Introduction
We start our account with a quote from a poem, The Conqueror Worm, which was first published in 1843 in Graham's Magazine. It is a poem by Edgar Allan Poe (fig. 1), about the inevitability of death. Did Poe know about the horrors of parasitic nematodes? Probably not, but Poe's parents were actors in the theatre where metaphors were used to describe life's events and tragedies. In his poem, The Conqueror Worm, the word Ȉworm’ is a metaphor for death, and is used in the same way as many ancients used this word. The ancients saw the actions of worms in the process of death and decay. The Conqueror Worm is interpreted to mean that human life is a mad folly ending in horrid death. The lines, above, from the poem describe the worm devouring, in a bloody manner, humans, who are mimes or puppets, while the do-gooders (seraphs) weep to no effect. The poem is also a strong metaphor for the effects of severe nematode parasitism on humans and the sometimes ineffective actions of concerned governments. In contrast to the tone of this poem, we choose to be optimistic, knowing that treatment with drugs can defeat the conqueror worm; but we need to know the weaknesses of the ambitious conqueror and to use and develop stronger weapons. This paper presents some recent insights into some of the mechanisms of actions of cholinergic anthelmintics and illustrates the potential use of microfluidic and expression techniques that facilitated recent advances in this field.

Fig. 1 Edgar Allan Poe, the author of the poem The Conqueror Worm, which was first published in 1843. Daguerreotype of Poe by William S. Hartshorn (1848), Library of Congress, Prints and Photographs Division [#91796062].
Nematode parasites are a major world problem
A group of diseases referred to as the neglected tropical diseases (NTDs) have an impact on significantly more people than HIV/AIDS, malaria and tuberculosis. The NTDs are mostly caused by parasitic nematodes. They may not kill, but they produce malnutrition that leads to debility, poor growth, reduced intellectual development, limb disfigurement and an increase in HIV/AIDS infection rates (Bentwich et al., Reference Bentwich, Teicher and Borkow2008). These diseases are associated with poverty, poor sanitation and poor education. The diseases perpetuate the poverty cycle because infected children cannot attend school, so they do not reach their full intellectual potential, and infected adults are less productive. Also important for human health is proper nutrition, which is adversely affected by the production loss caused by parasitic gastrointestinal nematodes in livestock.
We have used three parasitic nematodes that are models for NTDs and tractable to our molecular, pharmacological and electrophysiological methods. The nematodes are: (1) Ascaris suum (or lumbricoides, var. suum) which can produce ascariasis in humans and pigs; (2) Brugia malayi, which produces elephantiasis in humans; and (3) Oesophagostomum dentatum, which produces nodule formation and dysentery oesphagostomiasis in pigs and is a model for Oesophagostomum bifurcum which produces similar pathology in goats, pigs and humans in Togo and Ghana (Storey et al., Reference Storey, Anemana, van Oostayen and Magnussen2000).
Ascariasis is the most common human worm infection and is due to the large (20 cm) parasitic nematode, A. lumbricoides, which lives in the gastrointestinal tract. The worm is genomically very similar to, and may represent the same species as, A. suum of pigs (fig. 2A) (Liu et al., Reference Liu, Wu, Song, Wei, Xu, Lin, Zhao, Huang and Zhu2012). Ascariasis has a global prevalence of 36% (Chan et al., Reference Chan, Medley, Jamison and Bundy1994; Brooker et al., Reference Brooker, Pullan, Holland and Holland2013), producing symptoms of malnutrition, retardation of growth in children, diarrhoea, abdominal pain and death in a smaller proportion of cases (Bethony et al., Reference Bethony, Brooker, Albonico, Geiger, Loukas, Diemert and Hotez2006). Infection occurs in an estimated 1.4 billion people worldwide. Infection is spread by the faecal–oral route, and involves ingestion of embryonated eggs. Parasite infections, like ascariasis, which are spread by the faecal–oral route, are referred to as soil-transmitted nematodes (STNs). Worldwide, STNs are the major cause of morbidity in schoolchildren aged 5–14 years (Hotez, Reference Hotez2007).
