For many years, the presence of bilateral infundibulums, or conuses, was considered an essential part of the diagnosis of double-outlet right ventricle.Reference Van Praagh, Davidoff, Chin, Shiel, Reynolds and Van Praagh 1 Part of the justification for this suggested criterion, at the time, was to permit the distinction of tetralogy of Fallot from double-outlet right ventricle with subaortic septal defect.Reference Baron 2 There was, however, no historical justification for this, as Fallot, in his initial account of the three hearts forming the basis of his tetralogy, described how the aorta, along with the pulmonary trunk, arose exclusively from the right ventricle in one of them.Reference Fallot 3 Continued insistence on the presence of bilateral infundibulums to justify the diagnosis of double outlet, furthermore, transgresses the important principle of anatomical analysis now known as the morphological method.Reference Van Praagh, David, Wright and Van Praagh 4 This concept, introduced initially to show that presence of an inlet component was not an essential feature of an incomplete ventricle, stated justifiably that a given anatomical entity should not be diagnosed on the basis of another feature that is itself variable. The principle is equally valid in the setting of double-outlet right ventricle, as the latter entity is one particular type of ventriculo-arterial connection, rather than a specific anatomical phenotype.Reference Wilcox, Ho, Macartney, Becker, Gerlis and Anderson 5 It is the phenotypic anatomy that underscores the variability to be found in the hearts unified because of the ventriculo-arterial connection. Of the multiple variable anatomical features, perhaps the most important is the location of the interventricular communication. This feature plays the predominant role in determining the haemodynamic features, and largely determines the surgical options for repair.Reference Walters, Mavroudis, Tchervenkov, Jacobs, Lacour-Gayet and Jacobs 6 There is general agreement that the anatomical location of this channel between the ventricles is best described, as initially suggested by Lev et al,Reference Lev, Bharati, Meng, Liberthson, Paul and Idriss 7 as being subaortic, subpulmonary, doubly committed, or non-committed. In this respect, the investigators had determined commitment on the basis of the relationship between the communication itself and the ventricular outflow tracts. Agreement has still to be reached, however, with regard to the precise criteria to be used to determine such commitment. Thus far, discussions have focussed on when the defect should be considered non-committed. In this regard, defects that seem to be non-committed in anatomical terms can be tunnelled to one or other ventricular outlet in the operating room, meaning that they are surgically committed.Reference Lacour-Gayet, Haun and Ntalakoura 8 , Reference Devaney, Lee and Gelehrter 9 Problems also exist with the allegedly doubly committed defect, as it is usually assumed that the feature of this variant is absence, or gross hypoplasia, of the outlet septum. We have now encountered several hearts that call this presumption into question, such as those with a fibrous outlet septum, but in the setting of overtly non-committed defects. In other hearts, we have observed attachments of a fibrous outlet septum that place the interventricular defect in a subaortic rather than a doubly committed position, whereas we have observed yet other hearts with an extensive muscular outlet septum in the setting of doubly committed defects. In this review, we describe these variants, arguing that it is the insertions and location of the outlet septum that determine commitment of the interventricular communication to the arterial roots, irrespective of whether the outlet septum itself is fibrous, muscular, or absent. We supplement our anatomical descriptions with a review of development,Reference Anderson, Mohun and Spicer 10 , Reference Sizarov, Lamers, Mohun, Brown, Anderson and Moorman 11 which provides additional evidence demonstrating the independence of the building blocks of the outflow tracts.
Heart with non-committed defect and fibrous outlet septum
The heart showing this arrangement to best advantage had usual atrial arrangement, but with discordant atrioventricular connections, and a common atrioventricular junction. As expected in the setting of discordant atrioventricular connections, there was left-handed ventricular topology. The morphologically right ventricle was left-sided, receiving the morphologically right component of the common atrioventricular junction (Fig 1a). Both arterial trunks arose exclusively from the left-sided morphologically right ventricle, with the leaflets of their arterial valves separated from the leaflets of the common atrioventricular valve by the muscular ventriculo-infundibular fold, the latter structure representing the inner heart curvature. The infundibulums themselves, however, were incomplete. The adjacent leaflets of the aortic and pulmonary valves were in fibrous continuity, with minimal formation of a fibrous outlet septum (Fig 1b). The aorta itself was larger than the pulmonary trunk, and ascended into the mediastinum in anterior position.
