No CrossRef data available.
Article contents
Introduction
Published online by Cambridge University Press: 27 July 2018
Abstract
An abstract is not available for this content so a preview has been provided. Please use the Get access link above for information on how to access this content.
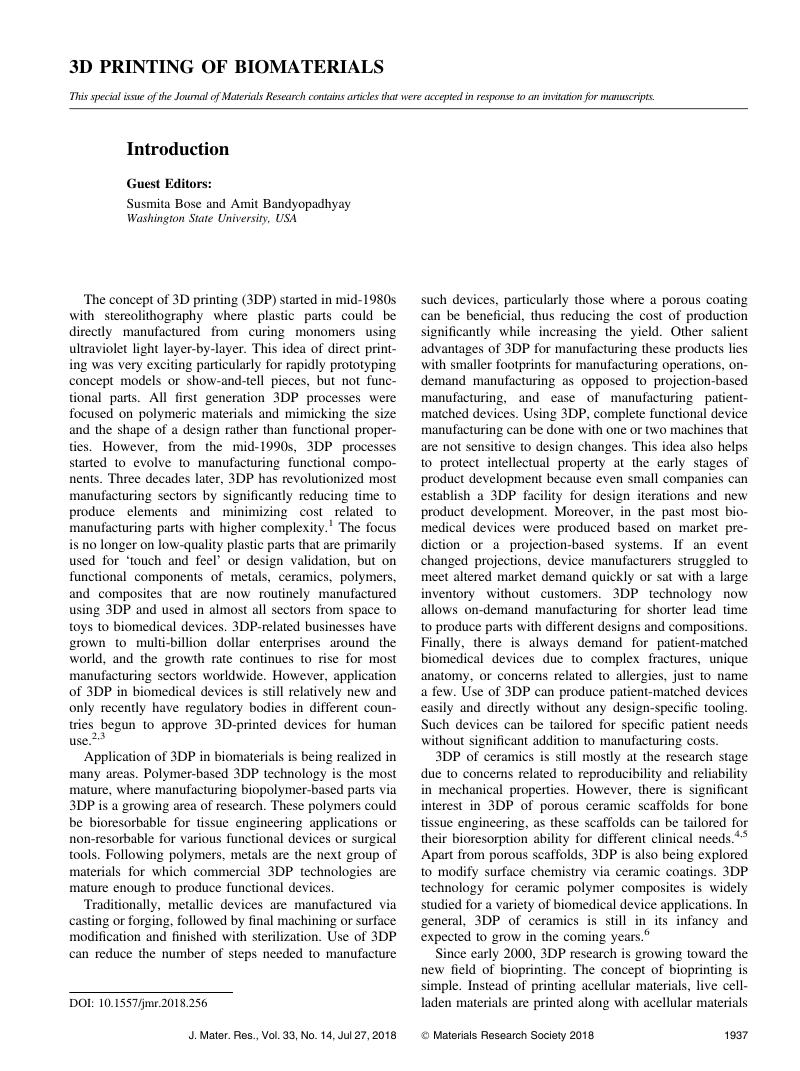
- Type
- 3D Printing of Biomaterials
- Information
- Journal of Materials Research , Volume 33 , Issue 14: Focus Issue: 3D Printing of Biomaterials , 27 July 2018 , pp. 1937 - 1938
- Copyright
- Copyright © Materials Research Society 2018
Footnotes
This special issue of the Journal of Materials Research contains articles that were accepted in response to an invitation for manuscripts.
References
REFERENCES
Additive Manufacturing, Bandyopadhyay, A. and Bose, S., eds. (CRC Press, 2015).CrossRefGoogle Scholar
Bose, S., Ke, D., Sahasrabudhe, H., and Bandyopadhyay, A.: Additive manufacturing of biomaterials. Prog. Mater. Sci. 93, 45–111 (2018).CrossRefGoogle Scholar
Bandyopadhyay, A., Bose, S., and Das, S.: 3D printing of biomaterials. MRS Bull. 40, 108–115 (2015).CrossRefGoogle Scholar
Bose, S., Robertson, S.F., and Bandyopadhyay, A.: 3D printing of bone implants and replacements. Am. Sci. 106, 112–119 (2018).CrossRefGoogle Scholar
Bose, S., Roy, M., and Bandyopadhyay, A.: Recent advances in bone tissue engineering scaffolds. Trends Biotechnol. 30, 546–554 (2012).CrossRefGoogle ScholarPubMed
Materials and Devices for Bone Disorders, Bose, S. and Bandyopadhyay, A., eds. (Elsevier, 2016).Google Scholar
Bose, S., Robertson, S.F., and Bandyopadhyay, A.: Surface modification of biomaterials and biomedical devices using additive manufacturing. Acta Biomater. 66, 6–22 (2018).CrossRefGoogle ScholarPubMed