purpose of determining the prevalence of set-based approaches in each group based on predefined indicators (Liker et al. Reference Liker, Sobek Ii, Ward and Cristiano1996). The outcome of the survey evidenced that SBD communication was more prevalent among Japanese suppliers than among their U.S. counterpart. Set-based CE is contrasted in the article with traditional CE by emphasizing the need for a paradigm shift in order to operate an effective, truly concurrent engineering. The SBCE paradigm is discussed in detail, explaining the difference with traditional CE when it comes to communicating requirements, exploring the solution space, communicating about designs (product or manufacturing process) by using ranges, gradually narrowing ranges, using parallel approaches, a large number of prototypes and by exploring trade-offs. The effect of the suppliers’ involvement early in the design process and the correlation with the use of set-based techniques is explored, concluding that there is a strong positive correlation between the two. Another correlation that is explored is the relationship between the use of set-based techniques and the degree to which product and process design overlaps, which surprisingly concludes on a weak correlation. Component interdependence and the use of Quality Function Deployment (QFD) are also considered set-based indicators, and they are equally explored in relation to the practice of SBCE among the groups. The two indicators display strong associations with SBD practices. The authors believe that the SBD philosophy has the potential to ‘provide high-bandwidth, trustworthy, and useful information creation and transmission’ processes. As such, these authors stress important implications of set-based CE, which include: (1) going slow early in the process and developing a large number of alternatives that allow for faster downstream design with less rework cycles; (2) developing new vocabularies for participants to communicate in sets; (3) switching organizational mindset to think in terms of narrowing sets rather than iterating on one single solution; (4) reconsidering the use of CAD systems early in the design process as they force commitment to many specific design decisions; and finally (5) potentially reducing the need for frequent face-to-face communication. Further research is recommended by the authors to develop indicators and metrics for point-based versus set-based design more carefully. The authors highlight the fact that the list of set-based indicators used in the survey is not exhaustive and that it should not be considered perfect either. According to the authors, the theories, the principles and basically what are the essential elements of SBCE should be better studied and formalized in order to perform accurate measures of the set-based culture of an organization.
Following the recommendations from Liker et al. (Reference Liker, Sobek Ii, Ward and Cristiano1996), Sobek et al. (Reference Sobek, Ward and Liker1999) published an article in the MIT Sloan Management Review in which they elaborated on Toyota’s principles of SBCE. The article describes the SBCE paradigm and its main characteristics as opposed to the traditional point-based approach. Three principles underlying SBCE are synthesized and presented as follows. (1) Map the design space. (Define feasible regions. Explore trade-offs by designing multiple alternatives. Communicate sets of possibilities.) (2) Integrate by intersection. (Look for intersections of feasible sets. Impose minimum constraint. Seek conceptual robustness.) (3) Establish feasibility before commitment. (Narrow sets gradually while increasing detail. Stay within sets once committed. Control by managing uncertainty at process gates.) The set-based communication and evaluation process is discussed using, for example, a matrix of alternatives including strengths and weaknesses with regard to the governing evaluation criteria. The convergence process is also presented focusing on a discipline groundwork (called Nemawashi), which for each discipline is about consistently finding the best solutions for the overall system under minimum constraints. The set-based selection process is explained in terms of narrowing sets gradually while increasing details but no formal approach, technique or tool is presented to support the overlapping of independent range solutions/alternatives that lead to discarding unfeasible designs. The SBCE principles combined with a culture of continuous creation of organizational knowledge appear to form the basis of the superior development system. It is argued that any organization that can implement these principles, as well as the related culture of organizational knowledge, may radically improve their design and development processes. Further study of the causality of the predicted outcomes/effects with regard to the synthesized SBCE principles is however prompted.
In essence, the authors who participated in formulating the Second Toyota Paradox collectively recommended further studies of the causal relationship between Toyota’s success and its practice of SBCE. The authors acknowledged that SBCE, as described, was the result of their perception of a system that is not explicitly documented or known to be well understood and performed in a systematic way. They, therefore, prompted researchers to construct the methodologies of SBCE and test them in other organizations in order to formulate a complete theory of SBD.