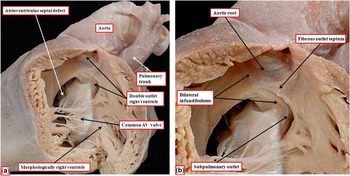
Figure 1 ( a ) The windowed left-sided morphologically right ventricle from a heart with usual atrial arrangement, discordant atrioventricular connections, and double-outlet right ventricle. There is an atrioventricular septal defect with a common atrioventricular junction. ( b ) The enlarged outflow tracts, revealing the presence of bilateral infundibulums, but with a fibrous outlet septum, such that neither arterial valve has complete muscular support. AV=atrioventricular.
Heart with doubly committed defect and fibrous outlet septum
When the ventriculo-arterial connections are concordant or discordant, and the outlet septum is fibrous rather than muscular, the hypoplastic nature of the outlet septum typically places a co-existing ventricular septal defect in a doubly committed and juxta-arterial position. In such situations, the fibrous outlet septum is aligned in a parallel manner relative to the crest of the muscular interventricular septum (Fig 2). On the basis of the morphology of the ventricular septal defect in hearts with such concordant or discordant ventriculio-arterial connections, it is generally presumed at present, when there is double-outlet right ventricle, that the absence of the outlet septum, or its fibrous rather than muscular structure, will again place the channel between the ventricles in a doubly committed position. This can, indeed, be the case. When such defects are truly doubly committed, however, the fibrous outlet septum is aligned at right angles to the crest of the muscular ventricular septum, and attaches to the midpoint of the ventriculo-infundibular fold, which represents the inner heart curvature (Fig 3). With this arrangement, the interventricular communication, opening to the right ventricle between the limbs of the septomarginal trabeculation, or septal band, is unequivocally doubly committed. As the outlet septum is inserted to the midpoint of the inner heart curvature, however, neither of the arterial roots is anchored to one or the other ventricle. This provides the opportunity for both roots to override the crest of the muscular ventricular septum, as is shown in another heart with a doubly committed interventricular communication, but with parallel rather than spiralling arterial trunks (Fig 4a). The arrangement has been described as double-outlet both ventricles. The specimen also serves to show the problems that exist in determining the precise area to be defined as the “ventricular septal defect” (Fig 4b).Reference Anderson, Spicer, Henry, Rigsby, Hlavacek and Mohun 12
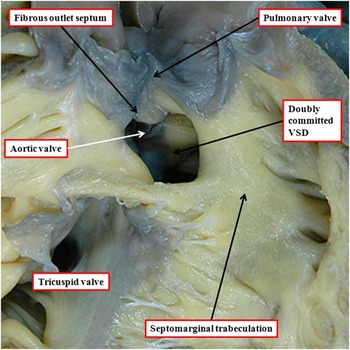
Figure 2 The image shows a doubly committed and juxta-arterial ventricular septal defect (VSD) in a heart with concordant ventriculo-arterial connections. There is a muscular postero-inferior rim to the defect. The roof of the defect is formed by the fibrous outlet septum, which is aligned in a parallel manner relative to the crest of the ventricular septum, which is reinforced by the limbs of the septomarginal trabeculation, or septal band.
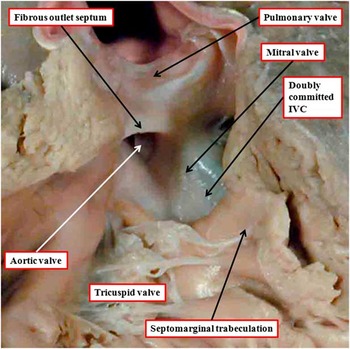
Figure 3 The image shows the outflow tracts from a heart with concordant atrioventricular connections and double-outlet right ventricle with doubly committed interventricular communication (IVC). Both arterial valves are supported predominantly by the right ventricle, but the leaflets of both valves are in fibrous continuity with the mitral valve in the roof of the interventricular communication. The aortic root is posterior and rightward relative to the pulmonary root – “normal relations”. The outlet septum is fibrous, and is aligned at right angles to the crest of the muscular ventricular septum, which is reinforced by the limbs of the septomarginal trabeculation. The fibrous outlet septum inserts to the midpoint of the fibrous inner heart curvature (compare with Fig 2).