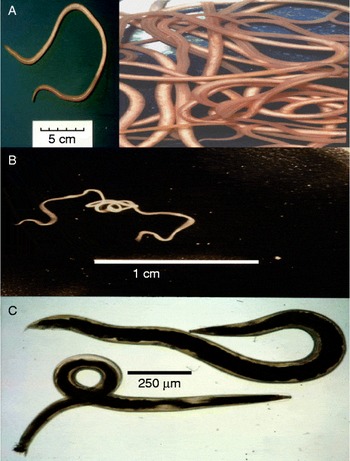
Fig. 2 Ascaris, Brugia and Oesophagostomum adult worms: (A) Ascaris suum, a single worm and scale bar (inset) and active swimming worms; (B) Brugia malayi, a single motile worm; (C) Oesophagostomum dentatum, female (top), male (bottom).
Lymphatic filariasis is another important NTD, produced by filarial nematodes like B. malayi (fig. 2B). These thread-like worms live in lymphatic vessels of humans for up to 6 years. Some 119 million individuals have been estimated to be afflicted with lymphatic filariasis (Michael et al., Reference Michael, Bundy and Grenfell1996), and many more (1 billion people worldwide) are at risk of contracting lymphatic filariasis and related filarial diseases. Transmission is via mosquitoes that bite and pick up microfilaria, which develop inside the mosquito into infective stages over 7–21 days. The larvae enter the mouthparts of the mosquito and then enter the punctured skin of the human following feeding. Although the infection may be symptomless, in about 10% of infected individuals blockage of the lymphatic vessels causes swelling known as elephantiasis. Elephantiasis is a gross swelling of the infected tissues that leaves individuals with severe disfigurement, an inability to work and sometimes exclusion from the social group.
Oesophagostomiasis infections in humans due to O. bifurcum are localized in northern Togo and Ghana (Storey et al., Reference Storey, Anemana, van Oostayen and Magnussen2000). The parasites produce nodules in the large colon and sometimes more serious dysentery. We are able to maintain a very similar parasite, Oesophagostomum dentatum, which causes infections of pigs (fig. 2C), by relatively simple passage techniques. We also have isolates of levamisole-sensitive and levamisole-resistant O. dentatum.
How can we control parasitic worms in general?
If we have a medical problem, then information on the cause of that problem allows us to limit, control and sometimes cure that problem. The more detailed and accurate our information is, the more effective the treatment is likely to be. Control of nematode parasitic disease has three focal points:
● preventative management, including sanitation and hygiene;
● vaccination;
● anthelmintic treatment.
Preventive management with effective sanitation and appropriate hygiene requires social cohesion and basic institutional and communal organization, which is available in developed economies but is not universally available. The essential components are clean water and effective sewage disposal; major advances in the control of the parasitic diseases would be possible if these were to be available and were maintained effectively. Vaccines against parasitic nematodes are very desirable but are not yet available. Trials with vaccines using two antigens – Necator americanus glutathione s-transferase 1 and N. americanus aspartic protease – are in phase 1 (Beaumier et al., Reference Beaumier, Gillespie, Hotez and Bottazzi2013). Vaccines against animal parasites, including lungworm (Dictyocaulus viviparous) and Haemonchus contortus (Albonico et al., Reference Albonico, Smith, Hall, Chwaya, Alawi and Savioli1994; Knox et al., Reference Knox, Redmond, Newlands, Skuce, Pettit and Smith2003; Smith & Zarlenga, Reference Smith and Zarlenga2006), have been produced, but of the few parasite vaccines that have been produced commercially, nearly all are based on attenuated organisms. The task is to identify stable antigens that induce strong immunity in the field, and many candidate antigens are from the excretory substance (ES) or the gut antigens of the nematode parasite. Despite the need for improved preventive management and vaccines, for many humans and animals, drugs remain the only option for treatment and prophylaxis.
Anthelmintics are used for treatment and prophylactic control
Over the past few years, governments of developing countries, WHO and public–private partnerships have collaborated to overcome the NTDs, but the fight has just started. In the absence of vaccines, and where sanitation and vector control is limited, control of these infections relies on regular administration of anthelmintic drugs.
For control of STN infections such as ascariasis, anthelmintic chemotherapy has focused on a small number of drugs that belong to three major groups (Martin, Reference Martin1997): (1) benzimidazoles, like albendazole, bind to nematode β-tubulin and act over several days to inhibit metabolism and egg production; (2) nematode-selective nicotinic compounds (levamisole and pyrantel) act more rapidly and produce spastic paralysis; and (3) avermectins, such as ivermectin and abamectin, inhibit motility and pharyngeal pumping.
