Section II - Hormones and Gestational Disorders
Published online by Cambridge University Press: 09 November 2022
Summary
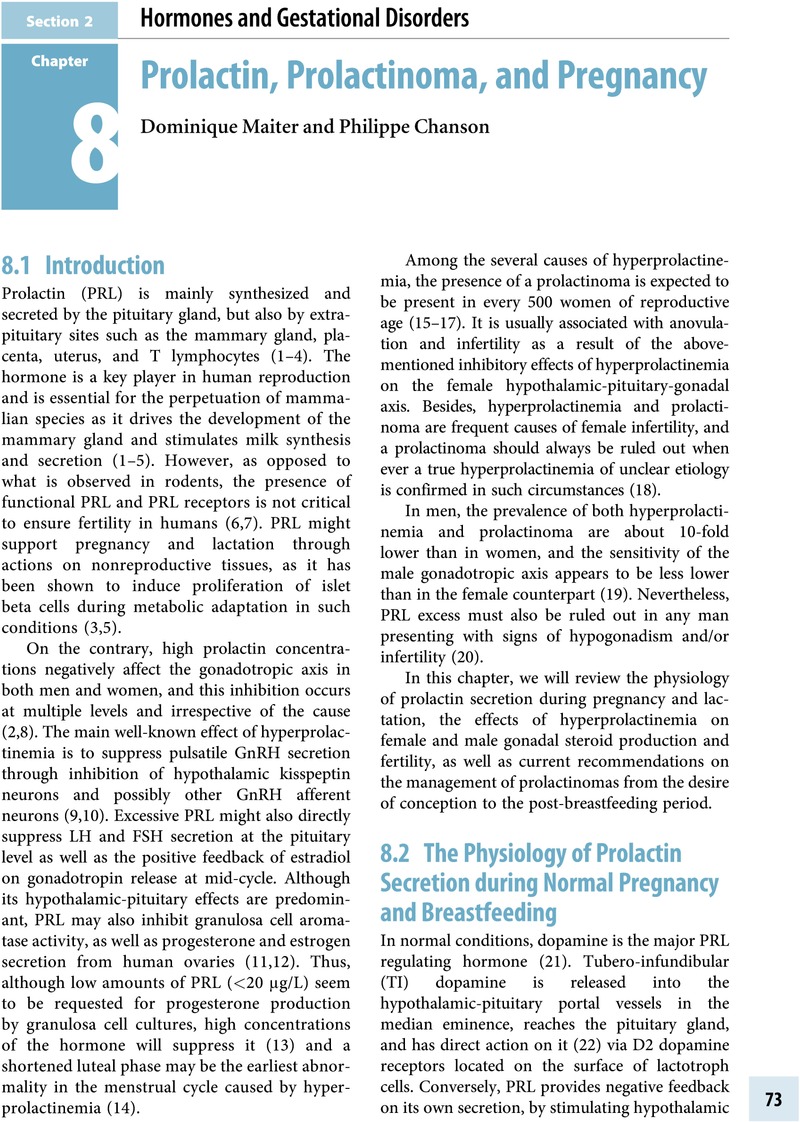
- Type
- Chapter
- Information
- Hormones and PregnancyBasic Science and Clinical Implications, pp. 73 - 198Publisher: Cambridge University PressPrint publication year: 2022