Figure 4 The images are from another heart with concordant atrioventricular connections and double-outlet right ventricle with doubly committed interventricular communication (IVC), again with a fibrous outlet septum, but in this instance with parallel arterial trunks, and with the aortic valve positioned anterior and to the right of the pulmonary valve. ( a ) The view from the apex of the right ventricle, revealing that both arterial valves are supported predominantly by the right ventricle. ( b ) A frontal section through the heart, showing the different areas within the cone of space subtended from the bilateral infundibulums (double-headed, dashed red arrow) to the crest of the muscular ventricular septum (white star with red borders). The inner heart curve, or the ventriculo-infundibular fold, in this heart is muscular, separating the leaflets of both arterial valves from those of the atrioventricular valve. The yellow, double-headed arrow shows the outflow from the left ventricle. The white, double-headed arrow is the area that would potentially produce biventricular repair. The pulmonary outflow tract, however, is restrictive. The green, double-headed arrow shows how a patch could be placed so as to connect the left ventricle with the aortic root.
Heart with doubly committed defect and muscular outlet septum
The heart shown in Figure 5a is from a patient with usual atrial arrangement and concordant atrioventricular connections. Both arterial trunks arise exclusively from the right-sided morphologically right ventricle. The muscular ventriculo-infundibular fold, or inner heart curvature, separates the leaflets of both arterial valves from those of the atrioventricular valves. The interventricular communication, as viewed from the right ventricle, is located between the limbs of the septomarginal trabeculation. The muscular outlet septum, however, is extensive, and inserts to the midpoint of the inner heart curvature, thus potentially committing the defect to both arterial roots (Fig 5a). In Figure 5b, we show another comparable heart with an obviously doubly committed defect, but again with an extensive muscular outlet septum inserted into the midpoint of the inner heart curvature. In the second heart, however, the inner curve is fibrous rather than muscular, being formed by continuity between the leaflets of the arterial and mitral valves (Fig 5b).
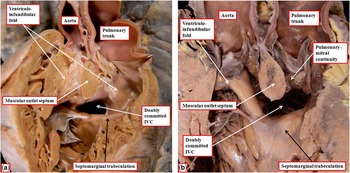
Figure 5 The images show two hearts with concordant atrioventricular connections and double-outlet right ventricle with doubly committed defects. In these hearts, unlike the heart shown in Figure 3, there is an extensive muscular outlet septum separating the arterial roots. The interventricular communication (IVC), nonetheless, opens to the right ventricle between the limbs of the septomarginal trabeculation. The insertion of the muscular outlet septum to the midpoint of the inner heart curvature, as was the case in the heart shown in Figure 3, places the defect in doubly committed position. In the heart shown in a , there are bilateral infundibulums, whereas in the heart shown in b there is fibrous continuity between the leaflets of the pulmonary and mitral valves.
Heart with subaortic defect and fibrous outlet septum
It is usually considered, at present, that the mere presence of a fibrous outlet septum in the setting of double-outlet right ventricle is sufficient to commit the interventricular communication, cradled within the limbs of the septomarginal trabeculation, to both arterial roots. This is not always the case. The heart shown in Figure 6a is from another patient with usual atrial arrangement and concordant atrioventricular connections, and with the interventricular communication, as anticipated, cradled between the limbs of the septomarginal trabeculation. The attachment of the fibrous outlet septum to the cranial extent of the superior limb of the septomarginal trabeculation places the entirety of the septal defect in the subaortic position. When the outlet septum in this location is muscular rather than fibrous, the defect is described as being subaortic (Fig 6b). In both instances, the outlet septum is aligned at approximately a right angle relative to the crest of the muscular interventricular septum (compare Fig 6a and b). In another heart, again with double-outlet right ventricle and bilateral infundibulums, there is gross hypoplasia of the muscular outlet septum, yet the defect, although part of an atrioventricular septal defect, is also subaortic (Fig 7). The heart with the common atrioventricular junction, therefore, serves to show the spectrum that can be found with regard to the dimensions and muscularisation of the outlet septum. It also points to the problems in determining commitment of the interventricular communication.
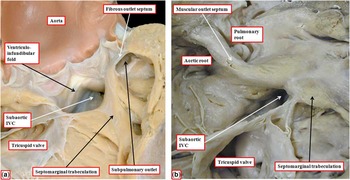
Figure 6 The images show two hearts with concordant atrioventricular connections and double-outlet right ventricle. ( a ) The outlet septum, which is muscular, is inserted to the anterior limb of the septomarginal trabeculation, committing the entirety of the interventricular communication (IVC) to the aortic root. The heart shown in b has a muscular outlet septum, but again its attachment to the anterior limb of the septomarginal trabeculation commits the interventricular communication to the aortic root. Both hearts have discontinuity between leaflets of the arterial and atrioventricular valves, but the presence of the fibrous outlet septum in a means that both infundibulums are incomplete, with fibrous continuity between the leaflets of the arterial valves through the fibrous outlet septum.