In endemic communities, the goal for control of filariasis has been to eliminate microfilaria from blood to interrupt transmission. Single-dose diethylcarbamazine with albendazole, or albendazole with ivermectin (Ottesen, Reference Ottesen2000) is advocated in the Global Program to Eliminate Lymphatic Filariasis. These drugs do not kill adults and have not changed over 20 years. There is a concern about resistance and a need for new antifilarial drugs (Kumar et al., Reference Kumar, Chaudhary, Foster, Novelli, Zhang, Wang, Spiro, Ghedin and Carlow2007). Although levamisole has been used for treatment (Mak & Zaman, Reference Mak and Zaman1980), it requires multiple doses. Development of novel cholinergic anthelmintics (derquantel, monepantel and the combination derquantel+avermectin) for other nematode parasites makes evaluation and characterization of Brugia nicotinic acetylcholine receptor channels (nAChRs) as target sites timely and imperative. Recent publication of the draft genome sequence of B. malayi has allowed identification of the nAChR subunit genes (Williamson et al., Reference Williamson, Walsh and Wolstenholme2007), and encourages the characterization of native receptors.
Anthelmintic resistance that follows mass drug administration (MDA) creates an urgent need for basic research and identification of new drugs. For MDA, it is easier to administer the therapeutic agent as a single dose without adjusting for weight. Albendazole is easier to administer for this reason and is used more widely. Fewer countries use levamisole and pyrantel on a wide scale because of the need to adjust for weight of individuals. The increased albendazole/mebendazole use appears to be leading to development of resistance in STNs of humans (Albonico et al., Reference Albonico, Wright, Ramsan, Haji, Taylor, Savioli and Bickle2005) as it did in animals (Jackson, Reference Jackson1993; Kaplan, Reference Kaplan2004). To address this problem of benzimidazole resistance, additional drugs are required, with a different site of action and suitable for prophylactic mass treatment with easy administration. Tribendimidine has been suggested as one drug for STN single-dose MDA. In filaria, there is also evidence of the emergence of resistance because of the regular use of ivermectin (Osei-Atweneboana et al., Reference Osei-Atweneboana, Eng, Boakye, Gyapong and Prichard2007). It is widely recognized that existing single-dose MDAs are limited and there is an urgent need to support research that allows development of novel anthelmintics or combinations that increase spectrum and limit resistance (Leathwick, Reference Leathwick2013).
Cross-resistance
Development of resistance to anthelmintics has followed continuous use for prophylaxis in animals. The resistance to one drug in a particular drug class has been associated with resistance to other drugs in that same class (Sangster & Gill, Reference Sangster and Gill1999). This is referred to as cross-resistance and is seen for drugs in the benzimidazole (albendazole could show cross-resistance with fenbendazole) and avermectin (ivermectin could show cross-resistance with doramectin) classes.
Nematode nicotinic receptors and anthelmintics
Major classes of anthelmintic act on ligand-gated ion-channels (LGICs), demonstrating that these channels are validated drug target sites, which should be characterized more fully to facilitate novel drug development and use. The nematode parasite neuromuscular system has provided a good drug target system for anthelmintics. We can now monitor the effects of drugs that target the neuromuscular system by measuring precise changes in the motility of the parasites. New microfluidic techniques (fig. 3) allow drug effects on the neuromuscular system to be dissected and quantitated through recording and analysing the sinusoidal movement of L3 larvae, using measurements of the amplitude (a), frequency (N), wavelength (λ) and velocity (v) to observe effects of the anthelmintics on these parameters (Chen et al., Reference Chen, Deutmeyer, Carr, Robertson, Martin and Pandey2011). It has also been found that the nematode L3 larvae are sensitive to applied electrical fields and can be corralled into a drug well or driven out of the drug well at fixed times to measure anthelmintic drug effects on the neuromuscular system (Carr et al., Reference Carr, Parashar, Gibson, Robertson, Martin and Pandey2011). It is likely that the microfluidic technology will allow better characterization of different phenotypes or isolates of the same species of nematode parasite: anthelmintic-sensitive and anthelmintic-resistant isolates differ in their motility (Carr et al., Reference Carr, Parashar, Gibson, Robertson, Martin and Pandey2011; Chen et al., Reference Chen, Deutmeyer, Carr, Robertson, Martin and Pandey2011).