5.5 The post-Second Toyota Paradox publication
As a way to follow up on recommendations from the SBCE landmark research, the post-Second Toyota Paradox research efforts are usually performed outside the context of Toyota or any SBCE-proven Japanese entity, but at the same time, by quite systematically leveraging the philosophy, theories and principles developed while formulating the Second Toyota Paradox. This is to say, the SBCE philosophy, theories and principles as discussed above are collectively accepted, not reconsidered but rather studied and implemented in a constructivist–pragmatist way in order to confirm the premises or formulate a more complete theory of SBD. For example, although Liker et al. indicated that the use of CAD systems early in the design process should be reconsidered as they force commitment to many specific design decisions, Nahm, Ishikawa and Inoue (Nahm & Ishikawa Reference Nahm and Ishikawa2006a,Reference Nahm and Ishikawab; Inoue et al. Reference Inoue, Nahm, Okawa and Ishikawa2010) pragmatically leverage advances in CAD systems to propose a CAD-oriented SBD methodology that can support preliminary design activities and decisions. Indeed, the authors’ research work is motivated by the limitations of the traditional CAD systems in (1) supporting the conceptual design stage with its design information scarceness and uncertainty and (2) handling non-geometrical information, which is complementary and necessary design information required to fully define an engineering artefact as well as linking the CAD systems with other engineering systems and CE processes. These authors’ research is geared to provide a blend of methods and tools characterized by a parametric communication and robust design convergence process that use uncertain and incomplete information in support of the SBD paradigm. Their work combines SBD with the parametric modelling technique available in the geometric modelling kernels of most of the CAD systems. The combination produces a new concept called set-based parametric design (SBPD) for which a preference set-based design (PSD) model and a design information solid (DIS) model are proposed to ease the incorporation of the SBPD concept into the current CAD systems. Simply put, the DIS model augments the CAD data structure by adding non-geometrical design information as attribute data of the boundary representation (B-Reps) of the solid models (Nahm & Ishikawa Reference Nahm and Ishikawa2006a). It then becomes possible to store qualitative information such as functions, design intent, applicability, materials, context domain information, e.g., assemblability data, search index strings, etc. A designer working with a parametric solid model may populate the geometrical and non-geometrical parameter data and constraints, which will later be scanned by the PSD model when exploring the design space. The PSD model is a set-to-set mapping that propagates a designer preference sets (input) onto the available rich parametric solid models, given the constraints, so as to explore the interval sets and produce outputs under design information uncertainty (Nahm & Ishikawa Reference Nahm and Ishikawa2006a). The PSD is a hybrid model that combines the Antonsson & Wood (Reference Antonsson and Wood1989) fuzzy set-based MoI with the Finch & Ward (Reference Finch and Ward1997) interval set-based approach (which in turn is made up of the Quantified Relations (QRs) and the interval propagation theorem (IPT) algorithm). To exemplify their approach, Nahm and Ishikawa present a prototype system implementation that uses the PARASOLID CAD kernel to illustrate both the feasibility of the DIS model and the robustness of a PSD processor while propagating, aggregating, modifying and narrowing interval sets in order to infer solution sets (Nahm & Ishikawa Reference Nahm and Ishikawa2006a). The research work of Nahm, Ishikawa and Inoue is essentially motivated by the need to develop space representation methods, space mapping methods and space narrowing methods in support of the effective implementation of SBCE. These authors discuss a new space-oriented design methodology, which is based on interval sets and preference functions defined on the sets. The approaches used for processing the intervals include decomposed fuzzy arithmetic, extended interval arithmetic, i.e., IPT, Design of Experiment (DoE) and a new design metric called preference and robustness index (PRI). The combination of the approaches and metrics allows for a set-to-set mapping from a design space to a performance space and subsequently narrowing of the interval sets to eliminate unfeasible subspace solutions (Nahm & Ishikawa Reference Nahm and Ishikawa2006a,Reference Nahm and Ishikawab; Inoue et al. Reference Inoue, Nahm, Okawa and Ishikawa2010). Following their research, Nahm and Ishikawa recommend that future research should pay more attention to the data-level, interface-level, and application-level integration of heterogeneous 3D-CAD systems as well as the collaboration/interoperability required between CAx tools (Nahm & Ishikawa Reference Nahm and Ishikawa2006a). Some limitations regarding the proposed PSD model are also highlighted, which mainly consist of the inability of the model to produce correct output intervals for some combinations of input intervals. The limitation is thought to be caused by the direct use of IPT. Further research is required to allow checking the appropriateness of an input set in order to avoid the designer’s trial-and-error procedure, which may become painful while searching for a correct input interval.