Figure 7 The image shows a heart with concordant atrioventricular connections with the double-outlet right ventricle in the setting of an atrioventricular septal defect and a common atrioventricular junction. The ventricular component of the defect is also subaortic. There are bilateral infundibulums, but with a hypoplastic outlet septum attached to the cranial limb of the septomarginal trabeculation.
Developmental considerations
When first formed, the outflow tract of the developing heart, which is now known to be derived from the second heart field, extends from the outlet of the distal component of the ventricular loop to the margins of the pericardial cavity.Reference Anderson, Mohun and Spicer 10 , Reference Sizarov, Lamers, Mohun, Brown, Anderson and Moorman 11 At this early stage, which is seen at Carnegie stage 12 in humans,Reference Sizarov, Lamers, Mohun, Brown, Anderson and Moorman 11 equivalent to embryonic day 10.5 in the mouse, the outflow tract has walls that are exclusively myocardial. When considered in terms of its shape, it is possible to recognise proximal, intermediate, and distal components of this muscular tube, which when first formed has a solitary lumen. With ongoing development, the walls of the distal part of the outflow tract are replaced by non-myocardial tissues. Its cavity is then divided distally by intrapericardial growth, in oblique fashion, of a spur of tissue from the dorsal wall of the aortic sac, thus producing separate aortic and pulmonary channels (Fig 8a).
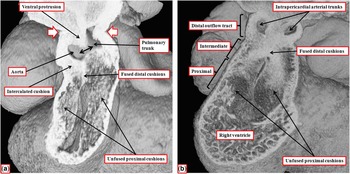
Figure 8 The images show the stages of separation of the outflow tract into the aortic and pulmonary channels that occur during the 12th day (E11.5) of development in the mouse. ( a ) The stage at which a protrusion has grown intrapericardially from the dorsal wall of the aortic sac, dividing the distal part of the outflow tract into the aortic and pulmonary channels. The white arrows with red borders show the margins of the pericardial cavity, with the double-headed black arrow showing the embryonic aortopulmonary foramen existing at this stage between the leading edge of the protrusion and the distal margins of the outflow cushions. The cushions themselves occupy the intermediate and proximal parts of the outflow tract. ( b ) The situation at the end of E11.5, with the intrapericardial arterial trunks now separated due to fusion of the protrusion with the distal ends of the outflow cushions, which have themselves now fused distally, although remaining unfused proximally. The fused components of the cushions are filled by columns of condensed mesenchyme, produced by cells that have migrated from the neural crest, with the columns also extending into the proximal initially unfused components of the cushions.Reference Anderson, Mohun and Spicer 10 , Reference Sizarov, Lamers, Mohun, Brown, Anderson and Moorman 11
By the time the protrusion has grown to separate the distal intrapericardial arterial channels, the jelly that initially lay in a circumferential manner between the endocardial and myocardial layers of the outflow tract has been populated with cells. The cells are from two sources – on the one hand migration from the neural crest and on the other hand by delamination from the endocardium by endothelial-to-mesenchymal proliferation. These processes produce cushions that face each other as they spiral throughout the intermediate and proximal parts of the outflow tract (Fig 8a). By the end of embryonic day 11 in the mouse, the distal part of the outflow tract is divided completely into the intrapericardial components of the aorta and pulmonary trunk by fusion of the protrusion from the dorsal wall of the aortic sac, which represents the aortopulmonary septum, with the distal ends of the outflow cushions (Fig 8b). The major cushions, which now occupy the intermediate and proximal parts of the outflow, have themselves now fused distally. In fact, the interdigitations of their margins with the intercalated outflow cushions, which by now have appeared at the junction between the distal and intermediate components of the outflow tract, set the stage for the formation of the arterial roots (Fig 9a). At this stage, both the newly separated arterial roots still take their origin from the distal part of the ventricular loop, by this time recognisable as the developing right ventricle, although the proximal components of the outflow cushions have yet to fuse (Fig 9b). At this point of development, therefore, the embryo can be considered to demonstrate double-outlet right ventricle. The aortic root, now separated from the pulmonary root, is posterior and right-sided (Fig 9a), and is positioned directly cranial to the embryonic interventricular communication (Fig 9b).