Fig. 3 Microfluidic chamber with drug well and micro-channels. The L3 larvae of nematode parasites or adult Caenorhabditis elegans are placed in the input–output port (worm inlet 1/0 port) via syringe and catheter. They can be driven into the drug well and held under a voltage field, and released at a defined time by reversing the voltage. The escape and behaviour of the larvae/worms following entry into the drug field is tested at different drug concentrations. The swimming of the larvae/worms in the channels is described by a sinusoidal wave with measurement of frequency (N), wavelength (λ), amplitude (a) and velocity (v).
Pyrantel and levamisole are selective agonists on nematode nAChRs. Ivermectin and moxidectin are allosteric modulators of channels that include the glutamate-gated chloride channels and nAChRs. We can see that LGICs are validated target sites by being sites of action of existing anthelmintics. LGICs are also good target sites for novel drugs, provided the new drugs are not made redundant by cross-resistance. The focus of this article is on cholinergic drugs that activate selectively the nAChRs.
Application of modern molecular methods based on small ribosomal DNA subunit sequences (Blaxter et al., Reference Blaxter, De Ley, Garey, Liu, Scheldeman, Vierstraete, Vanfleteren, Mackey, Dorris, Frisse, Vida and Thomas1998; Holterman et al., Reference Holterman, van der Wurff, van den Elsen, van Megen, Bongers, Holovachov, Bakker and Helder2006) divide the phylum Nematoda into Clades I, II, III, IV and V. Caenorhabditis elegans is in Clade V and has been a helpful genetic model for Clade V (Rhabditidae) parasitic nematodes, such as O. dentatum. Its genome has been sequenced and the nAChR subunit genes have been identified. However, accumulating evidence suggests that C. elegansis limited as a model for parasitic nematodes. Caenorhabditis elegans appears to have the largest number of nAChR subunit genes of all nematodes in contrast to those of Clade III, which are phylogenetically distant, and which have far fewer nAChR subunit genes (Williamson et al., Reference Williamson, Walsh and Wolstenholme2007). In C. elegans, the subunit genes unc-38, unc-29, unc-63, lev-1and lev-8are required to encode for the levamisole nAChR but lev-1 appears to be missing in Clade III parasites such as B. malayi and A. suum.
Knowledge has advanced of genomes of B. malayi (Ghedin et al., Reference Ghedin, Wang, Spiro, Caler, Zhao, Crabtree, Allen, Delcher, Guiliano, Miranda-Saavedra, Angiuoli, Creasy, Amedeo, Haas, El-Sayed, Wortman, Feldblyum, Tallon, Schatz, Shumway, Koo, Salzberg, Schobel, Pertea, Pop, White, Barton, Carlow, Crawford, Daub, Dimmic, Estes, Foster, Ganatra, Gregory, Johnson, Jin, Komuniecki, Korf, Kumar, Laney, Li, Li, Lindblom, Lustigman, Ma, Maina, Martin, McCarter, McReynolds, Mitreva, Nutman, Parkinson, Peregrin-Alvarez, Poole, Ren, Saunders, Sluder, Smith, Stanke, Unnasch, Ware, Wei, Weil, Williams, Zhang, Williams, Fraser-Liggett, Slatko, Blaxter and Scott2007), A. suum (Jex et al., Reference Jex, Liu, Li, Young, Hall, Li, Yang, Zeng, Xu, Xiong, Chen, Wu, Zhang, Fang, Kang, Anderson, Harris, Campbell, Vlaminck, Wang, Cantacessi, Schwarz, Ranganathan, Geldhof, Nejsum, Sternberg, Yang, Wang and Gasser2011) and transcriptomes of O. dentatum (Romine et al., Reference Romine, Martin and Beetham2013). With new sequencing technology, knowledge of the genomes of representative parasitic nematodes has advanced rapidly with B. malayi and A. suum. However, compared to C. elegans, there is a bottleneck; there are a limited number of functional studies from parasitic nematodes