Another noticeable post-Second Toyota Paradox research effort is the research done at Cranfield University by Al-Ashaab, Shehab, Khan, Araci, Maksimovic, et al. mostly under the FP7 Theme 4 of the 2009–2013 Lean Product and Process Development (LeanPPD) European research programme. These authors’ research is essentially motivated by the lack of formal SBCE methodology or process that can guide the systematic implementation of lean PD (Khan et al. Reference Khan, Al-Ashaab, Doultsinou, Shehab, Ewers, Sulowski, Frey, Fukuda and Rock2011; Khan Reference Khan2012; Al-Ashaab et al. Reference Al-Ashaab, Golob, Attia, Khan, Parsons, Andino, Perez, Guzman, Onecha and Kesavamoorthy2013, Reference Al-Ashaab, Golob, Urrutia, Gourdin, Petritsch, Summers and El-Nounu2016; Khan et al. Reference Khan, Al-Ashaab, Shehab, Haque, Ewers, Sorli and Sopelana2013; Araci et al. Reference Araci, Al-Ashaab and Maksimovic2016). In Khan et al. (Reference Khan, Al-Ashaab, Doultsinou, Shehab, Ewers, Sulowski, Frey, Fukuda and Rock2011), Khan (Reference Khan2012), SBCE is advocated as the keystone of lean PD, and five principles describing SBCE are synthesized from a literature review. These principles are similar to Sobek et al. (Reference Sobek, Ward and Liker1999) principles with the addition of Morgan, Liker and Ward principles for classifying development projects according to the company’s value strategy in relation to the customer value (Morgan & Liker Reference Morgan and Liker2006; Ward & Sobek Reference Ward and Sobek2014). A ‘set-based concurrent engineering baseline model’ is introduced by describing a stage-gate process through which alternative solutions are collected, narrowed into a set of solutions and converged onto an optimal solution before commencing the detailed design. Pugh matrices are used for the selection process. The model as presented and implemented is rather point-based, which limits its application for an SBD perspective. This is also pointed out by Raudberget (Reference Raudberget2015, p. 26), citing Al-Ashaab et al. (Reference Al-Ashaab, Golob, Attia, Khan, Parsons, Andino, Perez, Guzman, Onecha and Kesavamoorthy2013). In addition, there is no provision for a potential design or product model that could support the communication, narrowing, overlapping and refinement of sets. Furthermore, the iterative learning capability, which is central to SBCE and LPPD, is missing. However, Khan (Reference Khan2012) stresses the ability of the proposed model to enable SBCE and justifies the point-based outcomes of the thesis’s two pilot case studies by the inherent time and resource constraints in industrial product development projects. The constraints, in the case of the author’s pilot simulations, led to pressurized, non-informed and subjective decision-making (Khan Reference Khan2012, p. 184). In all cases, Khan et al. note that SBCE research should focus on developing models that can support the communication, narrowing, overlapping and refinement of sets and, furthermore, research should focus on enabling the iterative learning capability, which is central to SBCE and lean PD (Khan et al. Reference Khan, Al-Ashaab, Doultsinou, Shehab, Ewers, Sulowski, Frey, Fukuda and Rock2011, Reference Khan, Al-Ashaab, Shehab, Haque, Ewers, Sorli and Sopelana2013).