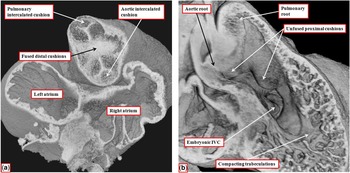
Figure 9 The images show the stages occurring during embryonic days 11 and 12 in the mouse heart that set the scene for formation of the arterial valves and the subpulmonary infundibulum. ( a ) A short-axis cut, viewed from above, through the intermediate part of the outflow tract from the data set shown in Figure 8b. The interdigitations between the fused distal cushions and the intercalated cushions produce the primordiums of the developing arterial valves. ( b ) A view of the developing right ventricle during E12.5. Both arterial roots are supported by the right ventricle. The proximal cushions remain unfused. The trabecular component of the ventricular wall is beginning to compact to produce the tension apparatus of the tricuspid valve and the septomarginal trabeculation. The embryonic interventricular communication (IVC), at this stage, is doubly committed, being positioned beneath both arterial roots. As seen in a , however, the developing aortic root is already posterior and to the right of the pulmonary root.
The two arterial roots themselves, at this stage of their development, remain enclosed within a turret of outflow tract myocardium. The dorsal walls of the proximal part of this turret, furthermore, are continuous with the musculature of the atrioventricular canal at the inner heart curvature. The next stage of development involves fusion of the proximal parts of the outflow cushions, along with transfer of the aortic root to the left ventricle. When these cushions first begin their process of fusion, they form an embryonic septum between the subaortic and subpulmonary outflow tracts, but with the subaortic outflow tract supported by the right ventricle, and walled dorsally by the muscular inner heart curvature interposing between the developing arterial and atrioventricular valves (Fig 10a). The process of transfer of the newly separated aortic root, along with its supporting proximal outflow tract, to the developing left ventricle also serves to bring the proximal margins of the fused cushions in line with the crest of the muscular ventricular septum. This, in turn, reduces markedly the size of the persisting interventricular communication. It is only subsequent to the transfer of the aortic root to the left ventricle, furthermore, that the interventricular communication is closed, with this process achieved by growth of tissue from the rightward and ventricular margins of the atrioventricular cushions (Fig 10b). In time, these contributions from the atrioventricular cushions will become the membranous part of the septum, better seen in the human heart than in the mouse. During these changes, the crest of the muscular ventricular septum is reinforced by compacting right ventricular trabeculations, which can then be recognised as the septomarginal trabeculation, along with its septoparietal extensions. After the commitment of the aortic root to the left ventricle, the core of the fused proximal cushion mass will be converted into extracavitary fibroadipose tissues. These tissues will then interpose between the aortic root and the subpulmonary infundibular sleeve, the latter having been formed by muscularisation of the surface of the cushions (Fig 11).
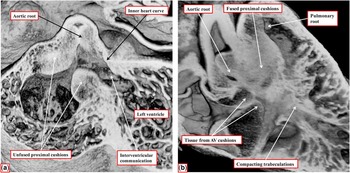
Figure 10 The images, prepared from episcopic data sets from mice sacrificed early ( a ) and late ( b ) during the 13th day of development, show the changes that take place during transfer of the subaortic outlet to the left ventricle. As seen in a , sectioned in “four chamber” plane, before fusion of the proximal outflow cushions, the aortic root remains supported by the developing left ventricle, with the myocardial inner heart curvature forming the cranial margin of the interventricular communication. ( b ) The arrangement as seen from the right side subsequent to fusion of the proximal cushions, which are now in line with the crest of the muscular ventricular septum. Expansion of the so-called “tubercles” from the ventricular surfaces of the atrioventricular cushions are closing; they are now representing the interventricular communication, thus walling the aortic root into the left ventricle. Note the compacting right ventricular trabeculations, which will form the septomarginal trabeculation. AV=atrioventricular.
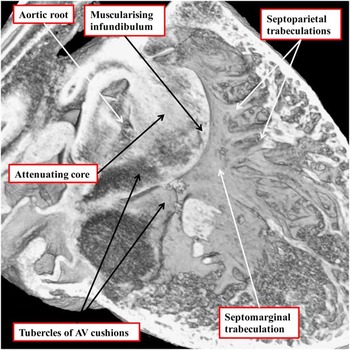
Figure 11 The image, taken from an episcopic data set prepared from a developing mouse embryo sacrificed at E14.5, shows the formation of the subpulmonary infundibulum from the proximal outflow cushions. The right ventricular surface of the fused proximal cushions is muscularising. Attenuation of the core of the cushions, likely by a process of cell death, will produce the extracavitary space that will separate the newly formed infundibulum from the aortic root. The right ventricular trabeculations can now be recognised as the septomarginal trabeculation and its septoparietal extensions. AV=atrioventricular.