characterizing ion-channels of neuromuscular transmission. Additional functional studies of ion-channels in native preparations of parasitic nematodes or expressed ion-channels are required. There are only a limited number of in vivo reports of ion-channels from filaria. The Saz group described contraction and electrophysiological studies from the filarid Dipetalonema vitiae body muscle and reported responses to acetylcholine (Rohrer et al., Reference Rohrer, Esch and Saz1988; Christ et al., Reference Christ, Goebel and Saz1990, Reference Christ, Oh and Saz1994). Cloned and expressed glutamate-gated chloride channels from Dirofilaria immitis have demonstrated ivermectin sensitivity (Yates & Wolstenholme, Reference Yates and Wolstenholme2004). For A. suum, H. contortus and O. dentatum, the situation is better; there are observations from A. suum body muscle (Martin, Reference Martin1982, Reference Martin1985; Robertson & Martin, Reference Robertson and Martin1993; Evans & Martin, Reference Evans and Martin1996; Qian et al., Reference Qian, Martin and Robertson2006) and A. suum expressed channels (Williamson et al., Reference Williamson, Robertson, Brown, Williams, Woods, Martin, Sattelle and Wolstenholme2009; Bennett et al., Reference Bennett, Williamson, Walsh, Woods and Wolstenholme2012); H. contortus expressed receptors (Boulin et al., Reference Boulin, Fauvin, Charvet, Cabaret, Bessereau and Neveu2011) and O. dentatum body muscle (Robertson et al., Reference Robertson, Bjorn and Martin1999; Qian et al., Reference Qian, Martin and Robertson2006) and O. dentatum expressed channels (Buxton et al., Reference Buxton, Charvet, Neveu, Cabaret, Cortet, Peineau, Abongwa, Courtot, Robertson and Martin2014). One of the interesting and significant findings is that the subunit combination of the nAChR receptor affects the sensitivity to the nicotinic anthelmintic drug (Williamson et al., Reference Williamson, Robertson, Brown, Williams, Woods, Martin, Sattelle and Wolstenholme2009; Buxton et al., Reference Buxton, Charvet, Neveu, Cabaret, Cortet, Peineau, Abongwa, Courtot, Robertson and Martin2014). Figure 4 illustrates expression of two (of the four) nAChR subtypes that have been expressed in Xenopus oocytes using combinations of muscle subunit cRNAs of Ode-unc-63, Ode-unc-38, Ode-unc-29 or Ode-acr-8 (Buxton et al., Reference Buxton, Charvet, Neveu, Cabaret, Cortet, Peineau, Abongwa, Courtot, Robertson and Martin2014). The Ode-UNC-63:Ode-UNC-29:Ode-UNC-38:Ode-ACR-8 subtype is most sensitive to levamisole and is competitively antagonized by derquantel, but Ode-UNC-63:Ode-UNC-29:Ode-UNC-38 is more sensitive to pyrantel and is non-competitively antagonized by derquantel. Interestingly, the Ode-UNC-63:Ode-UNC-29:Ode-UNC-38:Ode-ACR-8, has a 35 pS single channel conductance like the in vivo L-subtype receptor that is lost with levamisole resistance (Robertson et al., Reference Robertson, Bjorn and Martin1999), suggesting that it corresponds to the native receptor. The different subtypes of nAChR present in vivo (Robertson & Martin, Reference Robertson and Martin1993; Qian et al., Reference Qian, Martin and Robertson2006) and the expression experiments (Williamson et al., Reference Williamson, Robertson, Brown, Williams, Woods, Martin, Sattelle and Wolstenholme2009; Buxton et al., Reference Buxton, Charvet, Neveu, Cabaret, Cortet, Peineau, Abongwa, Courtot, Robertson and Martin2014) suggest that the cholinergic anthelmintic receptors may be more variable (and plastic) than we had anticipated. We do not know yet if, or how, this variability may play a role in resistance to the cholinergic anthelmintics.