Drawing upon previous groundwork, Al-Ashaab et al. (Reference Al-Ashaab, Golob, Urrutia, Gourdin, Petritsch, Summers and El-Nounu2016) discuss the development of an LPD performance measurement tool to assess the ‘leanness’ of a PD process. In recognizing that SBCE is the core component of LPPD, the authors devise an assessment tool that relies on the enablers of a ‘LeanPPD’ model and the principles of SBCE, as synthesized from the literature by Khan et al. (Reference Khan, Al-Ashaab, Doultsinou, Shehab, Ewers, Sulowski, Frey, Fukuda and Rock2011, Reference Khan, Al-Ashaab, Shehab, Haque, Ewers, Sorli and Sopelana2013). The tool supports assessing a company’s practice of SBCE and LPPD from four perspectives (Al-Ashaab et al. Reference Al-Ashaab, Golob, Urrutia, Gourdin, Petritsch, Summers and El-Nounu2016): (1) product development process, i.e., directly referring to the practice of SBCE and the presence of the chief engineer and value focus enablers; (2) tools and enablers (as collected by Khan et al.) regarding lean and SBCE; (3) knowledge focus, which is key to lean, i.e., knowledge-based environment to support the capture, storage, retrieval, communication and the institutional learning as advocated by Ward & Sobek (Reference Ward and Sobek2014); and finally (4) continuous improvement as a means for the tool to both express an as-is state and then drive to a to-be state implementation roadmap. The authors propose an assessment scale named SAUCE (Start-Awareness-Unstructured-Continued-Evolved) to allow a visual representation of a company’s current ‘leanness’ state, which also is an incentive to devise an improvement plan to reach the next level. The scale is non-cyclic (with reference to continuous improvement tools like PDSA, DMAIC, LAMDA), but it supports improvement towards the desired state. Regardless, assessments are performed in two companies in the aerospace and the automotive industry, with the intent of demonstrating the ability of the measurement tool and the scale to provide insights into a company’s practice of lean PD and SBCE in a systematic way. Indeed, the research developed on a tool to assess the lean PD and SBCE practice of a company based on enablers in the ‘LeanPPD’ model, as well as principles of SBCE as synthesized from the literature. However, the research did not discuss approaches, methodologies or frameworks that can actually support the lean transformation within an organization. This is seen as research opportunities by the authors. Other publications by the same authors focus on more specific SBCE enablers by attempting to develop upon such techniques and practices. For example, Araci et al. (Reference Araci, Al-Ashaab and Maksimovic2016) concentrate on the use of trade-off curves (ToC) to enable SBCE and LPPD, by proposing a process for generating and leveraging knowledge-based and math-based ToCs at each stage of the ‘set-based concurrent engineering baseline model’ described by Khan (Reference Khan2012). While these authors deepen the understanding and applicability of a well-known SBCE enabler (Sobek et al. Reference Sobek, Ward and Liker1999; Ward & Sobek Reference Ward and Sobek2014), they rely on the SBCE baseline model that was previously criticized for its point-based approach (Raudberget Reference Raudberget2015, p. 26). In addition, it is unclear how sets of design are composed, overlapped, narrowed and eliminated by using ToCs from different functional groups. Maulana et al. (Reference Maulana, Flisiak, Al-Ashaab, Araci, Lasisz, Beg and Rehman2016) potentially fill this gap in their application of SBCE to enhance the design performance of a surface jet pump. These authors first develop conceptual sets by using ToCs, stakeholders’ expertise and brainstorming. Then they eliminate unfeasible sets by leveraging an Analytical Hierarchy Process (AHP) (Saaty Reference Saaty1990), which is fed by data gathered from prototyping and testing, i.e., CFD simulations and past project data. Al-Ashaab et al. (Reference Al-Ashaab, Golob, Attia, Khan, Parsons, Andino, Perez, Guzman, Onecha and Kesavamoorthy2013) also use AHP in the same vein. They describe a transformation process towards LPD in a company. The transformation consists of two stages whereby the first infuses the principles of SBCE (Khan et al. Reference Khan, Al-Ashaab, Shehab, Haque, Ewers, Sorli and Sopelana2013) into an existing product development process by introducing the relevant lean activities and tools (e.g., QFD, brainstorming, DoE, Pugh matrix, AHP, FMEA, risk analysis, etc.) in a timely manner. The second stage infers a generic model for structuring activities and tools for the desired type of transformation to achieve lean PD. This is applied to a research-based case study. Although the results can be viewed as linked to a particular development context, they provide some guidelines on how to restructure existing PD activities and appropriately use SBCE principles and tools to transition to a lean PD. This again is a blend of activities, tools and techniques that implements an approach or so-called SBCE process, which does not rely on the well-defined model of SBCE. That is, one that is in the continuum of a design process model, product model or knowledge-based model with the aim of federating the three Ps (People–Product–Process) towards SBCE and LPD in traditional industrial contexts.