In the normal heart, after closure of the embryonic interventricular communication, it is no longer possible to recognise any septal tissues between the newly formed ventricular outflow tracts. Instead, the entirety of the now muscularised surface of the proximal outflow cushions becomes converted into the dorsal aspect of the free-standing muscular infundibular sleeve. Even after the aortic root has been transferred to the left ventricle at embryonic day 14, nonetheless, the inner heart curvature remains myocardial, serving to separate the developing leaflets of the aortic and mitral valves (Fig 12a). Only later in gestation, at embryonic day 16 in the mouse, does the inner curvature become converted into mesenchymal tissue, eventually developing into the region of aortic-to-mitral valvar fibrous ontinuity (Fig 12b).
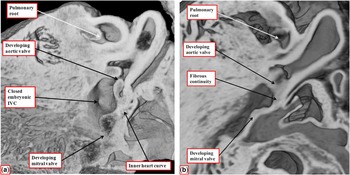
Figure 12 The images are from episcopic data sets prepared from developing mouse embryos at embryonic day 14.5 ( a ) and 16.5 ( b ). Both are cut to show the parasternal long-axis-equivalent section of the left ventricular outflow tract. ( a ) The site of the closed embryonic interventricular communication (IVC), with the aortic root fully committed to the left ventricle. The inner heart curvature is still myocardial at this stage, interposing between the leaflets of the developing aortic and mitral valves. As shown in b , it is not until embryonic day 16.5 that the myocardial tissues get converted into fibrous tissue.
We have also had the opportunity to study developmental defects involving the outflow tracts in genetically modified mice in which the Furin gene, which encodes an enzyme that activates several growth factor signalling proteins, had been conditionally perturbed in two different regions of the embryo, specifically those expressing either Islet-1 or Nkx 2.5genes. We examined the embryos at embryonic day 14.5, at which time, during normal development, the aortic root had been transferred to the left ventricle and the embryonic interventricular communication had been closed. We found various malformations including atrial and ventricular septal defects, common arterial trunk, and common atrioventricular junction. In several mice, however, we observed examples of double-outlet right ventricle. Significantly, the effects of perturbing the influence of the enzyme with the different Cre drivers showed how the fused proximal cushions could muscularise or remain hypoplastic. When the enzyme was perturbed under the influence of the Nkx 2.5-Cre, the arrangement was that of “classical” double-outlet right ventricle with subaortic interventricular communication and bilateral infundibulums (Fig 13).
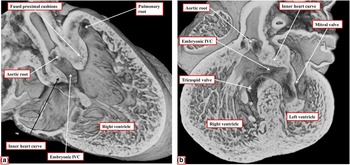
Figure 13 The images are taken from an episcopic data set prepared from a mouse embryo sacrificed at E14.5 subsequent to perturbation of the Furin enzyme using the Nkx 2.5 gene. As is seen in a , there is a double outlet from the right ventricle with a subaortic interventricular communication (IVC), due to attachment of the muscularising proximal outflow cushions antero-cephalad relative to the embryonic interventricular communication. The section taken at right angles to the image shown in a , seen in b , reveals the presence of the extensive muscular inner heart curvature interposed between the developing leaflets of the arterial and atrioventricular valves.
When, in contrast, the Furin enzyme was perturbed under the influence of the Isl1-Cre driver, the proximal outflow cushions, although fused, were grossly hypoplastic. In one of the mice, however, the fused outflow cushions inserted to the middle of the myocardial inner heart curvature, placing the embryonic interventricular communication in doubly committed position (Fig 14a). In the other embryo, in contrast, the hypoplastic cushions fused to the cranial margin of the interventricular communication, placing it in subaortic position as in the embryo with the muscularised outflow cushions (compare Figs 13a and 14a).