Fig. 4 Expression of different parasite nAChRs using Xenopus oocyte expression reveals the effect of subunit combinations on anthelmintic sensitivity. Antagonism of derquantel depends on nAChR subtype, demonstrated using two of the four different expressed O. dentatum subunits that can occur. (A) Expression of Ode-UNC-63:Ode-UNC-29:Ode-UNC-38:Ode-ACR-8 in Xenopus oocytes produces receptors that, under voltage clamp, produce currents to acetylcholine, levamisole, tribendimidine, pyrantel, bephenium, thenium and nicotine, with the biggest current to levamisole. This receptor is competitively antagonized by derquantel (plot). (B) Expression of Ode-UNC-63:Ode-UNC-29:Ode-UNC-38 in Xenopus oocytes produces receptors that, under voltage clamp, produce currents to acetylcholine, levamisole, tribendimidine, pyrantel, bephenium, thenium and nicotine, with the biggest current to pyrantel. This receptor is non-competitively antagonized by derquantel (plot).
Nicotinic anthelmintics such as pyrantel and levamisole selectively paralyse nematodes by activating cholinergic ion-channels (nAChRs) in their body muscle to produce contraction and spastic paralysis. Figure 5 illustrates the effect of cumulative application of levamisole to a muscle strip of A. suum. The importance of nematode nAChRs as drug targets has increased because of the recent introduction of novel cholinergic compounds. The new cholinergic anthelmintics, morantel tribendimidine and derquantel (Kaminsky et al., Reference Kaminsky, Ducray, Jung, Clover, Rufener, Bouvier, Weber, Wenger, Wieland-Berghausen, Goebel, Gauvry, Pautrat, Skripsky, Froelich, Komoin-Oka, Westlund, Sluder and Maser2008; Rufener et al., Reference Rufener, Maser, Roditi and Kaminsky2009; Buxton et al., Reference Buxton, Charvet, Neveu, Cabaret, Cortet, Peineau, Abongwa, Courtot, Robertson and Martin2014) have different nAChR subtype selectivities. Figure 4, for example, shows that tribendimidine is more potent than levamisole on the Xenopus oocyte expressed Ode(29-63-38) subtype, and that levamisole is more potent than tribendimidine on the Ode(29-63-8-38) subtype. The presence in vivo of different cholinergic receptor subtypes activated by anthelmintics has been demonstrated in experiments using the A. suum muscle contraction strip (fig. 5) and the antagonists derquantel, paraherquamide and methyllycaconitine (MLA). Paraherquamide is a more potent antagonist of nicotine than bephenium, and nicotine and bephenium are selective for different receptor subtypes. The cholinergic anthelmintic subtypes are separated into at least three in vivo subtypes: N-, L- and B-subtypes ((Robertson et al., Reference Robertson, Clark, Burns, Thompson, Geary, Trailovic and Martin2002; Qian et al., Reference Qian, Martin and Robertson2006). The three different subtypes present in A. suum are: the N-subtype, which is nicotine selective; the L-subtype, which is levamisole selective; and the B-subtype, which is bephenium selective; suggesting that resistance to the anthelmintic which stimulates the L-subtype may not be associated with resistance to anthelmintics which stimulate the N-subtype or the B-subtype.

Fig. 5 Photograph of an Ascaris body muscle flap being tested with levamisole under isometric contraction. The trace shows the contraction effect of levamisole applied in cumulative doses.
Few drugs with optimal properties exist and only albendazole is usually used for single-dose MDA. Tribendimidine has been developed by the Chinese Centers for Disease Control and Prevention and has potential for single-dose MDA, but details of its action in parasites are not clear. It is a promising anthelmintic with a symmetrical diamidine structure. The China State Food and Drug Administration approved it for human use in 2004 (Steinmann et al., Reference Steinmann, Zhou, Du, Jiang, Xiao, Wu, Zhou and Utzinger2008). It has broad-spectrum action in single-dose therapy against parasitic nematodes of humans, with effects against Ascaris, hookworm and Strongyloides (Xiao et al., Reference Xiao, Hui-Ming, Tanner, Utzinger and Chong2005). There is, however, some concern about the rapid metabolism of tribendimidine and production of potential carcinogens.