A potentially advanced model and practical approach to SBCE can be found in the research work done at the Department of Product and Production Development of Chalmers University of Technology (Michaelis et al. Reference Michaelis, Levandowski and Johannesson2013; Levandowski Reference Levandowski2014; Raudberget Reference Raudberget2015). These authors leverage the CC framework and Enhanced Function Means (E-FM) modelling by Johannesson & Claesson (Reference Johannesson and Claesson2005) to enable SBCE during product platform design. The related manufacturing process design is also considered. Configurability is perceived and advocated as a fundamental means to handle multiple design alternatives, allow mapping, exploring the design space and gradually narrowing sets while preserving the design bandwidth, i.e., decision delay. The design bandwidth concept is equivalent to the viable, flexible solution sets that represent architectural options from which a coherent product design can be extracted, instantiated and passed onto downstream processes for further design and validation (Berglund & Claesson Reference Berglund and Claesson2005). E-FM modelling presents the advantage of spanning the functional (FRs) and technological/physical (DSs) domains, which allows for variability both at the functional requirement level and the logical system design level, i.e., functional feature. It is not always easy to understand the difference and interaction between the CC framework and the E-FM modelling. The CC framework is essentially meant to encapsulate a system level design variability and thereby bridge the gap between the technological domain (DSs) and the physical domain (Parts) where applicable. To achieve this, the CC framework is built upon configuration rules for Part selection from catalogues, which allows for combining variability in the technological and functional domains with variability in the physical domain. The purpose is to translate architectural options into various workable product variants. Indeed, an instantiation input (design parameters) is usually passed to the CC framework, which may then extract a specific physical design variant based on the system level architecture it has inherited from the E-FM tree (Johannesson & Claesson Reference Johannesson and Claesson2005). Johannesson and Claesson mention that the information technology necessary to deploy the framework is commercially available in Product Data Management (PDM) systems such as iMAN from EDS PLM Solutions, which is now part of the Siemens PLM software, i.e., Teamcenter. Indeed, the E-FM and CC framework show core similarities with product models usually deployed in industrial PLM software but they also display a good compatibility with SBCE. For instance, Michaelis, Levandowski and Raudberget leverage the combinatorial effects of variability and configurability within the E-FM and CC framework to advocate the fair compatibility with SBCE design space exploration. These authors use variability within the E-FM tree model to handle multiple architectural options that can then be analysed with the purpose of reducing functional design solution couplings, and then progressively eliminate unfeasible designs at the conceptual level (Michaelis et al. Reference Michaelis, Levandowski and Johannesson2013; Levandowski Reference Levandowski2014; Raudberget Reference Raudberget2015). This is also seen as extendable to manufacturing processes (Levandowski et al. Reference Levandowski, Michaelis and Johannesson2014; Johannesson & Claesson Reference Johannesson and Claesson2005). The framework allows for SBCE convergence processes because it supports (1) the exploration of a product platform design space as well as the elimination of unfeasible designs and (2) the preservation of conceptual solution sets until variants are instantiated through the CC framework for embodiment design and a reality check. Although the framework shows capabilities at the conceptual level, it remains unclear how the functional groups (i.e., requirements engineering, advanced engineering, systems engineering, components engineering, etc.) may interact using it. To cite Johannesson & Claesson (Reference Johannesson and Claesson2005), the corresponding development approach that relates to the framework and fully exploits it remains unclear, i.e., the complete development process. The lifecycle stages beyond conceptual design are rarely addressed, which results in less substance when it comes to the SBCE front-loading process, reusable design knowledge management, prototyping and testing for an informed convergence process, value streams’ cross-functional interactions and, basically, the remaining SBCE means by which a product platform can be developed in a large-scale industrial conventional PD framework and lean context. Levandowski et al. (Reference Levandowski, Forslund, Söderberg and Johannesson2013) attempt to fill these gaps by exploring an approach whereby product variants are instantiated from the CC modeller and then