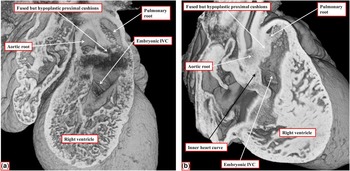
Figure 14 The images are taken from two data sets, again from mice sacrificed at E14.5 with ablation of the Furin gene under the influence of the Islet-1 Cre driver. In both embryos, the proximal outflow cushions are hypoplastic and have failed to muscularise (compare to Fig 13a, in which the cushions have muscularised). In the embryo shown in a , however, the fused outflow cushions are attached to the mid-portion of the still myocardial inner heart curvature, placing the interventricular communication (IVC) in a doubly committed position. In the embryo shown in b , in contrast, the hypoplastic cushions are attached antero-cranial to the defect, as in the embryo shown in Figure 13a, placing the defect in the subaortic location.
Discussion
The evidence from the various hearts illustrated in our review points to the independence of the building blocks of the ventricular outflow tracts, with this concept endorsed by the evidence provided by our examination of cardiac development in the mouse.Reference Anderson, Mohun and Spicer 10 The developmental findings show that the subvalvar ventricular outflow tracts are derived from the proximal component of the developing outflow tract. It is the posterior, or dorsal, part of this myocardial tube, together with the anterior or ventral wall of the atrioventricular canal, which forms the inner heart curvature. As the atrioventricular valves develop within the atrioventricular canal, and the arterial valves within the intermediate component of the outflow tract, it follows that this proximal part of the outflow tract interposes between the hinges of the developing valvar leaflets. The inner curvature, significantly, is still myocardial subsequent to closure of the embryonic interventricular communication (Fig 12a). Only at embryonic day 16.5 in the mouse, which is just before birth, does the fold become non-myocardial. It is persistence of this myocardial inner heart curvature between the leaflets of the atrioventricular and arterial valves that produces the so-called bilateral “conuses”. The septal component of the conuses, or infundibulums, in contrast, is formed by muscularisation of the proximal outflow cushions, which initially divide the proximal outflow tract into its aortic and pulmonary components.Reference Sizarov, Lamers, Mohun, Brown, Anderson and Moorman 11 If development has proceeded normally, this muscularising tissue mass will become converted into the subpulmonary infundibulum. In this setting, the central core of the initial cushion mass is transformed into the extracavitary tissues that interpose between the newly formed subpulmonary infundibular sleeve and the aortic root. In the normal heart, therefore, there is no formation of a muscular “outlet septum”.Reference Mori, Spicer and Anderson 13 As we now show, it is when the aortic root is not fully transferred to the left ventricle or when the embryonic interventricular communication fails to close that the proximal cushion mass is able to muscularise and retain its septal identity. We also show, nonetheless, that this process is independent of the fate of the inner heart curvature. Our observations also show that the development of these components of the developing outflow tract – namely, the inner heart curvature and the embryonic outlet septum – are themselves independent of the formation of the septomarginal trabeculation or septal band. The latter structure, along with the septoparietal trabeculations, and the tension apparatus of the tricuspid valve, is derived by compaction of the right ventricular trabeculations. These structures belong to the apical component of the ventricle rather than to the outflow tract.
All of these observations from the developing heart are pertinent to the classification and nomenclature of double-outlet right ventricle. It is now becoming accepted that persistence of the inner heart curvature as a myocardial entity, or in other words the presence of bilateral conuses, is no longer necessary for the definition of double-outlet right ventricle.Reference Ezon, Goldberg and Kyle 14 As we indicated in our discussion, there has never been any justification for this notion. When Fallot described his first three hearts obtained from patients with “la maladie bleue”, he described how, in one of them, the aorta arose exclusively from the right ventricle.Reference Fallot 3 More importantly, insistence on the presence of bilateral conuses to justify a diagnosis of double outlet transgresses the key principle known as the morphological method. This states that one variable feature within the heart should not be defined on the basis of another feature that is itself variable.Reference Van Praagh, David, Wright and Van Praagh 4 There are also more pragmatic reasons for discarding the concept of “bilateral conuses” as justification for the diagnosis of double-outlet right ventricle. When we recently assessed all the hearts with exclusive origin of both arterial trunks from the right ventricle in two large archives,Reference Ebadi, Spicer, Backer, Bleiweiss, Fricker and Anderson 15 in other words with so-called “200% double outlet”,Reference Lacour-Gayet, Haun and Ntalakoura 8 we found that two-thirds of the specimens lacked bilaterally complete subarterial infundibulums.