Little was known of the mode of action of tribendimidine until Hu et al. (Reference Hu, Xiao and Aroian2009) published observations on C. elegans. These authors used molecular experiments to suggest that the site of action of tribendimidine was the levamisole receptor (nAChR). These experiments are helpful but the observations are limited because the levamisole receptor of C. elegans has different pharmacological properties to the muscle receptors of parasitic nematodes. The Clade V C. elegans levamisole-sensitive receptor has different subunits and is not activated by nicotine. Observations on O. dentatum nAChR expressed in Xenopus oocytes show that tribendimidine has a different subtype selectivity to levamisole (Buxton et al., Reference Buxton, Charvet, Neveu, Cabaret, Cortet, Peineau, Abongwa, Courtot, Robertson and Martin2014) and may not, therefore, show cross-resistance with levamisole.
Derquantel and abamectin
Paraherquamide-A and macfortine-A are fermentation products of a Penicillium species that were discovered by Merck. Subsequently, Pfizer used nematode parasites that were resistant to levamisole, benzimidazoles and macrocyclic lactones, and maintained in jirds, to look for compounds that were ‘resistance busting’. The medicinal chemists modified the structure of paraherquamide by reducing the C-ring ketone of paraherquamide-A, to produce a less host-toxic 2-desoxyparaherquamide derivative which was given the generic name, derquantel. Derquantel is equally effective when given orally, intravenously, intraperitoneally or intramuscularly (Johnson et al., Reference Johnson, Coscarelli, Davis, Zaya, Day, Barsuhn, Martin, Vidmar, Lee, Conder, Geary, Ho and Thompson2004) in jirds against gastrointestinal levamisole-resistant parasites. It has been introduced as an animal anthelmintic with an avermectin, abamectin, because clinically they have additive/synergistic effects (Little et al., Reference Little, Hodge, Watson, Seed and Maeder2010).
We identified the mode of action of derquantel in muscle strip preparations of A. suum and found that derquantel was a competitive, but selective, cholinergic antagonist (Robertson et al., Reference Robertson, Clark, Burns, Thompson, Geary, Trailovic and Martin2002). Parasitic gastrointestinal nematodes resistant to levamisole retain sensitivity to the B-subtype agonist, bephenium (Sangster et al., Reference Sangster, Davis and Collins1991) and derquantel has B-subtype selectivity (Qian et al., Reference Qian, Martin and Robertson2006). These observations may explain why derquantel does not show cross-resistance to levamisole and why it has ‘resistance busting’ properties.
A significant observation (Puttachary et al., Reference Puttachary, Trailovic, Robertson, Thompson, Woods and Martin2013) that we have made using A. suum muscle in our muscle contraction assay and, separately, in current-clamp experiments, is that derquantel interacts additively with the macrocyclic lactone, abamectin (fig. 6). At higher acetylcholine concentrations the interaction is synergistic. This is a novel and important observation and suggests that the combination approach of derquantel + abamectin can enhance the therapeutic effects of the anthelmintics.

Fig. 6 (A) Isometric contraction of Ascaris suummuscle strips produced by application of increasing concentrations of acetylcholine and antagonism by 1 μm derquantel, 1 μm derquantel + 0.3 μm abamectin and wash. Note that derquantel decreases the responses to acetylcholine and that the addition of abamectin increases the inhibition. (B) The concentration-depolarizing–response plot of acetylcholine, showing mean ± standard error (SE) bars. Control (n= 11, filled circles); in the presence of 1 μm derquantel (n= 11); 1 μm derquantel +0.3 μm abamectin. The predicted additive effect is shown by the dashed line. The derquantel–abamectin combination is statistically (P< 0.05) more inhibitory than additive at concentrations>10 μm acetylcholine.
Brugia malayi preparations
We are particularly excited by developments of the B. malayi muscle-flap preparation for recording from single muscle cells (Robertson et al., Reference Robertson, Puttachary and Martin2011, Reference Robertson, Buxton and Martin2013). We glue one side of a 0.5 cm body cylinder section with GluShield (®Glushield, Seattle, Washington, USA) delivered with a modified patch pipette under the high power of the dissecting microscope. The body cylinder is then cut open and, after removal of the intestine and uterus, it is glued flat. We use Nomarski optics (fig. 7) to view the muscle cells. We make patch recordings following brief collagenase treatment to clear the extracellular matrix. Single-channel recordings using cell-attached and isolated inside-out patch recordings or whole-cell recordings allow us to study nAChRs in the intact preparation. Figure 7B shows our recently developed recording system for whole-cell currents made from a B. malayi muscle (Robertson et al., Reference Robertson, Puttachary and Martin2011, Reference Robertson, Buxton and Martin2013). It shows the sequential application of 30 μm acetylcholine, tribendimidine, pyrantel, levamisole and bephenium along with a bar chart of normalized currents. Interestingly bephenium is the least potent of the agonist series, which is different from the situation in A. suum muscle, suggesting that the receptor subtypes present are different from the receptors of B. malayi. Figure 7C shows the effect of longer-term application of 30 μm levamisole on adult motility, and that after a period of some 10 min the inhibition of motility begins to be lost.