passed to a PLM software to engage downstream activities in the development process. Virtual prototyping and simulations are performed through the normal PLM process and then trade-off curves are produced to inform the E-FM/CC platform design by means of an analog feedback, which then helps the product platform design convergence process. This approach discloses dislocated methodologies and tools that may foster scatter, hand-offs and waste. The same approach and gaps are present in Johannesson et al. (Reference Johannesson, Landahl, Levandowski and Raudberget2017). Since product modelling and configurable product models span multiple product development stages and domains (i.e., functional, technological, physical processes) (Wortmann & Erens Reference Wortmann and Erens1995; Erens Reference Erens1996; Andreasen, Hansen & Mortensen Reference Andreasen, Hansen and Mortensen1996; Männistö et al. Reference Männistö, Peltonen, Soininen and Sulonen2001; Huet et al. Reference Huet, Fortin, McSorley and Toche2011; Toche et al. Reference Toche, Pellerin, Fortin, Huet, Rivest, Bouras and Louhichi2012), it is argued by Toche (Reference Toche2017) that their ability to enable SBCE can be explored from a holistic PD perspective and by using a continuum of tools and methodology as they pertain to large-scale industrial conventional PD supporting frameworks such as PDM (including configuration management) and PLM. Similarly, although Digital Mock-ups (DMU), Functional DMU (FDMU) and industrial DMU (iDMU) extensively support the conventional industrial PDP (Lazzari & Raimondo Reference Lazzari and Raimondo2001; Drieux Reference Drieux2006; Garbade & Dolezal Reference Garbade, Dolezal and Krause2007; Enge-Rosenblatt et al. Reference Enge-Rosenblatt, Clauß, Schneider and Schneider2011; Herlem et al. Reference Herlem, Adragna, Ducellier, Durupt, Rivest, Bouras and Louhichi2012; Mas et al. Reference Mas, Menendez, Oliva, Rios, Gomez and Olmos2014), the prototyping (virtual and physical) and testing (including simulations) phases are also rarely used as actual means to enable the SBCE overlapping and decision-making process. These are all aspects that require more evaluation in future research.
Other significant post-Second Toyota Paradox research includes contributions from Parrish et al., Kerga, Taisch and Terzi, Avigad and Moshaiov, as well as Shahan and Seepersad. Parrish et al. explored the benefits of implementing SBCE during the design of reinforced concrete structures (Parrish et al. Reference Parrish, Wong, Tommelein and Stojadinovic2008a; Parrish Reference Parrish2009). Variability is considered in these authors’ contributions. Alternative design solutions are explored by using Building Information Modelling (BIM), which is similar to PLM in many aspects (Jupp Reference Jupp2016). Parrish (Reference Parrish2009) emphasizes stakeholders’ interaction while communicating, postponing and deciding about sets of design. This author proposes a comparative study of a variety of decision-making processes/methods, ranging from Robust Decision-making (Ullman Reference Ullman2001), Multi-attribute Utility Theory (Thurston Reference Thurston1990), AHP (Saaty Reference Saaty1990) to Choosing By Advantages (CBA) (Suhr Reference Suhr1999), to name a few. Parrish in her dissertation thesis states that ‘literature cannot prescribe a set of steps to implement an SBD process since it is context-specific. It varies with the stakeholders involved, the design phase, the decision unit, and the project itself’ (Parrish Reference Parrish2009, p. 266). A manifestation of this can be found in Kerga et al. (Reference Kerga, Rossi, Taisch and Terzi2014a), where trade-off curves are perceived as detrimental to the set-based innovation process in serious games. The theory of inventive problem solving (TRIZ) is proposed as an improved methodological approach while implementing SBCE. However, the performance gained compared to the use of trade-off curves is not explained. From another perspective, Avigad & Moshaiov (Reference Avigad and Moshaiov2010) propose an approach to delay conceptual decisions under uncertainty while maintaining and assessing the performance of multiple design concepts. This is done by translating the design problem into a multi-objective problem and by mapping each of the design concepts to a cluster of points in the objective space, representing the discrete option performances (Avigad & Moshaiov Reference Avigad and Moshaiov2010). The approach requires abstracting the design problem into a design space tree (i.e., Complete Concept tree) and then an objective and variability space. The authors argue that when achieved properly, the approach may allow comparing design concepts based on their robustness, i.e., sensitivity to change according to the delayed decisions’ uncertainty. This approach is relevant to SBCE during the design conceptual phase. It requires several levels of abstraction and computations that are not easy to position in the context of an industrial SBCE implementation.