If we accept, therefore, that double-outlet right ventricle is no more than one form of ventriculo-arterial connection, the question arises as to how the group of hearts thus united can best be sub-categorised. There is general agreement that the location of the interventricular communication is most useful in this regard. In this regard, Lev et al,Reference Lev, Bharati, Meng, Liberthson, Paul and Idriss 7 in a ground-breaking investigation, had proposed that the defect could best be analysed as being subaortic, subpulmonary, doubly committed, or non-committed. As yet, however, to the best of our knowledge, there is no consensus as to how to determine commitment. This feature can vary, depending on whether it is judged on the basis of anatomical or surgical criteria.Reference Lacour-Gayet, Haun and Ntalakoura 8 , Reference Devaney, Lee and Gelehrter 9 The final decision regarding the capacity to tunnel the defect to one or other subarterial outlet will be made in the operating room, although it is becoming increasingly evident that use of three-dimensional reconstructions of clinical data sets can greatly facilitate this process. From the anatomical stance, our current observations, which combine developmental and morphological findings, provide a simple approach to commitment as opposed to non-commitment. During development, the embryonic interventricular communication leads directly into the proximal outflow tract when it is supported exclusively by the right ventricle. The right ventricular aspect of the septum is then reinforced by the septomarginal trabeculation, formed by compaction to right ventricular trabeculations. When the aortic root is transferred to the left ventricle, the muscularising proximal cushions insert onto the septum between the limbs of the newly formed septomarginal trabeculation or septal band. When the aortic root is not properly transferred, then the persisting interventricular communication opens to the right ventricle between these limbs of the septal band.Reference Mostefa-Kara, Bonnet, Belli, Fadel and Houyel 16 A committed, as opposed to a non-committed, interventricular communication, therefore, can be defined as one that opens to the right ventricle between the limbs of the septal band, or septomarginal trabeculation. Such committed defects in congenitally malformed hearts are typically found in the presence of separate atrioventricular junctions. Non-committed defects in this setting will be muscular defects opening to the inlet or the apical component of the right ventricle.
The problem then remains as to how best to categorise those defects in hearts with common atrioventricular junction that are confluent with the ventricular component of the co-existing atrioventricular septal defect. As was observed in several of our specimens, such atrioventricular septal defects typically extend to become directly subarterial (Figs 1b and 7). In such settings, it is entirely feasible that the surgeon will be able to create a tunnel connecting half of the common atrioventricular junction to one or other outflow tract so as to achieve biventricular repair.Reference Lacour-Gayet, Haun and Ntalakoura 8 , Reference Devaney, Lee and Gelehrter 9 From the anatomical stance, nonetheless, it is not unreasonable to consider the ventricular components of such atrioventricular septal defects as being non-committed. The defect, of necessity, will extend to the diaphragmatic surface of the ventricular mass, and hence be appreciably more extensive than the defects seen in the setting of separated atrioventricular junctions.
The location of the interventricular communication between the limbs of the septomarginal trabeculation, therefore, can provide a suitable definition of commitment as opposed to non-commitment. The question then remains as to how best to distinguish double from subaortic or subpulmonary commitment. In this regard, to date there has been a tacit acceptance that the defect is doubly committed either when the outlet septum is absent or when it is fibrous rather than muscular. Our current observations show that there is no justification for this assumption. The interventricular communication can be non-committed, as part of an atrioventricular septal defect, when the outlet septum is fibrous. More importantly, the attachment of a fibrous outlet septum can commit the defect into a subaortic or subpulmonary location just as readily as that of a muscular outlet septum. Our findings suggest, therefore, that it is the location of the septal attachment of the outlet septum that is the key to commitment. This feature retains its primacy when the outlet septum is fibrous rather than being muscular. The interventricular communication will be doubly committed when the outlet septum attaches to the midpoint of the inner heart curvature, irrespective of whether the inner curve, or ventriculo-infundibular fold, is itself myocardial or fibrous. Presuming the presence of spiralling arterial trunks, the committed defect will be confined in subaortic position when the outlet septum is attached to the cranial limb of the septomarginal trabeculation. If attached to the caudal limb of the trabeculation, then the defect with be committed to the pulmonary trunk, this arrangement being found most usually when the arterial trunks arise in parallel a manner from the ventricular mass, with the aorta in the right-sided or anterior position. This approach to the analysis of commitment does no more than endorse the concept put forward by Macartney et alReference Macartney, Rigby, Anderson, Stark and Silverman 17 on the basis of their echocardiographic investigations. Our current experience attests the accuracy of their initial observations.
Acknowledgement
None.
Financial Support
This research received no specific grant from any funding agency or from commercial or not-for-profit sectors.
Conflicts of Interest
None.