Fig. 7 Nomarski photomicrograph of a Brugia malayi muscle flap dissection with a diagram of a patch-pipette placed for whole-cell current clamp recording of the nicotinic receptors. Scale bar 10 μm. (B) Bar chart (mean ± SE) of normalized currents produced by the nAChR agonists/anthelmintics (30 μm) on B. malayi muscles in whole-cell patch-clamp. All current responses were normalized to ACh currents (*P <0.05, paired t-test). Displayed above the bars are sample whole-cell current traces. Scale bar, horizontal 30 s, vertical 700 pA. (C) Plot of motility versus time (min) of adult female B. malayiin the absence and presence of 30 μm levamisole. Four worms/treatment were used for the assay. Comparisons of motility were made between control and treated worms at each time point, **P< 0.01, ***P< 0.001.
Concluding comments
The neglected tropical diseases, produced by phylogenetically separate Clades of nematode parasites, produce widespread and debilitating diseases in humans (de Silva et al., Reference de Silva, Brooker, Hotez, Montresor, Engels and Savioli2003; Bethony et al., Reference Bethony, Brooker, Albonico, Geiger, Loukas, Diemert and Hotez2006; Hotez et al., Reference Hotez, Brindley, Bethony, King, Pearce and Jacobson2008). Anthelmintic drugs are used for treatment and prophylaxis but there are concerns about the development of resistance in humans (Diawara et al., Reference Diawara, Drake, Suswillo, Kihara, Bundy, Scott, Halpenny, Stothard and Prichard2009, Reference Diawara, Halpenny, Churcher, Mwandawiro, Kihara, Kaplan, Streit, Idaghdour, Scott, Basanez and Prichard2013) as in animals (Kaplan, Reference Kaplan2004). There is a need for effective drugs against soil-transmitted nematodes (Hu et al., Reference Hu, Ellis, Yiu, Miller, Urban, Shi and Aroian2013) and filaria (Prichard, Reference Prichard2005). We think that the introduction of the new microfluidic technology and expression systems and research on the ligand-gated ion-channels is timely, specifically the nicotinic acetylcholine receptors, and therapeutically relevant. There is a need to use molecular and functional assays to study similarities, pharmacological diversity and modulation of the nAChR subtypes in the different Clades. The subtypes appear as separate target sites for the classic anthelmintics, monepantel and derquantel. Knowledge of subtype selectivities and drug interactions (e.g. derquantel and avermectins) allows combinations of anthelmintics to be produced to cover broader spectra of receptors and parasites. These are anticipated to be more effective therapeutically in the face of resistance. The need for other novel ‘resistance busting’ anthelmintics from other classes of anthelmintic exists for human as well as animal nematode parasite control. There is still an urgent need for vaccines, antivectoral agents, improved sanitation, better diagnostic methods and markers for resistance, while combinations of anthelmintics will also need to be studied at the basic and clinical levels. There are many interesting and exciting basic science and translational issues that need to be addressed. We look forward to seeing the field develop over the coming years.
Acknowledgments
The authors would like to thank the Filariasis Research Reagent Resource Centre (FR3; College of Veterinary Medicine, University of Georgia, USA) for the supply of live adult worms.
Financial support
Funding for this study was from an Iowa Center for Advanced Neurotoxicology (ICAN) grant to A.P.R. and National Institute of Allergy and Infectious Diseases (NIH) grant R 01 A1 047194 to R.J.M.; NIH grant R21 AI092185-02 to A.P.R. and a grant from Zoetis to R.J.M and A.P.R.
Conflict of interest
Part of the work reported by Puttachary et al. (2013) was supported by Zoetis. The authors declare no other conflict of interest.