The remaining part of the post-Second Toyota Paradox research usually consists of confirmatory studies of the SBCE performance using computational simulations or pilot projects. For example, Shahan & Seepersad (Reference Shahan and Seepersad2010) use multi-criteria optimization and discrete event simulations to explore the effects of highly iterative exchanges of single design solutions (point-based) versus minimally iterative exchanges of multiple solutions (set-based) on the overall lead time of a design process. These authors conclude in favour of the reduced overall iteration cycles (hence shorter lead times) in SBCE as well as the robust convergence in the case of flexible, optimal size, rich and less frequent exchanges of information. These findings are similar to those by Beauregard et al. (Reference Beauregard, Bhuiyan and Thomson2014). They are also consistent with the findings by Ford & Sobek (Reference Ford and Sobek2005) with regard to their assessment of the development project performance in set-based versus point-based using real option valuation models. As their simulations were done on serialized processes, Shahan & Seepersad (Reference Shahan and Seepersad2010) point out the necessity to investigate concurrent processes in the context of available computer-aided engineering tools, product lifecycle management and knowledge-based engineering capabilities.
6 Conclusions and perspectives
A critical literature review of SBD was carried out in this article by following an evidence-based procedure to analyse the literature. The objective of the review was to find answers to the following questions:
(i) What is the state of the art of SBD literature?
(ii) What are the most important contributions to the SBD focusing on the development of a model and methodology?
(iii) What are the key aspects to consider when developing such a model and methodology?
A classification and review of the most relevant contributions pertaining to the objectives were performed. The discussion allowed us to discover and explore themes and ideas connected to the key aspects to consider when developing models and methodologies intended to support a transition to SBCE.
From a broad perspective, the relatively low theoretical development of SBD, combined with a steady increase of the diversity of contributions since 2010, suggests a growing visibility, importance and interest being given to SBD from a variety of research fields. This progression might be interpreted as a manifestation of the Ward et al. (Reference Ward, Liker, Cristiano and Sobek1995) prediction that ‘companies adopting concurrent engineering through cross-functional teams and a structured development process that focus on designing the right product in the concept stage will inevitably move in the direction of set-based concurrent engineering’.
From the synthesis, it is possible to articulate a number of findings and research opportunities. First, the authors who participated in formulating the Second Toyota Paradox collectively recommended further study of the causal relationship between Toyota’s success and its practice of SBCE. These authors acknowledged the fact that SBCE, as described, was the result of their perception of a system that was not explicitly documented or known to be well understood and performed in a systematic way. Therefore, they prompted researchers to construct the methodologies of SBCE and test them in other organizations in order to formulate a complete theory of SBD. It is also found from the synthesis that research is required to extend the application of SBD theories and principles beyond the conceptual design stage, especially implications for detailed design, prototyping, testing and the rest of the product development cycle. In more detail, the product development stages beyond conceptual design are rarely addressed within the SBD literature. Research is required to better address the SBCE front-loading process, the reusable design knowledge management, prototyping and testing (for an informed convergence process), the value streams’ cross-functional interactions and, basically, the means by which a product platform can be developed in a large-scale lean industry conventional PD framework by implementing SBCE. Indeed, SBD authors consistently agree on the lack of holistic models that can support the cross-domain communication, overlapping, narrowing, and refinement of sets to enable the iterative institutional learning capability that is core to SBCE and LPD. To continue with the findings, product structuring, configurability and variability emerged from the synthesis as effective SBCE enablers. However, they remain scarce within the SBD literature. Their ability to enable SBCE is not explored from a holistic PD perspective and by using a continuum of tools and methodology as they pertain to large-scale industrial conventional PD supporting frameworks. Another aspect of SBD that requires more study is the practice of prototyping and testing and the relationship with the overall design process. Extensive prototyping and testing is key to SBD in order to foster the knowledge-based environment, the institutional learning capability and, therefore, accurately inform the decision-making process. Although this is frequently portrayed in the literature, the existing industrial design prototyping (virtual and physical) frameworks are rarely connected with SBCE. More specifically, the effects of major SBCE enablers (e.g., product structuring, configurability, prototyping, set-based selection process etc.) on the development process performance are rarely studied, whether by experimenting alternative hypotheses or disproving the null hypothesis. New approaches are required to explore additional themes and ideas that are not explicitly associated with SBD but which share characteristics of the same and, therefore, have the potential to improve its understanding and implementation. Overall, the discovery and study of the key aspects to consider when developing a model and methodology to transition to SBCE should be extended by finding, and empirically assessing, the compatibility and confluence between the themes. This is the subject of ongoing research by the authors.